Essentials of Inorganic Chemistry PDF
Document Details
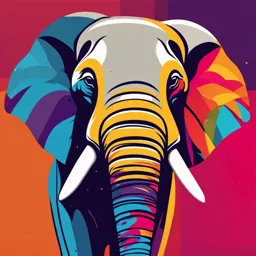
Uploaded by DiligentJasper2006
2015
Katja A. Strohfeldt
Tags
Summary
This is a textbook on inorganic chemistry, focusing on topics relevant to pharmacy, pharmaceutical sciences, and medicinal chemistry. It covers the structure and properties of various inorganic compounds and their applications in different fields.
Full Transcript
192 Essentials of Inorganic Chemistry OH OH Fe O...
192 Essentials of Inorganic Chemistry OH OH Fe O O n N N (a) (b) Figure 8.15 Chemical structure of 4-hydroxytamoxifen (a) and ferrocifen (b) which has been used as a basis for a new DACH–Pt–tamoxifen derivative. Oxaliplatin showed a cytotoxic effect of 6.3 μM when tested on the oestrogen-dependent human breast cancer cell line MCF-7, whilst the tamoxifen-vectorised derivatives (see Figure 8.16; R = H, 14 μM and R = OH, 4 μM) also presented an antiproliferative effect at a similar magnitude. Looking in more detail, research shows that the derivative that contains the hydroxyl group displays a higher RBA and also a better IC50 value. This shows that the hydroxyl group (also present in the active metabolite of tamoxifen) is important for the recognition by the oestrogen receptor. Nevertheless, the vectorisation of DACH–Pt does not really result in a significant improvement in comparison to oxaliplatin itself, and therefore this combination is not really beneficial as an SERM for the fight against breast cancer. The titanocene-tamoxifen derivative has an RBA value of 8.5%, which means it should recognise the oestro- gen receptor well. The results of the cytotoxicity tests were very unexpected, where a proliferative effect was observed. The estrogenic effect was comparable to that of oestrogen itself. It is believed that this estrogenic effect is due to the titanium moiety and/or its hydrolysis products (Figure 8.17). In order to bring ferrocifen into clinical studies, it was important to find a suitable formulation. This is an area notoriously difficult for metal-based drugs, and OH-ferrocifen finally entered phase II clinical trials. A variety of pharmaceutical approaches have been researched, including the use of nanoparticles, cyclodextrins and lipid nanocapsules. Organometallic Chemistry 193 R H3CH2C O O(CH2)3NMe2 O O O Pt H2N NH2 Figure 8.16 Chemical structure of the DACH–Pt–tamoxifen derivative (R = H, OH) CI O(CH2)2NMe2 M CI Figure 8.17 Chemical structure of titanocifen 194 Essentials of Inorganic Chemistry 8.4 Titanocenes Titanium, with chemical symbol Ti and atomic number 22, is a member of the d-block metals and belongs to group 4 of the periodic table of elements (Figure 8.18). Titanium is a metal, which puzzles the bioinorganic scientists, as it has no known native role in the human body (or any organism) despite it is the ninth most abundant element in the earth’s crust. The metal titanium is extremely corrosion-resistant and has a remarkable biocompatibility, which has led to its clinical applications. These include endoprostheses of knees and hips, dental implants, heart pacemakers and many more. Titanium complexes are used for their catalytic properties, and there are complexes that interact with biomolecules (this will be shown below). Nevertheless, there is no known function of titanium as a native essential metal [6, 7]. The most stable oxidation state is Ti(IV), and most titanium compounds with lower oxidation states are easily oxidised to Ti(IV). It is very characteristic that these Ti(IV) species easily undergo hydrolysis and form Ti—O bonds and fairly often polymeric structures. Titanium oxides and many Ti(IV) compounds do not show high solubility in water and therefore have only limited bioavailability. This might explain why titanium has no known biological role despite its high natural abundance, and is not an essential metal for any life form. Titanium dioxide (TiO2 ) is a white solid with a low aqueous solubility and is the natural occurring oxide of titanium present in minerals such as rutile (composed mainly of TiO2 ). TiO2 is used as a white pigment in paints, food colouring, sunscreens and toothpaste. For the purification of crude TiO2 , the raw material is reduced with carbon and then oxidised in the presence of chlorine. The resulting titanium tetrachloride (TiCl4 ) is purified by distillation and reacted with oxygen at around 2000 K to form TiO2 in its pure form. Current research into the medicinal applications of titanium complexes mainly focusses on their use as anticancer agents. It is important to note that these complexes are different from the above-described titanium alloys/metals or TiO2 , which are highly insoluble in aqueous media. The titanium complexes described below contain functional groups that can be easily exchanged and/or interact with biomolecules in order to fulfil their medicinal role. H He Li Be B C N O F Ne Na Mg Al Si P S Cl Ar K Ca Sc Ti V Cr Mn Fe Co Ni Cu Zn Ga Ge As Se Br Kr Rb Sr Y Zr Nb Mo Tc Ru Rh Pd Ag Cd In Sn Sb Te I Xe La- Cs Ba Hf Ta W Re Os Ir Pt Au Hg Tl Pb Bi Po At Rn Lu Ac- Fr Ra Rf Db Sg Bh Hs Mt Ds Rg Uub Lr Figure 8.18 Periodic table of elements; the element titanium is highlighted Organometallic Chemistry 195 H3C O CI C2H5O O Ti Ti CI C2H5O O O CH3 [(η5-Cp)2TiCI2] titanocene dichloride (a) cis,cis,cis-Δ-[Ti(bzac)2(OEt)2] budotitane (b) Figure 8.19 Chemical structures of Cp2 TiCl2 (a) and budotitane (b) 8.4.1 History of titanium-based anticancer agents: titanocene dichloride and budotitane The success of cisplatin stimulated the search for further metal-based anticancer drugs. Two titanium-based complexes, namely titanocene dichloride (Cp2 TiCl2 ) and budotitane (Ti(IV)(bzac)2 (OEt)2 , bzac, 1- phenylbutane-1,3-dionate), were identified as promising drug candidates and entered clinical trials. Both compounds contain labile groups in the cis position analogous to cisplatin. Unfortunately, hydrolysis in titanium compounds is faster than in cisplatin, and in these cases can lead to the formation of hydroxo-bridge species and potentially to TiO2 , which is insoluble in water. This represents the main challenge for the clinical application of this class of compounds (Figure 8.19). Cp2 TiCl2 was first synthesised in 1954 and is a red crystalline solid in its pure form. The Ti(IV) centre is coordinated by two 𝜂 5 -Cp ligands and two chlorine ligands. Cp2 TiCl2 can be synthesised by reacting titanium tetrachloride (TiCl4 ) with NaCp. Compared to those of ferrocene, the planar cyclopentadienyl ligands are coordinated to the titanium centre in a bent sandwich configuration. Furthermore, Cp2 TiCl2 is an exception to the 18 VEs rule, as it has only 16 VEs, but it is still a stable complex. Each Cp− ligand contributes six elec- trons, whilst each Cl− ligand contributes two electrons. Ti(IV) has no VEs left, which makes a total of 16 VEs. 2NaC5 H5 + TiCl4 → (C5 H5 )2 TiCl2 + 2NaCl⟩ Compared to cisplatin, much less is known on the mode of action of titanium-based drug candidates. In fact, the active species responsible for the anticancer activity of Cp2 TiCl2 in vivo has yet not been identified, nor has the coordination mechanism to DNA or eventual repair processes. Studies have shown that titanium will accumulate near phosphorus-rich areas, indicating a titanium–DNA interaction takes place. Crystallo- graphic studies suggest that, within Cp2 TiCl2 , the Cp groups sterically prevent the cross-linking of DNA. A direct comparison between Cp2 TiCl2 and cisplatin shows that their spectrum of activity differs, leading to the conclusion that their mode of action must also differ. Current studies have confirmed the uptake of Ti(IV) by transferrin and transport to the cancer cells. Within the cell, it is believed that Ti(IV) may bind, in contrast to cisplatin, to the DNA backbone, which means coordinating to the negatively charged phosphates. Generally, it is believed that this coordination is not very strong. 196 Essentials of Inorganic Chemistry Cp2 TiCl2 showed very promising results in preclinical studies. In vitro studies have been carried out by Köpf-Maier on a variety of human cancers including human lung and renal cell cancer carcinomas xenografted into mice. The results were very encouraging, as a significant response of the tumour to the chemotherapeutic agent was observed and Cp2 TiCl2 seemed to be an interesting candidate to bring into the clinic. The major challenge for a clinical application of Cp2 TiCl2 as an anticancer agent is presented by its hydrolytic instability at pH below 5.5, where both chlorides are lost. Even at neutral pH, research has shown that the Cp rings are lost quickly, resulting in the formation of hydrolysed products which are water insoluble. Therefore, it was necessary to develop a formulation before this compound could be brought into clinical trials. For its use in clinical trials, Cp2 TiCl2 was formulated as a lyophilised powder in malate buffer (pH = 3.2) or malic acid (pH = 3.5). Cp2 TiCl2 , formulated as a water-soluble powder, has entered phase I and phase II clinical trials. Results from phase I clinical trials were used to establish the maximum tolerable dose and to define the pharmacokinetic properties. Dose-limiting toxicities have been identified, as reversible damage to the liver and kidneys. Phase II clinical trials concentrated on the effect of Cp2 TiCl2 treatment of renal cell cancer and metastatic breast cancer. Unfortunately, the encouraging effect seen in preclinical studies could not be repeated in the clinical studies. The tumours did not show a significant response to Cp2 TiCl2 , and therefore clinical trials were discontinued. Budotitane is an octahedral Ti(IV) complex that was developed as a potential anticancer agent around the same time as the anticancer properties of Cp2 TiCl2 were discovered. Budotitane can be synthesised by reacting TiCl4 with the appropriate diketonate in an anhydrous solvent. Important structural features are the unsubstituted aromatic rings, which positively contribute to the cytotoxic activity, and the hydrolysable group. The latter group seems to be less important in regard to the cytotoxicity of budotitane but crucial for formu- lation purposes as it seems to determine the aqueous stability. Research has shown that the aqueous stability increases with the hydrolysable group in the order: I− < Br− < Cl− < F− < OR− (Figure 8.20). Another major drawback for the clinical use of budotitane is the presence of isomers. There is the possi- bility of a cis or trans arrangement of the —OC2 H5 group, with the cis arrangement believed to be the more stable one. Furthermore, the β-diketonato ligand is not symmetrical. This means that there are eight possible arrangements in this octahedral scheme. In solution, there is a mixture of isomers and it is difficult to isolate the isomers. So far, it is not known which isomer exhibits the anticancer activity (Figure 8.21). Preclinical studies using human xenografts in nude mice highlighted the potential of budotitane as novel chemotherapeutic agent in 1984. Budotitane showed cytotoxic activity comparable to cisplatin. Keppler et al. intensively studied its antitumour activity in a variety of animal models and found specific activity against Ehrlich ascetic tumour and colon cancer. Budotitane, similar to Cp2 TiCl2 , is prone to hydrolysis in conjunction with a low aqueous solubility. Therefore, significant research went into the development of an appropriate for- mulation. Several clinical studies were performed using cremophor EL as a nonionic surfactant, which has been used as a vehicle for the solubilisation of a wide variety of hydrophobic drugs (cremophor). Furthermore, 1,2-polyethyleneglycol has been added to encourage the coprecipitation of the drug. All three ingredients (budotitane, cremophor EL and 1,2-polyethyleneglycol) are dissolved in anhydrous ethanol and mixed, and the solvent is then evaporated off. Using this procedure, micelles containing 100–200 mg/100 ml of active pharmaceutical ingredient are formed, which are stable for a few hours. Budotitane went through several phase I and phase II clinical trials. Budotitane was administered as intravenous (IV) infusion twice a week to patients with solid tumours refractory to previous treatments. Cardiac arrhythmia was identified as the dose-limiting 2Hbzac + TiCl4 → [Ti(bzac)2 (OEt)2 ] + 4HCl Figure 8.20 Synthesis of budotitane Organometallic Chemistry 197 CH3 H3C O H3C O O O OC2H5 O OC2H5 O OC2H5 Ti Ti Ti O OC2H5 O OC2H5 O OC2H5 O H3C O O CH3 CH3 cis,cis,cis-Λ-[Ti(bzac)2(OEt)2] cis,cis,trans-Λ-[Ti(bzac)2(OEt)2] cis,trans,cis-Λ-[Ti(bzac)2(OEt)2] OC2H5 CH3 H3C CH3 OC2H5 O O O O Ti Ti O O O O OC2H5 OC2H5 H3C trans,trans,trans-[Ti(bzac)2(OEt)2] trans,cis,cis-[Ti(bzac)2(OEt)2] Figure 8.21 Isomers of budotitane side effect. Unfortunately, problems with the formulation, such as the existence of isomers and the difficulty in analysing and characterising the loaded micelles, led to a discontinuation of the clinical trials. Poor water solubility and fast hydrolysis are the main problems for the use of titanocene or titanium-based compounds as anticancer agents. Nevertheless, this intensive research in the 1980s and 1990s has encouraged further research as described in the following. 8.4.2 Further developments of titanocenes as potential anticancer agents As mentioned, poor aqueous solubility and instability in water are the main problems that restrict titanocenes in clinical applications. McGowan and coworkers renewed the interest in research in this area with their elegant synthesis of ring-substituted cationic titanocene dichloride derivatives. The idea was based on the introduction of charges in order to improve the aqueous solubility. Indeed, the resulting cationic titanocenes are more water-soluble and show a significant cytotoxic activity especially against cisplatin-resistant ovarian cancer (Figure 8.22). Following on from these results, Tacke and coworkers based their research on novel synthetic methods starting from substituted fulvenes. A fulvenes is an organic molecule with the chemical formula C6 H6 , which consists of a five-membered ring system and can be used to easily introduce functional groups into titanocenes (Figure 8.23). Reaction of substituted fulvenes with titanium dihalides via a reductive dimerisation process leads to the so-called ansa-titanocenes, which are normally used as catalysts. These ansa-titanocenes are characterised by a carbon–carbon bridge between the cyclopentadienyl (Cp) rings, which restricts the geometry of the Cp 198 Essentials of Inorganic Chemistry + R M N CI CI Figure 8.22 Examples of substituted titanocenes 1 R R Figure 8.23 Chemical structure of fulvene MCI2 CI M CI Figure 8.24 Synthesis of ansa-titanocenes using reductive dimerisation of fulvenes rings. In vitro testing showed that they exhibit a moderate cytotoxic activity, which is similar or better than Cp2 TiCl2 when tested against a model of renal cell cancer (Figure 8.24). The reaction of substituted fulvenes with superhydride (LiBEt3 H) in a so-called hydridolithiation reaction or the reaction with an organolithium compound in a carbolithiation reaction followed by transmetallation with titanium tetrachloride allows access to a variety of substituted titanocenes. Hydridolithiation allows access to benzyl-bridged titanocenes, whilst titanocenes obtained via carbolithiation typically contain more functional groups. This can be of advantage, for example, for the introduction of groups that can be eas- ily ionised in order to improve the water solubility of these titanocenes, but can also be a disadvantage as additional stereocentres are potentially introduced (Figures 8.25 and 8.26). These titanocenes have been intensively studied with regard to their potential anticancer activity. It has been shown that some of these compounds are active against a variety of human cancer types. The so-called titanocene Y has been the most intensively studied titanocene of this series. It has shown good activity against Organometallic Chemistry 199 R R H H H 2 + LiBEt3H 2 Li+ TiCI4 R R CI M CI Figure 8.25 Synthesis of benzyl-substituted titanocenes using hydridolithiation (Adapted from.) aryl aryl aryl aryl H H aryl Li TiCI4 H 2 Li aryl + 2 2 CI aryl M aryl CI Figure 8.26 Synthesis of substituted titanocenes using carbolithiation a model of renal cell cancer as well as other human cancer cell lines in a variety of in vitro experiments (Figure 8.27). In general, titanocenes obtained via these methods reached IC50 values in the low micromolar range when tested against a renal cell cancer model. This represents an up to 2000-fold improvement compared to Cp2 TiCl2. Nevertheless, the main problems are the potential presence of stereocentres and still a poor aqueous solubility. Further research has been carried out with the aim to replace the chloride ligands by other groups. This includes the incorporation of chelating ligands similar to the research undertaken for cisplatin. Nevertheless, this research did not result in any major improvement with regard to the cytotoxic activity. Also, some initial formulation studies have been carried out with only limited success. The mode of action of these titanocenes is so far not clear. It is believed that they coordinate to DNA via its phosphate backbone. It has also been shown that they can use transferrin as a transporter molecule into the cancer cell. 200 Essentials of Inorganic Chemistry MeO CI M CI OMe Figure 8.27 Chemical structure of titanocene Y 8.5 Vanadocenes Vanadium, with chemical symbol V and atomic number 23, is a member of the d-block metals and belongs to group 5 of the periodic table of elements (Figure 8.28). Vanadium can be found in the earth’s crust in numerous minerals and is isolated from ores mostly as a by-product. Its main application is in the steel industry, where it is used as an alloy in combination with iron. Vanadium pentaoxide is also being used as a catalyst for the production of sulfuric acid. The metal vanadium has very similar properties to titanium. Therefore, it is not surprising that its metallocene, vanadium dichloride, was also subjected to research as a potential anticancer agent. H He Li Be B C N O F Ne Na Mg Al Si P S Cl Ar K Ca Sc Ti V Cr Mn Fe Co Ni Cu Zn Ga Ge As Se Br Kr Rb Sr Y Zr Nb Mo Tc Ru Rh Pd Ag Cd In Sn Sb Te I Xe La- Cs Ba Hf Ta W Re Os Ir Pt Au Hg Tl Pb Bi Po At Rn Lu Ac- Fr Ra Rf Db Sg Bh Hs Mt Ds Rg Uub Lr Figure 8.28 Periodic table of elements; the element vanadium is highlighted Organometallic Chemistry 201 [VO4 ]3− → [VO3 × OH]2− → · · · → [V3 O9 ]3− → V2 O5 × (H2 O)n → [V10 O28 ]6− → [VO2 ]+ pH 12 pH 9 pH 6.5 pH 2.2 pH < 1 Figure 8.29 Vanadium oxide formation depending on pH O v O O O Figure 8.30 Chemical structure of vanadate Vanadium is easily passivated by an oxide film, and the metal is insoluble in nonoxidising acids. Typical oxidation states are +II, +III, +IV and +V, whilst the biologically active oxidation states are +IV and +V. Vanadium reacts to vanadium halide by reacting the metal with the corresponding halogen under heating, whilst it also reacts with oxygen with the formation of V2 O5. Vanadium (+V) oxides are amphoteric and, as a result, vanadates (VO4 3− ) and dioxovanadium ions (VO2 + ) are formed in aqueous solutions depending on the pH (Figure 8.29). Vanadium is an essential trace metal in the human body, but still very little is known about its biological function. Vanadium is mainly found in its ionic state bound to proteins. As mentioned, the metal mostly occupies oxidation states +V and +IV in biological systems, resulting in electron configurations of [Ar]3d0 for V+5 and [Ar]3d1 for V+4. The chemical formula for the tetrahedral ion vanadate is written as VO4 3+ ; whereas the diatomic oxovanadium(+IV) ion, also called vanadyl, has the chemical formula VO2+ (Figure 8.30). Vanadium compounds are well known for their toxicity. The most famous example is the poisonous mush- room toadstool, Amanita muscaria. A. muscaria contains the toxic compound amavadin, which is a toxic octahedral vanadium complex (see Figure 8.31). N O O O O O O O O N O Figure 8.31 Chemical structure of amavadin 202 Essentials of Inorganic Chemistry Vanadate and vanadyl are known to cause adverse effects in mammals, including loss of body weight, gas- trointestinal problems, reproductive toxicity and morbidity. However, their toxicity depends on a variety of factors such as the chemical form, oxidation state, route of administration and duration of exposure. Never- theless, toxic effects of vanadate or vanadyl are observed only at dose levels significantly greater than usual uptake through diet. Nevertheless, it is important to improve the understanding of the adverse and toxic effects of vanadium compounds before any compound can be successfully developed for clinical use. 8.5.1 Vanadocene dichloride as anticancer agents Vanadocene dichloride [(𝜂 5 -C5 H5 )2 VCl2 , dichloro bis(𝜂 5 -cyclopentadienyl)vanadium(IV)] is structurally very similar to Cp2 TiCl2. It also consists of a metal centre with an oxidation number of +IV, in this case vanadium, and two Cp− and two chloride ligands. Vanadocene dichloride is a 17-electron complex containing an unpaired electron and is therefore paramagnetic (Figure 8.32). Vanadocene dichloride has found application as a catalyst for polymerisation reactions, but was also intensively studied as an anticancer agent in parallel to Cp2 TiCl2 because of their structural similarities. Vanadocene dichloride has proven to be even more effective than its titanium analogue as an antiproliferative agent against both animal and human cell lines in preclinical testing. The main problems are the difficult characterisation of the active vanadium compounds and their fast hydrolysis. Because of their paramagnetic character, it is difficult to apply standard classical analysis techniques such as NMR (nuclear magnetic resonance) to identify the antiproliferative vanadium species. Furthermore, vanadocene dichloride undergoes fast hydrolytic processes and is even more prone to hydrolysis than titanocene dichloride. This poses even more challenges for its potential clinical application. In recent years, researchers have shown renewed interest in the use of substituted vanadocene dichlorides as potential anticancer agents. A selection of substituted vanadocenes have been synthesised and tested for their cytotoxic activity against testicular cancer. Examples of these compounds include vanadocenes con- taining substituted cyclopentadienyl ligands and/or replacement groups for the chloride ligands – similar to the research being undertaken for cisplatin analogues. Results of in vitro studies show that these com- pounds exhibit good but variable cytotoxic activity depending on the substitution pattern and induce apoptosis (cell-induced cell death). Interestingly, only organometallic vanadium(+IV) complexes showed cytotoxic activity against testicular cancer. When the purely inorganic compound vanadyl(IV) sulfate was tested in the same study, no cytotoxic effect was observed at the same concentrations. It is also important to note that titanocene dichloride and other metallocenes had no cytotoxic effect against testicular cancer. It was con- cluded that the mode of action of vanadium-induced cytotoxicity must be different from that of titanocene dichloride and other metallocenes (Figure 8.33). In parallel to the research undertaken with substituted titanocene dichlorides as potential chemotherapeutic agents, some of their vanadocene analogues have been synthesised. Some examples include the hydrolithiation of fulvenes (see Section 8.4.2) and subsequent transmetallation with vanadium tetrachloride. The resulting CI V CI Figure 8.32 Chemical structure of vanadocene dichloride Organometallic Chemistry 203 O OCN V V O CI Figure 8.33 Examples of substituted vanadocenes dichlorides OMe MeO MeO CI V CI OMe MeO OMe Figure 8.34 Example of a substituted vanadocenes synthesised via transmetallation substituted vanadocene dichlorides were found to be highly toxic compounds when tested in vitro against a model of renal cell cancer and more potent than the corresponding titanocene. Further preclinical studies are still needed (Figure 8.34). 8.5.2 Further vanadium-based drugs: insulin mimetics Towards the end of the nineteenth century, inorganic vanadium compounds were under evaluation as potential treatment options for Diabetes Mellitus (DM) as so-called insulin mimetics. Sodium vanadate (Na3 V(+V)O4 ) was tested for its ability to lower glucose levels in the blood of candidates with and without DM. The inor- ganic vanadium compound showed mild effects in some of the patients suffering from DM, whilst no severe