Ismail Organic Chemistry Book 2022 PDF
Document Details
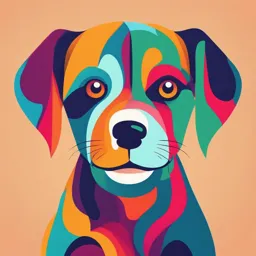
Uploaded by ObservantMoscovium
University of Hargeisa
2022
Tags
Summary
This book covers organic chemistry, focusing on unsaturated hydrocarbons. It delves into topics like organic and inorganic compounds, bonding characteristics, and structural formulas.
Full Transcript
Contents Chapter one: Unsaturated Hydrocarbons........................................................................................................ 1 1.1 Organic and Inorganic Compounds............................................................................................................ 1...
Contents Chapter one: Unsaturated Hydrocarbons........................................................................................................ 1 1.1 Organic and Inorganic Compounds............................................................................................................ 1 Organic chemistry........................................................................................................................................ 1 Inorganic chemistry...................................................................................................................................... 1 1.2 Bonding Characteristics of the Carbon Atom............................................................................................ 2 1.3 Hydrocarbons and Hydrocarbon Derivatives............................................................................................. 2 1.4 Alkanes: Acyclic Saturated Hydrocarbons.................................................................................................. 4 1.5 Structural Formulas.................................................................................................................................... 4 Skeletal structural formula.......................................................................................................................... 5 Line-Angle Structural Formulas.................................................................................................................... 5 Three dimensional Structural Representations........................................................................................... 6 1.6 Alkane Isomerism....................................................................................................................................... 7 Constitutional isomers................................................................................................................................. 8 1.7 IUPAC Nomenclature for Alkanes.............................................................................................................. 8 Continuous and branched- chain alkanes.................................................................................................... 8 Alkyl group (continuous).............................................................................................................................. 9 1.8 Classification of Carbon Atoms................................................................................................................ 16 1.9 Branched-Chain Alkyl Groups.................................................................................................................. 18 Complex Branched-Chain Alkyl Groups.......................................................................................................... 19 1.10 Cycloalkanes........................................................................................................................................... 20 IUPAC Nomenclature for Cycloalkanes.............................................................................................................. 21 1.11 Isomerism in Cycloalkanes..................................................................................................................... 23 Stereoisomers............................................................................................................................................ 23 1.12 Sources of Alkanes and Cycloalkanes.................................................................................................... 25 1.13 Physical Properties of Alkanes and Cycloalkanes.................................................................................. 26 1.14 Chemical Properties of Alkanes and Cycloalkanes................................................................................. 27 Combustion of alkanes............................................................................................................................... 27 Halogenation................................................................................................................................................ 27 A substitution reaction.............................................................................................................................. 27 I 1.15 Halogenated Alkanes and Cycloalkanes................................................................................................. 29 Nomenclature of Halogenated Alkanes..................................................................................................... 30 Physical Properties of Halogenated Alkanes.............................................................................................. 30 Concepts to Remember............................................................................................................................. 31 Chapter Two: Unsaturated Hydrocarbons..................................................................................................... 33 2.1 Unsaturated Hydrocarbons.................................................................................................................... 33 A functional group..................................................................................................................................... 33 Types of unsaturated hydrocarbons.......................................................................................................... 33 2.2 Characteristics of Alkenes and Cycloalkenes........................................................................................... 33 Alkenes....................................................................................................................................................... 33 Cycloalkenes............................................................................................................................................... 34 Dienes and trienes..................................................................................................................................... 34 2.3 IUPAC Nomenclature for Alkenes and Cycloalkenes............................................................................... 35 Common Names (Non-IUPAC Names........................................................................................................ 37 Alkenes as Substituents............................................................................................................................. 38 2.4 Line-Angle Structural Formulas for Alkenes............................................................................................. 38 2.5 Constitutional Isomerism in Alkenes....................................................................................................... 39 Determining Structural Formulas for Alkene Constitutional Isomers....................................................... 40 2.6 Cis–Trans Isomerism in Alkenes............................................................................................................... 41 Cis and Trans isomerism in substituted alkenes........................................................................................ 42 2.7 Physical Properties of Alkenes and Cycloalkenes.................................................................................... 43 2.8 Chemical Reactions of Alkenes................................................................................................................ 44 An addition reaction.................................................................................................................................. 44 Symmetrical Addition Reactions................................................................................................................ 44 Unsymmetrical Addition Reactions............................................................................................................ 46 2.9 Polymerization of Alkenes: Addition Polymers........................................................................................ 49 Substituted-Ethene Addition Polymers..................................................................................................... 50 Butadiene-Based Addition Polymers......................................................................................................... 51 Addition Copolymers................................................................................................................................. 51 2.10 Alkynes................................................................................................................................................... 52 IUPAC Nomenclature for Alkynes.............................................................................................................. 52 Physical and Chemical Properties of Alkynes............................................................................................ 53 II 2.11 Aromatic Hydrocarbons......................................................................................................................... 54 2.12 Names for Aromatic Hydrocarbons....................................................................................................... 55 Benzene Derivatives with One Substituent............................................................................................... 55 Benzene Derivatives with Two Substituents.............................................................................................. 57 Benzene Derivatives with Three or More Substituents................................................................................... 59 Physical Properties and Sources of Aromatic Hydrocarbons..................................................................... 60 Chemical Reactions of Aromatic Hydrocarbons........................................................................................ 61 2.13 Fused-Ring Aromatic Hydrocarbons...................................................................................................... 62 Concepts to Remember............................................................................................................................. 62 Chapter 3: Alcohols, Phenols, and Ethers...................................................................................................... 64 3.1 Bonding Characteristics of Oxygen Atoms in Organic Compounds......................................................... 64 3.3 Nomenclature for Alcohols...................................................................................................................... 66 Polyhydroxy alcohols................................................................................................................................. 68 Isomerism for Alcohols.................................................................................................................................. 68 Methyl Alcohol (Methanol)........................................................................................................................ 69 Preparation of methanol............................................................................................................................ 69 Ethyl Alcohol (Ethanol)............................................................................................................................... 69 Preparation of ethanol............................................................................................................................. 70 Denatured alcohol..................................................................................................................................... 70 Ethylene Glycol (1,2-Ethanediol) and Propylene Glycol (1,2-Propanediol)............................................... 71 Glycerol (1, 2, 3-Propanetriol)................................................................................................................... 72 3.6 Physical Properties of Alcohols................................................................................................................ 72 Boiling Points and Water Solubilities......................................................................................................... 73 3.7 Preparation of Alcohols............................................................................................................................ 73 3.8 Classification of Alcohols.......................................................................................................................... 74 Combustion................................................................................................................................................ 76 Intramolecular Alcohol Dehydration......................................................................................................... 76 Elimination reaction................................................................................................................................... 77 Zaitsev’s rule.............................................................................................................................................. 78 Intermolecular Alcohol Dehydration......................................................................................................... 78 Condensation reaction............................................................................................................................... 78 Oxidation.................................................................................................................................................... 80 III Halogenation.............................................................................................................................................. 84 3.10 Phenols................................................................................................................................................... 84 Nomenclature for Phenols......................................................................................................................... 84 Physical and Chemical Properties of Phenols............................................................................................ 85 Acidity of Phenols...................................................................................................................................... 86 3.16 Nomenclature for Ethers....................................................................................................................... 87 Functional Group Isomerism...................................................................................................................... 90 Nomenclature for Thiols............................................................................................................................ 90 Chapter 4: Aldehydes and Ketones................................................................................................................ 93 4.1 The Carbonyl Group................................................................................................................................. 93 4.2 The Aldehyde and Ketone Functional Groups......................................................................................... 93 Nomenclature for Aldehydes..................................................................................................................... 93 4.3 Ketones.................................................................................................................................................... 95 4.4 Nomenclature for Ketones....................................................................................................................... 96 Isomerism for Aldehydes and Ketones...................................................................................................... 98 4.5 Preparation of Aldehydes and Ketones................................................................................................... 98 4.6 Oxidation and Reduction of Aldehydes and Ketones.............................................................................. 99 Reduction of Aldehydes and Ketones...................................................................................................... 100 Chapter 5: Carboxylic Acids, Esters and other Acid Derivatives.................................................................. 102 5.1 carboxylic acids...................................................................................................................................... 102 5.2 IUPAC Nomenclature for Carboxylic Acids............................................................................................. 102 Dicarboxylic Acids.................................................................................................................................... 104 Aromatic Carboxylic Acids........................................................................................................................ 104 5.3 Common Names for Carboxylic Acids.................................................................................................... 106 Preparation of Carboxylic Acids............................................................................................................... 106 5.4 Structure of Esters................................................................................................................................. 106 5.5 Preparation of Esters............................................................................................................................. 107 Ester Hydrolysis........................................................................................................................................ 110 Ester Saponification................................................................................................................................. 110 Chapter 6: Amines and Amides.................................................................................................................... 110 6.1 Structure and Classification of Amines.................................................................................................. 110 6.2 Nomenclature for Amines...................................................................................................................... 112 IV 6.3 Structure and Classification of Amides.................................................................................................. 113 6.4 Nomenclature for Amides...................................................................................................................... 114 6.5 Preparation of Amides........................................................................................................................... 116 Amidification reaction.............................................................................................................................. 117 V Chapter one: Unsaturated Hydrocarbons 1.1 Organic and Inorganic Compounds Organic chemistry is the study of hydrocarbons (compounds of carbon and hydrogen) and their derivatives. Organic chemistry is the study of carbon compounds with the exception of the simple carbon compounds: 1. Oxides 2. Carbonates 3. Hydrogen carbonates 4. Cyanides 5. carbides Inorganic chemistry is the study of all substances other than hydrocarbons and their derivatives. There are approximately 10 million organic compounds and only an estimated 1.7 million inorganic compounds (Figure 1). This is an approximately 6:1 ratio between organic and inorganic compounds. 1 1.2 Bonding Characteristics of the Carbon Atom Why does the element carbon form six times as many compounds as all the other elements combined? The answer is that carbon atoms have the unique ability to bond to each other in a wide variety of ways that involve long chains of carbon atoms or cyclic arrangements (rings) of carbon atoms. Sometimes both chains and rings of carbon atoms are present in the same molecule. Bonding requirements for carbon atom To obtain full outer shell (octet rule), carbon require to form four covalent bonds. Carbon can meet this four-bond requirement in three different ways: 1. By bonding to four other atoms. This situation requires the presence of four single bonds. 2. By bonding to three other atoms. This situation requires the presence of two single bonds and one double bond. 3. By bonding to two other atoms. This situation requires the presence of either two double bonds or a triple bond and a single bond. 1.3 Hydrocarbons and Hydrocarbon Derivatives A hydrocarbon is a compound that contains only carbon and hydrogen atoms. Thousands of hydrocarbons are known. A hydrocarbon derivative is a compound that contains carbon and hydrogen and one or more additional elements. 2 Additional elements commonly found in hydrocarbon derivatives include O, N, S, P, F, Cl, and Br. Millions of hydrocarbon derivatives are known. Hydrocarbons may be divided into two large classes: saturated and unsaturated: A saturated hydrocarbon is a hydrocarbon in which all carbon–carbon bonds are single bonds. Saturated hydrocarbons are the simplest type of organic compound. An unsaturated hydrocarbon is a hydrocarbon in which one or more carbon–carbon multiple bonds (double bonds, triple bonds, or both) are present. In general, saturated and unsaturated hydrocarbons undergo distinctly different chemical reactions. Two categories of saturated hydrocarbons exist, those with acyclic carbon atom arrangements and those with cyclic carbon atom arrangements. The term acyclic means “not cyclic.” The following notations contrast simple acyclic and cyclic arrangements of six-carbon atoms. 3 1.4 Alkanes: Acyclic Saturated Hydrocarbons An alkane is a saturated hydrocarbon in which the carbon atom arrangement is acyclic. Thus an alkane is a hydrocarbon that contains only carbon–carbon single bonds (saturated) and has no rings of carbon atoms (acyclic). The molecular formulas of all alkanes fit the general formula CnH2n+2, where n is the number of carbon atoms present. Note also that the geometrical arrangement of atoms about each carbon atom is tetrahedral, an arrangement consistent with the principles of VSEPR theory 1.5 Structural Formulas A structural formula is a two-dimensional structural representation that shows how the various atoms in a molecule are bonded to each other. Structural formulas are of two types: expanded structural formulas and condensed structural formulas. An expanded structural formula is a structural formula that shows all atoms in a molecule and all bonds connecting the atoms. A condensed structural formula is a structural formula that uses groupings of atoms, in which central atoms and the atoms connected to them are written as a group, to convey molecular structural information. The expanded and condensed structural formulas for methane, ethane, and propane follow. 4 The condensed structural formulas of hydrocarbons in which a long chain of carbon atoms is present are often condensed even more. The formula can be further abbreviated as: Where parentheses and a subscript are used to denote the number of – CH2--groups in the chain Skeletal structural formula A skeletal structural formula is a structural formula that shows the arrangement and bonding of carbon atoms present in an organic molecule but does not show the hydrogen atoms attached to the carbon atoms. Line-Angle Structural Formulas A line-angle structural formula is a structural representation in which a line represents a carbon– carbon bond and a carbon atom is understood to be present at every point where two lines meet and at the ends of lines. Line-angle structural formulas for the alkanes propane, butane, and pentane are as follows: 5 Three dimensional Structural Representations 6 1.6 Alkane Isomerism Isomers are compounds that have the same molecular formula (that is, the same numbers and kinds of atoms) but that differ in the way the atoms are arranged. Isomers, even though they have the same molecular formula, are always different compounds with different properties. Contrasting the two C4H10 isomers structurally, note that butane has a chain of four carbon atoms. It is an example of a continuous-chain alkane. A continuous- chain alkane is an alkane in which all carbon atoms are connected in a continuous non-branching chain. The other C4H10 isomer, isobutane, has a chain of three carbon atoms with the fourth carbon attached as a branch on the middle carbon of the three-carbon chain. It is an example of a branched-chain alkane. A branched-chain alkane is an alkane in which one or more branches (of carbon atoms) are attached to a continuous chain of carbon atoms. There are three isomers for alkanes with five carbon atoms (C5H12): The number of possible alkane isomers increases dramatically with increasing numbers of carbon atoms in the alkane, as shown in Table 7 Several different types of isomerism exist. The alkane isomerism examples discussed in this section are examples of constitutional isomerism. Constitutional isomers are isomers that differ in the connectivity of atoms, that is, in the order in which atoms are attached to each other within molecules. Constitutional isomers are also frequently called structural isomers. The condensed structural formulas for branched-chain alkanes can be further condensed to give linear (straight-line) condensed structural formulas. The linear condensed structural formula for the alkane is: Groups in parentheses in such formulas are understood to be attached to the car- bon atom that precedes the group in the structural formula 1.7 IUPAC Nomenclature for Alkanes Rules were formulated and are updated periodically by the International Union of Pure and Applied Chemistry (IUPAC), are known as IUPAC rules. The advantage of the IUPAC naming system is that it assigns each compound a name that not only identifies it but also enables its structural formula to be drawn. Continuous and branched- chain alkanes The existence of both continuous-chain alkanes and branched- chain alkanes was noted, IUPAC names for the first ten continuous-chain alkanes are given in Table 1.2 8 Note that all of these names end in -ane, Note also that beginning with the five-carbon alkane, Greek numerical prefixes are used to denote the actual number of carbon atoms in the continuous chain. The key to name branched-chain alkanes is to know the name of the branch or branches that are attached to the main carbon chain. These branches are formally called substituents A substituent is an atom or group of atoms attached to a chain (or ring) of carbon atoms. Note that substituent is a general term that applies to carbon-chain attachments in all organic molecules, not just alkanes. Alkyl group (continuous) For branched-chain alkanes, the substituents are specifically called alkyl groups. An alkyl group is the group of atoms that would be obtained by removing a hydrogen atom from an alkane. The two most commonly encountered alkyl groups are the two simplest: the one-carbon and two- carbon alkyl groups. Their formulas and names are In these formulas the “squiggle” on the left denotes the point of attachment to the carbon chain. Note that alkyl groups do not lead a stable, independent existence; that is, they are not molecules. They are always found attached to another entity (usually a carbon chain). Table 1.3 Names for the First Six Continuous-Chain Alkyl Groups 9 IUPAC rules for naming branched-chain alkanes are as follows: Rule 1: Identify the longest continuous carbon chain (the parent chain), which may or may not be shown in a straight line, and name the chain. CH3CH2CH (CH3) CH2 CH2C H3 condensed formula Rule 2: Number the carbon atoms in the parent chain from the end of the chain nearest a substituent (alkyl group). There are always two ways to number the chain (either from left to right or from right to left). This rule gives the first-encountered alkyl group the lowest possible number. 10 Rule 3: If only one alkyl group is present, name and locate it (by number), and prefix the number and name to that of the parent carbon chain. Note that the name is written as one word, with a hyphen between the number and the name of the alkyl group. Rule 4: If two or more of the same kind of alkyl group are present in a molecule, indicate the number with a Greek numerical prefix (di-, tri-, tetra-, penta-, and so forth). In addition, a number specifying the location of each identical group must be included. These position numbers, separated by commas, precede the numerical prefix. Numbers are separated from words by hyphens. 11 Note that the numerical prefix di- must always be accompanied by two numbers, tri- by three, and so on, even if the same number is written twice, as in 3, 3-dimethylpentane. Rule 5: When two kinds of alkyl groups are present on the same carbon chain, number each group separately, and list the names of the alkyl groups in alphabetical order. Note that ethyl is named first in accordance with the alphabetical rule. Note that the prefix di- does not affect the alphabetical order; ethyl precedes propyl Rule 6: Follow IUPAC punctuation rules, which include the following: (1) Separate numbers from each other by commas. (2) Separate numbers from letters by hyphens. (3) Do not add a hyphen or a space between the last-named substituent and the name of the parent alkane that follows. 12 Give the IUPAC name for each of the following branched-chain alkanes a. 2,3-dimethylpentane b. 4-ethyl-2,7-dimethyloctane Practice Exercise Give the IUPAC name for each of the following alkanes Generating the Structural Formula of an Alkane from Its IUPAC Name Draw the condensed structural formula for 3-ethyl-2,3-dimethylpentane 13 Practice Exercise Determining Structural Formulas for and Naming Alkane Constitutional Isomers Draw skeletal structural formulas for, and assign IUPAC names to, all C6H14 alkane constitutional isomers. Solution Table 1.1 indicates that there are five constitutional isomers with the chemical formula C6H14. Part of the purpose of this example is to consider the “thinking pattern” needed to identify these five isomers. There are two concepts embedded in the thinking pattern 1) Carbon chains of varying length are examined for isomerism possibilities, starting with the chain of maximum length and then examining increasingly shorter chain lengths. 2) Substituents are added to the various carbon chains, with the number of added carbons determined by the chain length. Various location possibilities for the substituents are examined. Step 1: A C6 carbon chain is the longest chain possible; it contains all available carbon atoms. This is the molecule hexane, the first of the five constitutional isomers. No substituents are added to this chain, as that would increase the carbon count beyond six. Step 2: Decreasing the carbon-chain length by one gives a C5 chain. A methyl group must be added to the chain to bring the carbon count back up to six. Theoretically, there are five possible positions for the methyl group: 14 These five structures do not represent five new isomers. The first and last structures represent two alternate ways of drawing the molecule hexane, the first isomer. A methyl group (or any alkyl group) added to the end carbons of a carbon chain will always increase the chain length. The second and third structures do represent new isomers: The fourth of the five structures is not a new isomer. Numbering its carbon chain from the right end shows that it is 2-methylpentane rather than 4-methylpentane. Thus the second and fourth structures are two representations of the same molecule. Step 3: Decreasing the chain length to four carbon atoms is the next consideration. Two carbon atoms must now be added as attachments. This can be done in two ways—dimethyl and ethyl. Examining dimethyl possibilities first, eliminating structures that have methyl groups on terminal carbon atoms gives the following possibilities. The first and second structures are the same; both represent the molecule 2,2-dimethylbutane, a fourth isomer. The third structure, 2,3-dimethylbutane, is different from the other two. It is the fifth isomer. What about ethyl butanes? 15 Neither of these structures is a new isomer because both have a five-carbon chain. Both structures are actually depictions of 3-methylpentane, one of the isomers previously identified. Step 4: A chain length of three does not generate any new isomers. A trimethyl structure is impossible, as the middle carbon atom, the only carbon to which substituents can be attached, would have five bonds. An ethyl methyl structure extends the carbon chain length, as does a single three-carbon attachment. Thus, there are five constitutional isomers: hexane, 2-methylpentane, 3-methylpentane, 2,2- dimethylbutane, and 2,3-dimethylbutane. 1.8 Classification of Carbon Atoms Each of the carbon atoms within a hydrocarbon structure can be classified as a primary (1°), secondary (2°), tertiary (3°), or quaternary (4°) carbon atom. 16 A primary carbon atom is a carbon atom in an organic molecule that is directly bonded to one other carbon atom. Both carbon atoms in ethane are primary carbon atoms. A secondary carbon atom is a carbon atom in an organic molecule that is directly bonded to two other carbon atoms. A propane molecule contains a secondary carbon atom as well as two primary carbon atoms. A tertiary carbon atom is a carbon atom in an organic molecule that is directly bonded to three other carbon atoms. The molecule 2-methylpropane contains a tertiary carbon atom. A quaternary carbon atom is a carbon atom in an organic molecule that is directly bonded to four other carbon atoms. The molecule 2,2-dimethylpropane contains a quaternary carbon atom. The alkane 2,2,3-trimethyl pentane is the simplest alkane in which all four types of carbon atoms (1°, 2°, 3°, and 4°) are present. 17 1.9 Branched-Chain Alkyl Groups Methyl, ethyl, n-propyl and n-butyl are continuous chain alkyl groups Isopropyl, isobutyl, s- butyl and t-butyl are branched -chain alkyl groups For the two groups whose names contain the prefix iso-, the common structural feature is an end-of-chain arrangement that contains two methyl groups. For the secondary-butyl group, the point of attachment of the group to the main carbon chain involves a secondary carbon atom. For the tertiary-butyl group, the point of attachment of the group to the main carbon chain involves a tertiary carbon atom. The name secondary-butyl is often shortened to sec-butyl or simply s-butyl. Similarly, tertiary-butyl is often written as tert- butyl or t-butyl. A single compound or group can have several acceptable names, but no two compounds or groups can have the same name An alternate name for the sec-butyl group is the 1,1-dimethylethyl groups. 18 Two examples of alkanes containing branched-chain alkyl groups follow. Complex Branched-Chain Alkyl Groups “Simple” names, such as isobutyl and tert-butyl, do not exist for most branched-chain alkyl groups containing five or more carbon atoms. The IUPAC system provision for naming such larger groups involves naming them as if they were themselves compounds. The following rules are used Rule 1:The longest continuous carbon chain that begins at the point of attachment of the alkyl group becomes the base name. Rule 2: The base chain is numbered beginning at the point of attachment. 19 Rule 3: Substituents on the base chain are listed in alphabetical order, using numerical prefixes when necessary, and substituent locations are designated using numbers. Two additional examples of IUPAC nomenclature for complex branched-chain alkyl groups are 1.10 Cycloalkanes A cycloalkane is a saturated hydrocarbon in which carbon atoms connected to one another in a cyclic (ring) arrangement are present. The simplest cycloalkane is cyclopropane, which contains a cyclic arrangement of three carbon atoms The general formula for Cycloalkanes is CnH2n. The general formula for Cycloalkanes is CnH2n. Thus a given cycloalkane contains two fewer hydrogen atoms than an alkane with the same number of hydrogen atoms Line-angle structural formulas are generally used to represent cycloalkane structures. The line- angle structural formula for cyclopropane is a triangle, that for cyclobutane a square, that for cyclopentane a pentagon, and that for cyclohexane a hexagon. 20 In such structures, the intersection of two lines represents a CH2 group. Three- and four-way intersections of lines are possible when substituents are present on a ring. A three-way intersection represents a CH group, and a four-way intersection is simply a carbon atom. Cycloalkanes of ring sizes ranging from 3 to over 30 are found in nature, and, in principle, there is no limit to ring size. Five-membered rings (cyclopentane) and six- membered rings (cyclohexane) are especially abundant in nature IUPAC Nomenclature for Cycloalkanes 1. If there is just one ring substituent, it is not necessary to locate it by number. 2. When two ring substituents are present, the carbon atoms in the ring are numbered beginning with the substituent of higher alphabetical priority and preceding in the direction (clockwise or counterclockwise) that gives the other substituent the lower number. 3. When three or more ring substituents are present, ring numbering begins at the substituent that leads to the lowest set of location numbers. When two or more equivalent numbering sets exist, alphabetical priority among substituents determines the set used. 21 Solution a. This molecule is a cyclobutane (four-carbon ring) with a methyl substituent. The IUPAC name is simply methylcyclobutane. No number is needed to locate the methyl group because all four ring positions are equivalent. b. This molecule is a cyclopentane with ethyl and methyl substituents. The numbers for the carbon atoms that bear the substituents are 1 and 2. On the basis of alphabetical priority, the number 1 is assigned to the carbon atom that bears the ethyl group. The IUPAC name for the compound is 1-ethyl-2-methylcyclopentane. c. This molecule is a dimethylpropylcyclohexane. Two different 1,2,3 numbering systems exist for locating the substituents. On the basis of alphabetical priority, the numbering system that has carbon 1 bearing a methyl group is used; methyl has alphabetical priority over propyl. Thus the compound name is 1,2-dimethyl-3-propylcyclohexane. When a ring system contains fewer carbon atoms than an alkyl group attached to it, the compound is named as an alkane rather than as a cycloalkane; the ring is named as a cycloalkyl group. 22 1.11 Isomerism in Cycloalkanes Constitutional isomers are possible for cycloalkanes that contain four or more carbon atoms. For example, there are five cycloalkane constitutional isomers that have the formula C5H10: one based on a five-membered ring, one based on a four membered ring, and three based on a three- membered ring. These isomers are A second type of isomerism, called stereoisomerism, is possible for some substituted cycloalkanes. Whereas constitutional isomerism results from differences in connectivity, stereoisomerism results from differences in configuration Stereoisomers are isomers that have the same molecular and structural formulas but different orientations of atoms in space. Several forms of stereoisomerism exist. The form associated with cycloalkanes is called cis–trans isomerism. Cis– trans isomers are isomers that have the same molecular and structural formulas but different orientations of atoms in space because of restricted rotation about bonds. In alkanes, there is free rotation about all carbon–carbon bonds. In cycloalkanes, the ring structure restricts rotation for the carbon atoms in the ring. The consequence of this lack of rotation in a cycloalkane is the creation of “top” and “bottom” positions for the two attachments on each of the ring carbon atoms. This “top–bottom” situation leads to cis–trans isomerism in cycloalkanes in which each of two ring carbon atoms bears two different attachments. Consider the following two structures for the molecule 1,2-dimethylcyclo- pentane. In structure A, both methyl groups are above the plane of the ring (the “top” side). In structure B, one methyl group is above the plane of the ring (the “top” side) and the other below it (the 23 “bottom” side). Structure A cannot be converted into structure B without breaking bonds. Hence structures A and B are isomers; there are two 1,2-dimethylcyclopentanes. The first isomer is called cis-1,2-dimethylcyclopentane and the second trans-1,2-dimethylcyclopentane. Cis- is a prefix that means “on the same side.” In cis-1,2-dimethylcyclopentane, the two methyl groups are on the same side of the ring. Trans- is a prefix that means “across from.” In trans-1,2- dimethylcyclopentane, the two methyl groups are on opposite sides of the ring. Determine whether cis–trans isomerism is possible for each of the following cycloalkanes. If so, then draw structural formulas for the cis and trans isomers. Solution a. Cis–trans isomerism is not possible because there are not two substituents on the ring. b. Cis–trans isomerism is not possible. There are two substituents on the ring, but they are on the same carbon atom. Each of two different carbons must bear substituents. 24 1.12 Sources of Alkanes and Cycloalkanes Alkanes and cycloalkanes are not “laboratory curiosities” but, rather, two families of extremely important naturally occurring compounds. Natural gas and petroleum (crude oil) constitute their largest and most important natural source. Deposits of these resources are usually associated with underground dome-shaped rock formations. When a hole is drilled into such a rock formation, it is possible to recover some of the trapped hydrocarbons—that is, the natural gas and petroleum 25 1.13 Physical Properties of Alkanes and Cycloalkanes 1. Alkanes and cycloalkanes are insoluble in water. Water molecules are polar, and alkane and cycloalkane molecules are non-polar. Molecules of unlike polarity have limited solubility in one another 2. Alkanes and cycloalkanes have densities lower than that of water. Alkane and cycloalkane densities fall in the range 0.6 g/mL to 0.8 g/mL, compared with water’s density of 1.0 g/ml. When alkanes and cycloalkanes are mixed with water, two layers form (because of insolubility), with the hydrocarbon layer on top (because of its lower density). 3. The boiling points of continuous-chain alkanes and cycloalkanes increase with an increase in carbon-chain length or ring size. Branching on a carbon chain lowers the boiling point of an alkane. A comparison of the boiling points of unbranched alkanes and their 2-methyl-branched isomers is given in this Figure. Branched alkanes are more compact, with smaller surface areas than their straight-chain isomers. Cycloalkanes have higher boiling points than their noncyclic counterparts with the same number of carbon atoms. These differences are due in large part to cyclic systems having more rigid and more symmetrical structures. Cyclopropane and cyclobutane are gases at room temperature, and cyclopentane through cyclooctane are liquids at room temperature. 26 1.14 Chemical Properties of Alkanes and Cycloalkanes Alkanes are the least reactive type of organic compound. They can be heated for long periods of time in strong acids and bases with no appreciable reaction. Strong oxidizing agents and reducing agents have little effect on alkanes. Alkanes are not absolutely unreactive. Two important reactions that they undergo are combustion, which is reaction with oxygen, and halogenations, which is reaction with halogens. Combustion of alkanes A combustion reaction is a chemical reaction between a substance and oxygen (usually from air) that proceeds with the evolution of heat and light (usually as a flame). Alkanes readily undergo combustion when ignited. When sufficient oxygen is present to support total combustion, carbon dioxide and water are the products. Halogenation A halogenation reaction is a chemical reaction between a substance and a halogen in which one or more halogen atoms are incorporated into molecules of the substance. Halogenation of an alkane produces a hydrocarbon derivative in which one or more halogen atoms have been substituted for hydrogen atoms. An example of an alkane halogenation reaction is Alkane halogenation is an example of a substitution reaction, a type of reaction that occurs often in organic chemistry. A substitution reaction is a chemical reaction in which part of a small reacting molecule replaces an atom or a group of atoms on a hydrocarbon or hydrocarbon derivative. A general equation for the substitution of a single halogen atom for one of the hydrogen atoms of an alkane is 27 Note the following features of this general equation: 1. The notation R-H is a general formula for an alkane. R- in this case represents an alkyl group. Addition of a hydrogen atom to an alkyl group produces the parent hydrocarbon of the alkyl group. 2. The notation R-X on the product side is the general formula for a halogenated alkane. X is the general symbol for a halogen atom. 3. Reaction conditions are noted by placing these conditions on the equation arrow that separates reactants from products. Halogenation of an alkane requires the presence of heat or light. The symbol R is used frequently in organic chemistry and will be encountered in numerous generalized formulas in subsequent chapters; it always represents a generalized organic group in a structural formula. An R group can be an alkyl group—methyl, ethyl, propyl, etc.—or any number of other organic groups. In halogenation of an alkane, the alkane is said to undergo fluorination, chlorination, bromination, or iodination, depending on the identity of the halogen reactant. Chlorination and bromination are the two widely used alkane halogenation reactions. Fluorination reactions generally proceed too quickly, and iodination reactions go too slowly. Methane and chlorine, when heated to a high temperature or in the presence of light, react as follows: The reaction does not stop at this stage, however, because the chlorinated methane product can react with additional chlorine to produce polychlorinated products. 28 1.15 Halogenated Alkanes and Cycloalkanes A halogenated alkane is an alkane derivative in which one or more halogen atoms are present. Similarly, a halogenated cycloalkane is a cycloalkane derivative in which one or more halogen atoms are present. Produced by halogenation reactions, these two types of compounds represent the first class of hydrocarbon derivatives formally considered in this text. Alkanes have the general molecular formula CnH2n+2.Halogenated alkanes containing one halogen atom have the general molecular formula CnH2n+1X; a halogen atom has replaced a hydrogen atom. If two halogen atoms are present in a halogenated alkane, the general molecular formula is CnH2nX2. Since cycloalkanes have the general molecular formula CnH2n a halogenated cycloalkane with one halogen atom present will have a general molecular formula of CnH2n-1X. 29 Nomenclature of Halogenated Alkanes The IUPAC rules for naming halogenated alkanes are similar to those for naming branched alkanes, with the following modifications: 1. Halogen atoms, treated as substituents on a carbon chain, are called fluoro-, chloro-, bromo-, and iodo-. 2. When a carbon chain bears both a halogen and an alkyl substituent, the two substituents are considered of equal rank in determining the numbering system for the chain. The chain is numbered from the end closer to a substituent, whether it is a halo- or an alkyl group. 3. Alphabetical priority determines the order in which all substituents present are listed. The following names are derived using these rule adjustments. Simple halogenated alkanes can also be named as alkyl halides. These common (non-IUPAC) names have two parts. The first part is the name of the hydrocarbon portion of the molecule (the alkyl group). The second part (as a separate word) identifies the halogen portion, which is named as if it were an ion (chloride, bromide, and so on), even though no ions are present (all bonds are covalent bonds). The following examples contrast the IUPAC names and the common names (in parentheses) of selected halogenated alkanes. Physical Properties of Halogenated Alkanes Halogenated alkane boiling points are generally higher than those of the corresponding alkane. An important factor contributing to this effect is the polarity of carbon–halogen bonds, which results in increased dipole–dipole interactions. Two general trends relative to boiling points and melting points of halogenated hydrocarbons containing a single halogen atom are: 1. Boiling points and melting points increase as the size of the alkyl group present increases. This is due to increasing intermolecular forces associated with increased molecular surface area. 2. Boiling points and melting points increase as the size of the halogen atom increases from fluorine (F) to iodine (I). 30 Halogenated hydrocarbons do not have hydrogen-bonding capabilities since all hydrogen atoms are bonded to carbon atoms. Thus water solubility is very limited regardless of molecular size. Some halogenated alkanes have densities that are greater than that of water, a situation not common for organic compounds. Chloroalkanes containing two or more chlorine atoms, bromoalkanes, and iodoalkanes are all denser than water. Concepts to Remember Sign in at www.ismailpages.com to view tutorials and simulations, develop problem-solving skills, and complete online homework assigned by your professor Carbon atom bonding characteristics: Carbon atoms in organic compounds must have four bonds. Types of hydrocarbons: Hydrocarbons, binary compounds of carbon and hydrogen, are of two types: saturated and unsaturated. In saturated hydrocarbons, all carbon–carbon bonds are single bonds. Unsaturated hydrocarbons have one or more carbon–carbon multiple bonds—double bonds, triple bonds, or both. Alkanes: Alkanes are saturated hydrocarbons in which the car- bon atom arrangement is that of an unbranched or branched chain. The formulas of all alkanes can be represented by the general formula CnH2n+2, where n is the number of carbon atoms present Structural formulas: Structural formulas are two-dimensional representations of the arrangement of the atoms in molecules. These formulas give complete information about the arrangement of the atoms in a molecule but not the spatial orientation of the atoms. Two types of structural formulas are commonly encountered: expanded and condensed. Isomers: Isomers are compounds that have the same molecular formula (that is, the same numbers and kinds of atoms), but that differ in the way the atoms are arranged. Constitutional isomers: Constitutional isomers are isomers that differ in the connectivity of atoms, that is, in the order in which atoms are attached to each other within molecules. Conformations: Conformations are differing orientations of the same molecule made possible by free rotation about single bonds in the molecule. Alkane nomenclature: The IUPAC name for an alkane is based on the longest continuous chain of carbon atoms in the molecule. A group of carbon atoms attached to the chain is an alkyl group. Both the position and the identity of the alkyl group are prefixed to the name of the longest carbon chain. Line-angle structural formulas: A line-angle structural formula is a structural representation in which a line represents a carbon–carbon bond and a carbon atom is understood to be present at every point where two lines meet and at the ends of the line. Line-angle structural formulas are the most concise method for representing the structure of a hydrocarbon or hydrocarbon derivative. 31 Cycloalkanes: Cycloalkanes are saturated hydrocarbons in which at least one cyclic arrangement of carbon atoms is present. The formulas of all cycloalkanes can be represented by the general formula CnH2n, where n is the number of carbon atoms present Cycloalkane nomenclature: The IUPAC name for a cycloalkane is obtained by placing the prefix cyclo- before the alkane name that corresponds to the number of carbon atoms in the ring. Alkyl groups attached to the ring are located by using a ring- numbering system. Cis–trans isomerism: For certain disubstituted cycloalkanes, cis–trans isomers exist. Cis–trans isomers are compounds that have the same molecular and structural formulas but different arrangements of atoms in space because of restricted rotation about bonds. Natural sources of saturated hydrocarbons: Natural gas and petroleum are the largest and most important natural sources of both alkanes and cycloalkanes. Physical properties of saturated hydrocarbons: Saturated hydro- carbons are not soluble in water and have lower densities than water. Melting and boiling points increase with increasing car- bon chain length or ring size. Chemical properties of saturated hydrocarbons: Two important reactions that saturated hydrocarbons undergo are combustion and halogenation. In combustion, saturated hydrocarbons burn in air to produce CO2 and H2O. Halogenation is a substitution reaction in which one or more hydrogen atoms of the hydrocarbon are replaced by halogen atoms. Halogenated alkanes: Halogenated alkanes are hydrocarbon derivatives in which one or more halogen atoms have replaced hydrogen atoms of the alkane Halogenated alkane nomenclature: Halogenated alkanes are named by using the rules that apply to branched-chain alkanes, with halogen substituents being treated the same as alkyl groups 32 Chapter Two: Unsaturated Hydrocarbons 2.1 Unsaturated Hydrocarbons An unsaturated hydrocarbon is a hydrocarbon in which one or more carbon– carbon multiple bonds (double bonds, triple bonds, or both) are present. Unsaturated hydrocarbons are chemically more reactive than their saturated counterparts. The increased reactivity of unsaturated hydrocarbons is related to the presence of the carbon– carbon multiple bond(s) in such compounds. These multiple bonds serve as locations where chemical reactions can occur. A functional group is the part of an organic molecule where most of its chemical reactions occur. Carbon–carbon multiple bonds are the functional group for an unsaturated hydrocarbon. Types of unsaturated hydrocarbons Unsaturated hydrocarbons are subdivided into three groups on the basis of the type of multiple bond(s) present: (1) alkenes, which contain one or more carbon–carbon double bonds (2) alkynes, which contain one or more carbon– carbon triple bonds and (3) aromatic hydrocarbons, which exhibit a special type of “delocalized” bonding that involves a six-membered carbon ring. 2.2 Characteristics of Alkenes and Cycloalkenes Alkenes An alkene is an acyclic unsaturated hydrocarbon that contains one or more carbon– carbon double bonds. The alkene functional group is thus a C=C group. Note the close similarity between the family names alkene and alkane; they differ only in their endings: -ene versus -ane. The -ene ending means a double bond is present. The simplest type of alkene contains only one carbon–carbon double bond. Such compounds have the general molecular formula CnH2n. Thus alkenes with one double bond have two fewer hydrogen atoms than do alkanes (CnH2n+2,). The two simplest alkenes are ethene (C2H4) and propene (C3H6). Comparing the geometrical shape of ethene with that of methane (the simplest alkane) reveals a major difference. The arrangement of bonds about the carbon atom in methane is tetrahedral, 33 whereas the carbon atoms in ethene have a trigonal planar arrangement of bonds; that is, they form a flat, triangle- shaped arrangement. Three-dimensional representations of the structures of ethene and methane In ethene, the atoms are in a flat (planar) rather than a tetrahedral arrangement. Bond angles are 120° Cycloalkenes A cycloalkene is a cyclic unsaturated hydrocarbon that contains one or more carbon–carbon double bonds within the ring system. Cycloalkenes in which there is only one double bond have the general molecular formula CnH2n-2 The simplest cycloalkene is the compound cyclopropene (C3H4), a three- membered carbon ring system containing one double bond. Dienes and trienes Alkenes with more than one carbon–carbon double bond are relatively common. When two double bonds are present, the compounds are often called dienes; for three double bonds the designation trienes is used. Cycloalkenes that contain more than one double bond are possible but are not common. 34 2.3 IUPAC Nomenclature for Alkenes and Cycloalkenes The IUPAC rules previously presented for naming alkanes and cycloalkanes can be used, with some modification, to name alkenes and cycloalkenes. Rule 1. Replace the alkane suffix -ane with the suffix -ene, which is used to indicate the presence of a carbon–carbon double bond. Rule 2. Select as the parent carbon chain the longest continuous chain of carbon atoms that contains both carbon atoms of the double bond. For example, select Rule 3. Number the parent carbon chain beginning at the end nearest the double bond. If the double bond is equidistant from both ends of the parent chain, begin numbering from the end closer to a substituent. Rule 4. Give the position of the double bond in the chain as a single number, which is the lower- numbered carbon atom participating in the double bond. This number is placed immediately before the name of the parent carbon chain 35 Rule 5. Use the suffixes -diene, -triene, -tetrene, and so on when more than one double bond is present in the molecule. A separate number must be used to locate each double bond. Rule6. A number is not needed to locate the double bond in unsubstituted cycloalkenes with only one double bond because that bond is assumed to be between carbons 1 and 2. Rule7. In substituted cycloalkenes with only one double bond, the double-bonded carbon atoms are numbered 1 and 2 in the direction (clockwise or counter- clockwise) that gives the first- encountered substituent the lower number. Again, no number is used in the name to locate the double bond Rule8. In cycloalkenes with more than one double bond within the ring, assign one double bond the numbers 1 and 2 and the other double bonds the lowest numbers possible. 36 a. 2-hexene b. 2-ethyl-1-butene c. Methylcyclobutane d. 2-methyl-1,3-cyclopentadiene Practical Exercise Common Names (Non-IUPAC Names) The simpler members of most families of organic compounds, including alkenes, have common names in addition to IUPAC names. The two simplest alkenes, ethene and propene, have common names, They are ethylene and propylene, respectively. 37 Alkenes as Substituents Just as there are alkanes and alkyl groups, there are alkenes and alkenyl groups. An alkenyl group is a noncyclic hydrocarbon substituent in which a carbon–carbon double bond is present. The three most frequently encountered alkenyl groups are the one-, two-, and three-carbon entities, which may be named using IUPAC nomenclature (methylidene, ethenyl, and 2-propenyl) or common names (methylene, vinyl, and allyl). The use of these alkenyl group names in actual compound nomenclature is illustrated in the following examples. 2.4 Line-Angle Structural Formulas for Alkenes Line-angle formulas for the three- to six-carbon acyclic 1-alkenes are as follows. Representative line-angle structural formulas for substituent-bearing alkenes include Diene representations in terms of line-angle structural formulas include 38 2.5 Constitutional Isomerism in Alkenes Constitutional isomerism is possible for alkenes, just as it was for alkanes. In general, there are more alkene isomers for a given number of carbon atoms than there are alkane isomers. This is because there is more than one location where a double bond can be placed in systems containing four or more carbon atoms. A comparison of structural isomerism possibilities for four- and five-carbon alkane and alkene systems Positional isomers are constitutional isomers with the same carbon-chain arrangement but different hydrogen atom arrangements as the result of differing location of the functional group present. 39 Skeletal isomers are constitutional isomers that have different carbon-chain arrangements as well as different hydrogen atom arrangements. The C4 alkenes 1-butene and 2-methylpropene are skeletal isomers. All alkane isomers discussed in the previous chapter were skeletal isomers; positional isomerism is not possible for alkanes because they lack a functional group. Determining Structural Formulas for Alkene Constitutional Isomers Draw condensed structural formulas for all alkene constitutional isomers that have the molecular formula C5H10. Solution The answers for this problem have already been considered. The structures of the five C5H10 alkene constitutional isomers are given in the above figure. The purpose of this example is to consider the “thinking pattern” used to obtain the given answers. There are two concepts in the thinking pattern. 1. The different carbon skeletons (both unbranched and branched) that are possible using five carbon atoms are determined. 2. For each of the carbon skeletons determined, different positions for placement of the double bond are then considered. Step 1: There are three possible arrangements for five carbon atoms: These arrangements are the constitutional isomers for a 5-carbon alkane Step 2: For the first carbon skeleton (the unbranched chain), there are two possible locations for the double bond; that is, there are two positional isomers: Moving the double bond farther to the right than in the second structure does not produce new isomers but, rather, duplicates of the two given structures. A double bond between carbons 3 and 4 (numbering from the left side) is the same as having the double bond between carbons 2 and 3 (numbering from the right side). 40 For the second carbon skeleton, there are three positional isomers—that is, three different positions for the double bond: For the third carbon skeleton, alkene structures are not possible. Placing a double bond at any location within the structure creates a situation where the central carbon atom has five bonds. Thus there are five alkene constitutional isomers with the molecular formula C5H10. Practical exercise 2.6 Cis–Trans Isomerism in Alkenes Cis–trans isomerism is possible for some alkenes. Such isomerism results from the structural rigidity associated with carbon–carbon double bonds: Unlike the situation in alkanes, where free rotation about carbon–carbon single bonds is possible, no rotation about carbon–carbon double bonds (or carbon–carbon triple bonds) can occur. There are two requirements for cis-trans isomerism: 1. Rotation must be restricted in the molecule. 2. There must be two different groups on each doubly bonded carbon atom The simplest alkene for which cis and trans isomers exist is 2-butene. 41 Alkenes with a C=CH2 unit do not exist as cis-trans isomers. (2-propene) Alkenes with a C=CR2 unit, where the two R groups are the same, do not exist as cis-trans isomers. Alkenes of the type R–CH=CH–R can exist as cis and trans isomers Cis and Trans isomerism in substituted alkenes Determine whether each of the following substituted alkenes can exist in cis–trans isomeric forms. a. 1-Bromo-1-chloroethene b. 2-Chloro-2-butene Solution a. The condensed structural formula for this compound is Redrawing this formula to emphasize the four attachments to the double-bonded carbon atoms gives The carbon atom on the right has two identical attachments. Hence cis–trans isomerism is not possible. b. The condensed structural formula for this compound is 42 Redrawing this formula to emphasize the four attachments to the double-bonded carbon atoms gives Because both carbon atoms of the double bond bear two different attachments, cis–trans isomers are possible. Practice Exercise Determine whether each of the following substituted alkenes can exist in cis–trans isomeric forms. a. 1-Chloropropene b. 2-Chloropropene Answers: a. Yes; b. No 2.7 Physical Properties of Alkenes and Cycloalkenes The general physical properties of alkenes and cycloalkenes include insolubility in water, solubility in non-polar solvents, and densities lower than that of water. Thus they have physical properties similar to those of alkanes. The melting point of an alkene is usually lower than that of the alkane with the same number of carbon atoms. Alkenes with 2 to 4 carbon atoms are gases at room temperature. Unsubstituted alkenes with 5 to 17 carbon atoms and one double bond are liquids, and those with still more carbon atoms are solids. 43 2.8 Chemical Reactions of Alkenes Alkenes, like alkanes, are very flammable. The combustion products, as with any hydrocarbon, are carbon dioxide and water. Pure alkenes are, however, too expensive to be used as fuel. Aside from combustion, nearly all other reactions of alkenes take place at the carbon–carbon double bond(s). These reactions are called addition reactions because a substance is added to the double bond. This behavior contrasts with that of alkanes, where the most common reaction type, aside from combustion, is substitution. An addition reaction is a reaction in which atoms or groups of atoms are added to each carbon atom of a carbon–carbon multiple bonds in a hydrocarbon or hydrocarbon derivative. A general equation for an alkene addition reaction is In this reaction, the (A) part of the reactant A-B becomes attached to one carbon atom of the double bond, and the B part to the other carbon atom (As this occurs, the carbon–carbon double bond simultaneously becomes a carbon– carbon single bond. Addition reactions can be classified as symmetrical or unsymmetrical addition reaction. Symmetrical Addition Reactions A symmetrical addition reaction is an addition reaction in which identical atoms (or groups of atoms) are added to each carbon of a carbon–carbon multiple bond. The two most common examples of symmetrical addition reactions are hydrogenation and halogenation. A hydrogenation reaction is an addition reaction in which H2 is incorporated into molecules of an organic compound. In alkene hydrogenation, a hydrogen atom is added to each carbon atom of a double bond. This is accomplished by heating the alkene and H2 in the presence of a catalyst (usually Ni or Pt). 44 The hydrogenation of vegetable oils is a very important commercial process today. Vegetable oils from sources such as soybeans and cottonseeds are composed of long-chain organic molecules that contain several double bonds. When these oils are hydrogenated, they are converted to low- melting solids that are used in margarines and shortenings. A halogenation reaction is an addition reaction in which a halogen is incorporated into molecules of an organic compound. In alkene halogenation, a halogen atom is added to each carbon atom of a double bond. Chlorination (Cl2) and bromination (Br2) are the two halogenation processes most commonly encountered. No catalyst is needed. In general terms, halogenation of an alkene can be written as Bromination is often used to test for the presence of carbon–carbon double bonds in organic substances. Bromine in water or carbon tetrachloride is reddish-brown. The dibromo – compound(s) formed from the symmetrical addition of bromine to an organic compound is(are) colorless. Thus the decolorization of a Br2 solution indicates the presence of carbon–carbon double bonds 45 Unsymmetrical Addition Reactions An unsymmetrical addition reaction is an addition reaction in which different atoms (or groups of atoms) are added to the carbon atoms of a carbon–carbon multiple bond. Two important types of unsymmetrical addition reactions are hydrohalogenation and hydration. A hydrohalogenation reaction is an addition reaction in which a hydrogen halide (HCl, HBr, or HI) is incorporated into molecules of an organic compound. In alkene hydrohalogenation, one carbon atom of a double bond receives a halogen atom and the other carbon atom receives a hydrogen atom. Hydrohalogenation reactions require no catalyst. For symmetrical alkenes, such as ethene, only one product results from hydrohalogenation. A hydration reaction is an addition reaction in which H2O is incorporated into molecules of an organic compound. Alkene hydration requires a small amount of H2SO4 (sulfuric acid) as a catalyst. For symmetrical alkenes, only one product results from hydration Markovnikov’s rule states that when an unsymmetrical molecule of the form HQ adds to an unsymmetrical alkene, the hydrogen atom from the HQ becomes attached to the unsaturated carbon atom that already has the most hydrogen atoms. For example, the addition of HCl to propene (an unsymmetrical alkene) could produce either 1- chloropropane or 2-chloropropane. One product usually predominates. The dominant product can be predicted by using Markovnikov’s rule. Thus the major product in our example involving propene is 2-chloropropane. 46 Predicting Products in Alkene Addition Reactions Using Markovnikov’s Rule Using Markovnikov’s rule, predict the predominant product in each of the following addition reactions. Solution a. The predominant product of the addition will be 2-bromopentane b. Carbon 1 of the double bond does not have any H atoms directly attached to it. Carbon 2 of the double bond has one H atom (H atoms are not shown in the structure but are implied) attached to it. The H atom from the HCl will add to carbon 2, giving: 1-chloro-1-methylcyclopentane as the product c. Each carbon atom of the double bond in this molecule has one hydrogen atom. Thus Markovnikov’s rule does not favor either carbon atom. The result is two isomeric products that are formed in almost equal quantities. Practice Exercise Using Markovnikov’s rule, predict the predominant product in each of the following addition reaction. 47 In compounds that contain more than one carbon–carbon double bond, such as dienes and trienes, addition can occur at each of the double bonds. In the complete hydrogenation of a diene and in that of a triene, the amounts of hydrogen needed are twice as much and three times as much, respectively, as that needed for the hydrogenation of an alkene with one double bond. Predicting Reactants and Products in Alkene Addition Reactions Supply the structural formula of the missing substance in each of the following addition reactions. Solution a. This is a hydration reaction. Based on Markovnikov’s rule, the H will become attached to carbon 1, which has more hydrogen atoms than carbon 2, and the -OH group will be attached to carbon 2 b. The reactant alkene will have a double bond between the two carbon atoms that bromine atoms are attached to in the product. c. HBr d. Pentane 48 Practice Exercise Supply the structural formula of the missing substance in each of the following addition reactions. 2.9 Polymerization of Alkenes: Addition Polymers A polymer is a large molecule formed by the repetitive bonding together of many smaller molecules. A monomer is the small molecule that is the structural repeating unit in a polymer. A polymerization reaction is a chemical reaction in which the repetitious combining of many small molecules (monomers) produces a very large molecule (the polymer) The type of polymer that alkenes and substituted alkenes form is an addition polymer An addition polymer is a polymer in which the monomers simply “add together” with no other products formed besides the polymer. The simplest alkene addition polymer has ethylene (ethene) as the monomer. With appropriate catalysts, ethylene readily adds to itself to produce polyethylene. 49 The formula of the simplest repeating unit (the monomer with the double bond changed to a single bond) is written in parentheses and then the subscript n is added after the parentheses, with n being understood to represent a very large number. Using this notation, the formula of polyethylene becomes This notation clearly identifies the basic repeating unit found in the polymer Substituted-Ethene Addition Polymers Many substituted alkenes undergo polymerization similar to that of ethene when they are treated with the proper catalyst. For a monosubstituted-ethene monomer, the general polymerization equation is Some Common Polymers Obtained from Ethene-Based Monomers a. polyethylene b. polypropylene c. poly(vinyl chloride) (PVC) d. Teflon, and e. Polystyrene 50 Butadiene-Based Addition Polymers When dienes such as 1,3-butadiene are used as the monomers in addition polymerization reactions, the resulting polymers contain double bonds and are thus still unsaturated. In general, unsaturated polymers are much more flexible than the ethene-based saturated polymers. Natural rubber is a flexible addition polymer whose repeating unit is isoprene that is, 2- methyl-1,3-butadiene Addition Copolymers A copolymer is a polymer in which two different monomers are present. Saran Wrap is a polymer in which two different monomers are present: chloroethene (vinyl chloride) and 1,1-dichloroethene. 51 2.10 Alkynes An alkyne is an acyclic unsaturated hydrocarbon that contains one or more carbon–carbon triple bonds. The alkyne functional group is, thus, a C≡C group. As the family name alkyne indicates, the characteristic “ending” associated with a triple bond is -yne. The general formula for an alkyne with one triple bond is CnH2n−2 Thus the simplest member of this type of alkyne has the formula C2H2, and the next member, with n = 3, has the formula C3H4. The presence of a carbon–carbon triple bond in a molecule always results in a linear arrangement for the two atoms attached to the carbons of the triple bond. Thus ethyne is a linear molecule The simplest alkyne, ethyne (C2H2), is the most important alkyne from an industrial standpoint. A colorless gas, it goes by the common name acetylene and is used in oxyacetylene torches, high- temperature torches used for cutting and welding materials. IUPAC Nomenclature for Alkynes The rules for naming alkynes are identical to those used to name alkenes, except the ending -yne is used instead of -ene. Consider the following structures and their IUPAC names. Common names for simple alkynes are based on the name acetylene, as shown in the following examples. 52 Isomerism and Alkynes Because of the linearity (180 angles) about an alkyne’s triple bond, cis–trans isomerism, such as that found in alkenes, is not possible for alkynes because there are no “up” and “down” positions. However, constitutional isomers are possible—both relative to the carbon chain (skeletal isomers) and to the position of the triple bond (positional isomers). Physical and Chemical Properties of Alkynes The physical properties of alkynes are similar to those of alkenes and alkanes. In general, alkynes are insoluble in water but soluble in organic solvents, have densities less than that of water, and have boiling points that increase with molecular mass. Low-molecular-mass alkynes are gases at room temperature The triple-bond functional group of alkynes behaves chemically quite similarly to the double- bond functional group of alkenes. Thus there are many parallels between alkene chemistry and alkyne chemistry. The same substances that add to double bonds (H2, HCl, Cl2, and so on) also add to triple bonds. For example, propyne reacts with H2 to form propene first and then to form propane. 53 Alkynes, like alkenes and alkanes, are flammable; that is, they readily undergo combustion reactions. 2.11 Aromatic Hydrocarbons An aromatic hydrocarbon is an unsaturated cyclic hydrocarbon that does not readily undergo addition reactions. The bonding present in this type of compound must differ significantly from that in alkenes and alkynes. Such is indeed the case. Benzene, the simplest aromatic hydrocarbon, is the key to understanding the “special type” of bonding that is characteristic of an aromatic hydrocarbon and to specifying the identity of the aromatic hydrocarbon functional group. Benzene, a flat, symmetrical molecule with a molecular formula of C6H6 , has a structural formula that involves a six-membered carbon ring in which three double bonds are present. This structure is one of two equivalent structures that can be drawn for benzene that differ only in the locations of the double bonds (1,3,5 positions versus 2,4,6 positions): Neither of these conventional structures, however, is totally correct. the correct bonding structure for benzene is an average of the two “triene” structures. Related to this “average”-structure situation is the concept that electrons associated with the ring double bonds are not held between specific carbon atoms; instead, they are free to move “around” the carbon ring. Thus the true structure for benzene, an intermediate between that represented by the two “triene” structures, is a situation in which all carbon–carbon bonds are equivalent; they are neither single nor double bonds but something in between. Placing a double-headed arrow between the conventional 54 structures that are averaged to obtain the true structure is one way to denote the average structure. An alternative notation for denoting the bonding in benzene—a notation that involves a single structure—is In this “circle-in-the-ring” structure for benzene, the circle denotes the electrons associated with the double bonds that move “around” the ring. Each carbon atom in the ring can be considered to participate in three conventional (localized) bonds (two C-C bonds and one C-H bond) and in one delocalized bond (the circle) that involves all six carbon atoms. A delocalized bond is a covalent bond in which electrons are shared among more than two atoms. This delocalized bond is what causes benzene and its derivatives to be resistant to addition reactions, a property normally associated with unsaturation in a molecule. The structure represented by the notation is called an aromatic ring system, and it is the functional group present in aromatic compounds. An aromatic ring system is a highly unsaturated carbon ring system in which both localized and delocalized bonds are present. 2.12 Names for Aromatic Hydrocarbons Replacement of one or more of the hydrogen atoms on benzene with other groups produces benzene derivatives. Compounds with alkyl groups or halogen atoms attached to the benzene ring are commonly encountered. Benzene Derivatives with One Substituent The IUPAC system of naming monosubstituted benzene derivatives uses the name of the substituent as a prefix to the name benzene. Examples of this type of nomenclature include 55 A few monosubstituted benzenes have names wherein the substituent and the benzene ring taken together constitute a new parent name. Two important examples of such nomenclature with hydrocarbon substituents are Both of these compounds are industrially important chemicals. Monosubstituted benzene structures are often drawn with the substituent at the “12 o’clock” position, as in the previous structures. However, because all the hydrogen atoms in benzene are equivalent, it does not matter at which carbon of the ring the substituted group is located. Each of the following formulas represents chlorobenzene. For monosubstituted benzene rings that have a group attached that is not easily named as a substituent, the benzene ring is often treated as a group attached to this substituent. In this reversed approach, the benzene ring attachment is called a phenyl group, and the compound is named according to the rules for naming alkanes, alkenes, and alkynes 56 Benzene Derivatives with Two Substituents When two substituents, either the same or different, are attached to a benzene ring, three isomeric structures are possible. To distinguish among these three isomers, the positions of the substituents relative to one another must be specified. This can be done in either of two ways: by using numbers or by using nonnumerical prefixes. The prefix system uses the prefixes ortho-, meta-, and para- (abbreviated o-, m-, and p-). Ortho- means 1,2 disubstitution; the substituents are on adjacent carbon atoms. Meta- means 1,3 disubstitution; the substituents are one carbon removed from each other. Para- means 1,4 disubstitution; the substituents are two carbons removed from each other (on opposite sides of the ring). 57 When prefixes are used, the three isomeric dichlorobenzene have the second-listed set of names above. When one of the two substituents in a disubstituted benzene imparts a special name to the compound (as, for example, toluene), the compound is named as a derivative of that parent molecule. The special substituent is assumed to be at ring position 1 When neither substituent group imparts a special name, the substituents are cited in alphabetical order before the ending -benzene. The carbon of the benzene ring bearing the substituent with alphabetical priority becomes carbon A benzene ring bearing two methyl groups is a situation that generates a new special base name. Such compounds (there are three isomers) are not named as dimethylbenzenes or as methyl toluenes. They are called xylenes. The xylenes are good solvents for grease and oil and are used for cleaning microscope slides and optical lenses and for removing wax from skis. 58 Benzene Derivatives with Three or More Substituents When more than two groups are present on the benzene ring, their positions are indicated with numbers. The ring is numbered in such a way as to obtain the lowest possible numbers for the carbon atoms that have substituents. If there is a choice of numbering systems (two systems give the same lowest set), then the group that comes first alphabetically is given the lower number. Assigning IUPAC Names to Benzene Derivatives Assign IUPAC names to the following benzene derivatives. a. No substituents that will change the parent name from benzene are present on the ring. Alphabetical priority dictates that the chloro group is on carbon 1 and the ethyl group on carbon the compound is named 1-chloro-3-ethylbenzene (or m-chloroethylbenzene). b. Again, no substituents that will change the parent name from benzene are present on the ring. Alphabetical priority among substituents dictates that the bromo group is on carbon 1, the chloro group on carbon 3, and the ethyl group on carbon 5. The compound is named 1-bromo-3-chloro-5- ethylbenzene. c. This compound is named with the benzene ring treated as a substituent—that is, as a phenyl group. The compound is named 2-bromo-3-phenylbutane. The methyl group present on the benzene ring changes the parent name from benzene to toluene. 59 d. Carbon 1 bears the methyl group. Numbering clockwise, we obtain the name 2-chlorotoluene Practice Exercise Assign IUPAC names to the following benzene derivatives. Answers: a. 1-Bromo-3-ethylbenzene or m-bromoethylbenzene; b. 1-Chloro-4-propylbenzene or o-chloropropylbenzene; c. 3-Phenylhexane; d. 4-Bromo-1,2-dichlorobenzene Physical Properties and Sources of Aromatic Hydrocarbons In general, aromatic hydrocarbons resemble other hydrocarbons in physical properties. They are insoluble in water, are good solvents for other nonpolar materials, and are less dense than water. Benzene, monosubstituted benzenes, and many disubstituted benzenes are liquids at room temperature. Benzene itself is a colorless, flammable liquid that burns with a sooty flame because of incomplete combustion. At one time, coal tar was the main source of aromatic hydrocarbons. Petroleum is now the primary source of such compounds. At high temperatures, with special catalysts, saturated hydrocarbons obtained from petroleum can be converted to aromatic hydrocarbons. The production of toluene from heptane is representative of such a conversion. Benzene was once widely used as an organic solvent. Such use has been discontinued because benzene’s short- and long-term toxic effects are now recognized. Benzene inhalation can cause nausea and respiratory problems. 60 Two common situations in which a person can be exposed to low-level benzene vapors are: 1. Inhaling gasoline vapors while refueling an automobile. Gasoline contains about 2% (v/v) benzene. 2. Being around a cigarette smoker. Benzene is a combustion product present in cigarette smoke. For smokers themselves, inhaled cigarette smoke is a serious benzene exposure source. Chemical Reactions of Aromatic Hydrocarbons Aromatic hydrocarbons do not readily undergo the addition reactions. Benzene undergoes substitution reactions Substitution reactions are characterized by different atoms or groups of atoms replacing hydrogen atoms in a hydrocarbon molecule. Two important types of substitution reactions for benzene and other aromatic hydrocarbons are alkylation and halogenation. 1. Alkylation: An alkyl group (R-) from an alkyl chloride (R-Cl) substitutes for a hydrogen atom on the benzene ring. A catalyst, AlCl3, is needed for alkylation. In general terms, the alkylation of benzene can be written as Alkylation is the most important industrial reaction of benzene. 2. Halogenation (bromination or chlorination): A hydrogen atom on a benzene ring can be replaced by bromine or chlorine if benzene is treated with Br2 or Cl2 in the presence of a catalyst. The catalyst is usually FeBr3 for bromination and FeCl3 for chlorination. 61 Aromatic halogenation differs from alkane halogenation (Section 12.17) in that light is not required to initiate aromatic halogenation 2.13 Fused-Ring Aromatic Hydrocarbons Benzene and its substituted derivatives are not the only type of aromatic hydrocarbon that exists. Another large class of aromatic hydrocarbons is the fused-ring aromatic hydrocarbons. A fused-ring aromatic hydrocarbon is an aromatic hydrocarbon whose structure contains two or more carbon rings fused together. Two carbon rings that share a pair of carbon atoms are said to be fused. The three simplest fused-ring aromatic compounds are naphthalene, anthracene, and phenanthrene. All three are solids at room temperature. Concepts to Remember Sign in at www.ismailpage.com to view tutorials and simulations, develop problem-solving skills, and complete online homework assigned by your professor Unsaturated hydrocarbons: An unsaturated hydrocarbon is a hydrocarbon that contains one or more carbon–carbon multiple bonds. Three main classes of unsaturated hydrocarbons exist: alkenes, alkynes, and aromatic hydrocarbons Alkenes and cycloalkenes: An alkene is an acyclic unsaturated hydrocarbon in which one or more carbon–carbon double bonds are present. A cycloalkene is a cyclic unsaturated hydro- carbon that contains one or more carbon–carbon double bonds within the ring system Alkene nomenclature: Alkenes and cycloalkenes are given IUPAC names using rules similar to those for alkanes and cycloalkanes, except that the ending -ene is used. Also, the double bond takes precedence both in selecting and in numbering the main chain or ring 62 Isomerism in alkenes: Two subtypes of constitutional isomers are possible for alkenes: skeletal isomers and positional isomers. Positional isomers differ in the location of the functional group (double bond) present Cis–trans isomerism in alkenes:. Cis–trans isomerism is possible for some alkenes because there is restricted rotation about a carbon–carbon double bond Physical properties of alkenes: Alkenes and alkanes have similar physical properties. They are nonpolar, insoluble in water, less dense than water, and soluble in nonpolar solvents Addition reactions of alkenes: Numerous substances, including H2, Cl2, Br2, HCl, HBr, and H2O, add to an alkene carbon–carbon double bond. When both the alkene and the substance to be added are unsymmetrical, the addition proceeds according to Markovnikov’s rule: The carbon atom of the double bond that already has the greater number of H atoms gets one more Addition polymers: Addition polymers are formed from alkene monomers that undergo repeated addition reactions with each other. Many familiar and widely used materials, such as fibers and plastics, are addition polymers Alkynes and cycloalkynes: Alkynes and cycloalkynes are unsaturated hydrocarbons that contain one or more carbon–carbon triple bonds. They are named in the same way as alkenes and cycloalkenes, except that their parent names end in -yne. Like alkenes, alkynes undergo addition reactions. These occur in two steps, an alkene forming first and then an alkane. Aromatic hydrocarbons: Benzene, the simplest aromatic hydro- carbon, and other members of this family of compounds contain a six-membered ring with a cyclic, delocalized bond. This aromatic ring is often drawn as a hexagon containing a circle, which represents six electrons that move freely around the ring Nomenclature of aromatic hydrocarbons: Monosubstituted benzene compounds are named by adding the substituent name to the word benzene. Positions of substituents in disubstituted benzenes are indicated by using a numbering system or the ortho- (1,2), meta- (1,3), and para- (1,4) prefix system. Chemical reactions of aromatic hydrocarbons: Aromatic hydrocarbons undergo substitution reactions rather than addition reactions. Important substitution reactions are alkylation and halogenation 63 Chapter 3: Alcohols, Phenols, and Ethers 14 In this chapter, hydrocarbon derivatives whose functional groups contain one oxygen atom participating in two single bonds (alcohols, phenols, and ethers) are considered. Chapter 4 focuses on derivatives whose functional groups have one oxygen atom participating in a double bond (aldehydes and ketones), and Chapter 5 examines functional groups that contain two oxygen atoms, one participating in single bonds and the other in a double bond (carboxylic acids, esters, and other acid derivatives) are examined. 3.1 Bonding Characteristics of Oxygen Atoms in Organic Compounds An understanding of the bonding characteristics of the oxygen atom is a prerequisite for the study of compounds with oxygen-containing functional groups. Normal bonding behavior for oxygen atoms in such functional groups is the formation of two covalent bonds. Oxygen is a member of Group VIA of the periodic table and thus possesses six valence electrons. To complete its octet by electron sharing, an oxygen atom can form either two single bonds or a double bond. Thus, in organic chemistry, carbon forms four bonds, hydrogen forms one bond, and oxygen forms two bonds. 64 3.2 Structural Characteristics of Alcohols Alcohols are the first type of hydrocarbon derivative containing a single oxygen atom to be considered. They have the generalized formula An alcohol is an organic compound in which an−OH group is bonded to a saturated carbon atom. A saturated carbon atom is a carbon atom that is bonded to four other atoms The −OH group, the functional group that is characteristic of an alcohol, is called a hydroxyl group. A hydroxyl group is the −OH functional group. Examples of condensed structural formulas for alcohols include Alcohols may be viewed structurally as being alkyl derivatives of water in which a hydrogen atom has been replaced by an alkyl group. Alcohols may also be viewed structurally as hydroxyl derivatives of alkanes in which a hydrogen atom has been replaced by a hydroxyl group. 65 3.3 Nomenclature for Alcohols Common names exist for alcohols with simple (generally C1 through C4) alkyl groups. A common name is assigned using the following rules: Rule 1: Name all of the carbon atoms of the molecule as a single alkyl group. Rule 2: Add the word alcohol, separating the words with a space. IUPAC rules for naming alcohols that contain a single hydroxyl group follow. Rule 1: Name the longest carbon chain to which the hydroxyl group is attached. The chain name is obtained by dropping the final -e from the alkane name and adding the suffix - ol. Rule 2: Number the chain starting at the end nearest the hydroxyl group, and use the appropriate number to indicate the position of the −OH group. (In numbering of the longest carbon chain, the hydroxyl group has precedence over (“outranks”) double and triple bonds, as well as over alkyl, cycloalkyl, and halogen substituents.) Rule 3: Name and locate any other substituents present. Rule 4: In alcohols where the −OH group is attached to a carbon atom in a ring, the ring is numbered beginning with the −OH group. Numbering then proceeds in a clockwise or counterclockwise direction to give the next substituent the lower number. The number “1” (for the hydroxyl group) is omitted from the name since by definition the hydroxyl-bearing carbon is carbon 1 IUPAC and Common Names of Monohydroxy Alcohols That Contain Up to Four Carbon Atoms 66 EXAMPLE 1 Determining IUPAC Names for Alcohols Name the following alcohols, utilizing IUPAC nomenclature rules. Solution 67 a. 3-methyl-3-hexanol b. 2-ethyl-1-butanol c. 3,4-dimethylcyclo-hexanol d. 3,4-dimethyl-1-heptanol Practice Exercise Name the following alcohols utilizing IUPAC nomenclature rules. Polyhydroxy alcohols Polyhydroxy alcohols—alcohols that possess more than one hydroxyl group— can be named with only a slight modification of the preceding IUPAC rules. An alcohol in which two hydroxyl groups are present is named as a diol; one containing three hydroxyl groups is named as a triol, and so on. In these names for diols, triols, and so forth, the final -e of the parent alkane name is retained for pronunciation reasons. The first two of the preceding compounds have the common names ethylene glycol and propylene glycol. These two a