kaplan pharmacology.pdf
Document Details
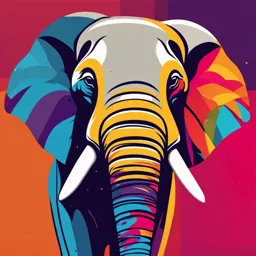
Uploaded by EquitableEiffelTower
Sanaa
2020
Tags
Related
- Pharmacology Review - NRAN 80424 Spring 2024 - PDF
- Tara Shanbhag_ Smita Shenoy - Pharmacology for Medical Graduates, 4th Updated Edition - E-Book (2020, Elsevier India) - libgen.li.pdf
- pharmacology cat 2 special.pdf
- FARMACO II (Silvia).pdf
- 26 Pharmacist.pdf
- Essentials of Medical Pharmacology PDF 8th Edition
Full Transcript
USMLE® is a joint program of the Federation of State Medical Boards (FSMB) and the National Board of Medical Examiners (NBME), which neither sponsor nor endorse this product. All rights reserved under International and Pan-American Copyright Conventions. By payment of the required fees, you have...
USMLE® is a joint program of the Federation of State Medical Boards (FSMB) and the National Board of Medical Examiners (NBME), which neither sponsor nor endorse this product. All rights reserved under International and Pan-American Copyright Conventions. By payment of the required fees, you have been granted the non-exclusive, non-transferable right to access and read the text of this eBook on screen. No part of this text may be reproduced, transmitted, downloaded, decompiled, reverse engineered, or stored in or introduced into any information storage and retrieval system, in any form or by any means, whether electronic or mechanical, now known or hereinafter invented, without the express written permission of the publisher. © 2020 by Kaplan, Inc. Published by Kaplan Medical, a division of Kaplan, Inc. 750 Third Avenue New York, NY 10017 All rights reserved. The text of this publication, or any part thereof, may not be reproduced in any manner whatsoever without written permission from the publisher. 10 9 8 7 6 5 4 3 2 1 This item comes as a set and should not be broken out and sold separately. ISBN-13: 978-1-5062-5489-0 Editors Steven R. Harris, PhD Adjunct Professor of Pharmacology and Physiology Former Associate Dean for Academic Affairs Kentucky College of Osteopathic Medicine Pikeville, KY Adjunct Professor of Pharmacology Campbell University School of Osteopathic Medicine Buies Creek, NC Contributors Laszlo Kerecsen, MD Professor of Pharmacology Midwestern University AZCOM Glendale, AZ Bimal Roy Krishna, PhD, FCP Professor and Director of Pharmacology College of Osteopathic Medicine Touro University, NV The editors would like to thank Craig Davis, PhD, for his previous contributions. We want to hear what you think. What do you like or not like about the Notes? Please email us at [email protected]. Table of Contents Part I: General Principles Chapter 1: Pharmacokinetics Chapter 2: Pharmacodynamics Chapter 3: Practice Questions Part II: Autonomic Pharmacology Chapter 1: The Autonomic Nervous System Chapter 2: Cholinergic Pharmacology Chapter 3: Adrenergic Pharmacology Chapter 4: Autonomic Drugs: Glaucoma Treatment and ANS Practice Problems Chapter 5: Autonomic Drug List and Practice Questions Part III: Cardiac and Renal Pharmacology Chapter 1: Diuretics Chapter 2: Antihypertensives Chapter 3: Drugs for Heart Failure Chapter 4: Antiarrhythmic Drugs Chapter 5: Antianginal Drugs Chapter 6: Antihyperlipidemics Chapter 7: Cardiac and Renal Drug List and Practice Questions Part IV: CNS Pharmacology Chapter 1: Sedative-Hypnotic-Anxiolytic Drugs Chapter 2: Alcohols Chapter 3: Drugs Used for Depression, Bipolar Disorders, and Attention Deficit Hyperactivity Disorder (ADHD) Chapter 4: Drugs Used in Parkinson Disease and Psychosis Chapter 5: Anticonvulsants Chapter 6: Drugs Used in Anesthesia Chapter 7: Opioid Analgesics Chapter 8: Drugs of Abuse Chapter 9: CNS Drug List and Practice Questions Part V: Antimicrobial Agents Chapter 1: Antibacterial Agents Chapter 2: Antifungal Agents Chapter 3: Antiviral Agents Chapter 4: Antiprotozoal Agents Chapter 5: Antimicrobial Drug List and Practice Questions Part VI: Drugs for Inflammatory and Related Disorders Chapter 1: Histamine and Antihistamines Chapter 2: Drugs Used in Gastrointestinal Dysfunction Chapter 3: Drugs Acting on Serotonergic Systems Chapter 4: Eicosanoid Pharmacology Chapter 5: Drugs Used for Treatment of Rheumatoid Arthritis Chapter 6: Drugs Used for Treatment of Gout Chapter 7: Glucocorticoids Chapter 8: Drugs Used for Treatment of Asthma Chapter 9: Inflammatory Disorder Drug List and Practice Questions Part VII: Drugs Used in Blood Disorders Chapter 1: Anticoagulants Chapter 2: Thrombolytics Chapter 3: Antiplatelet Drugs Chapter 4: Blood Disorder Drug List and Practice Questions Part VIII: Endocrine Pharmacology Chapter 1: Drugs Used in Diabetes Chapter 2: Steroid Hormones Chapter 3: Antithyroid Agents Chapter 4: Drugs Related to Hypothalamic and Pituitary Hormones Chapter 5: Drugs Used for Bone and Mineral Disorders Chapter 6: Endocrine Drug List and Practice Questions Part IX: Anticancer Drugs, Immunopharmacology, and Toxicology Chapter 1: Anticancer Drugs Chapter 2: Immunopharmacology Chapter 3: Toxicology Chapter 4: Anticancer Drugs, Immunopharmacology, and Toxicology Practice Questions Additional resources available at www.kaptest.com/usmlebookresources PART I General Principles Pharmacokinetics 1 Learning Objectives Answer questions about permeation, absorption, distribution, biotransformation, elimination, and steady state Solve problems concerning important pharmacokinetics calculations PHARMACOKINETICS Pharmacokinetic characteristics of drug molecules concern the processes of absorption, distribution, metabolism, and excretion. The biodisposition of a drug involves its permeation across cellular membrane barriers. Figure I-1-1. Drug Biodisposition Permeation Drug permeation is dependent on the following: Solubility. The ability to diffuse through lipid bilayers (lipid solubility) is important for most drugs; however, water solubility can influence permeation through aqueous phases. Concentration gradient. Diffusion down a concentration gradient—only free, unionized drug forms contribute to the concentration gradient. Surface area and vascularity. Important with regard to absorption of drugs into the systemic circulation. The larger the surface area and the greater the vascularity, the better is the absorption of the drug. Ionization Many drugs are weak acids or weak bases, and can exist in either nonionized or ionized forms in an equilibrium, depending on the pH of the environment and the pKa (the pH at which the molecule is 50% ionized and 50% nonionized). Only the nonionized (uncharged) form of a drug crosses biomembranes. The ionized form is better renally excreted because it is water soluble. Note For Weak Acids and Weak Bases Ionized = water soluble Nonionized = lipid soluble Figure I-1-2. Degree of Ionization and Clearance Versus pH Deviation from pKa Clinical Correlate Gut bacteria metabolize lactulose to lactic acid, acidifying the fecal masses and causing ammonia to become ammonium. Therefore, lactulose is useful in hepatic encephalopathy. Ionization Increases Renal Clearance of Drugs Only free, unbound drug is filtered. Both ionized and nonionized forms of a drug are filtered. Only nonionized forms undergo active secretion and active or passive reabsorption. Ionized forms of drugs are “trapped” in the filtrate. Acidification of urine → increases ionization of weak bases → increases renal elimination. Alkalinization of urine → increases ionization of weak acids → increases renal elimination. Clinical Correlate To Change Urinary pH Acidify: NH4Cl, vitamin C, cranberry juice Alkalinize: NaHCO3, acetaz olamide (historically) See Aspirin Overdose and Management in Section VI. Figure I-1-3. Renal Clearance of Drug Modes of Drug Transport Across a Membrane Table I-1-1. The 3 Basic Modes of Drug Transport Across a Membrane Energy Mechanism Direction Carrier Saturable Required Passive diffusion Down gradient No No No Facilitated Down gradient No Yes Yes diffusion Active transport Against gradient Yes Yes Yes (concentration/electrical) Bridge to Physiology Ion and molecular transport mechanisms are discussed in greater detail in Part I of Physiology. ABSORPTION Absorption concerns the processes of entry of a drug into the systemic circulation from the site of its administration. The determinants of absorption are those described for drug permeation. Intravascular administration (e.g., IV) does not involve absorption, and there is no loss of drug. Bioavailability = 100% With extravascular administration (e.g., per os [PO; oral], intramuscular [IM], subcutaneous [SC], inhalation), less than 100% of a dose may reach the systemic circulation because of variations in bioavailability. Plasma Level Curves Cmax= maximal drug level obtained with the dose. tmax = time at which Cmax occurs. Lag time = time from administration to appearance in blood. Onset of activity = time from administration to blood level reaching minimal effective concentration (MEC). Duration of action = time plasma concentration remains greater than MEC. Time to peak = time from administration to Cmax. Figure I-1-4. Plot of Plasma Concentration Versus Time Bioavailability (f) Bioavailability is the measure of the fraction of a dose that reaches the systemic circulation. By definition, intravascular doses have 100% bioavailability, f = 1. AUC: area under the curve PO: oral IV: intravenous bolus AUCIV: horizontally striped area AUCPO: vertically striped area Figure I-1-5. Area Under the Curve for an IV Bolus and Extravascular Doses First-Pass Effect With oral administration, drugs are absorbed into the portal circulation and initially distributed to the liver. For some drugs, their rapid hepatic metabolism decreases bioavailability, i.e., the “first-pass” effect. Clinical Correlate Lidocaine is given IV and nitroglycerin is given sublingually to avoid extensive first-pass metabolism. Figure I-1-6. Bioavailability and First-Pass Metabolism DISTRIBUTION Distribution is the process of distribution of a drug from the systemic circulation to organs and tissue. Under normal conditions, protein-binding capacity is much larger than is drug concentration. Consequently, the free fraction is generally constant. Many drugs bind to plasma proteins, including albumin, with an equilibrium between bound and free molecules (recall that only unbound drugs cross biomembranes). Drug + Protein ⇌ Drug-Protein Complex (Active, free) (Inactive, bound) Competition between drugs for plasma protein-binding sites may increase the “free fraction,” possibly enhancing the effects of the drug displaced. Example: sulfonamides and bilirubin in a neonate Clinical Correlate Drugs with high plasma protein binding and narrow therapeutic range (e.g., warfarin, phenytoin) are prone to drug interactions. Special Barriers to Distribution There are some special barriers to distribution: Placental: most small molecular weight drugs cross the placental barrier, although fetal blood levels are usually lower than maternal (e.g., propylthiouracil [PTU] versus methimazole in pregnancy) Blood–brain: permeable only to lipid-soluble drugs or those which are transported by facilitated diffusion or active transport.” (e.g., levodopa versus dopamine) Apparent Volume of Distribution Apparent volume of distribution (Vd) is a kinetic parameter of a drug which correlates dose with plasma level at zero time. This relationship can be used for calculating Vd by using the dose only if one knows C0. Vd is low when a high percentage of a drug is bound to plasma proteins. Vd is high when a high percentage of a drug is being sequestered in tissues. This raises the possibility of displacement by other agents; examples: verapamil and quinidine can displace digoxin from tissue-binding sites. Vd is needed to calculate a loading dose in the clinical setting (see Pharmacokinetic Calculation section, Equation 4). Bridge to Physiology Approximate Vd Values (weight 70 kg) Plasma volume (3 L) Blood volume (5 L) Extracellular fluid (ECF 12–14 L) Total body water (TBW 40–42 L) Redistribution In addition to crossing the blood–brain barrier (BBB), lipid-soluble drugs redistribute into fat tissues prior to elimination. In the case of CNS drugs, the duration of action of an initial dose may depend more on the redistribution rate than on the half-life. With a second dose, the blood/fat ratio is less; therefore, the rate of redistribution is less and the second dose has a longer duration of action. Figure I-1-7. Redistribution BIOTRANSFORMATION The general principle of biotransformation is the metabolic conversion of drug molecules to more water-soluble metabolites that are more readily excreted. In many cases, metabolism of a drug results in its conversion to compounds that have little or no pharmacologic activity. In other cases, biotransformation of an active compound may lead to the formation of metabolites that also have pharmacologic actions. A few compounds (prodrugs) have no activity until they undergo metabolic activation. Some compounds are converted to toxic metabolites, e.g., acetaminophen. Figure I-1-8. Biotransformation of Drugs Clinical Correlate Active Metabolites Biotransformation of the benzodiazepine diazepam results in formation of nordiazepam, a metabolite with sedative-hypnotic activity and a long duration of action. Biotransformation Classification There are two broad types of biotransformation, phase I and phase II. Clinical Correlate Active components in grapefruit juice include furanocoumarins capable of inhibiting the metabolism of many drugs, including alprazolam, midazolam, atorvastatin, and cyclosporine. Such compounds may also enhance oral bioavailability decreasing first-pass metabolism and by inhibiting drug transporters in the GI tract responsible for intestinal efflux of drugs. Phase I Phase I biotransformation is modification of the drug molecule via oxidation, reduction, or hydrolysis. Microsomal metabolism ‒ Cytochrome P450 isozymes: major enzyme systems involved in phase I reactions; localized in smooth endoplasmic reticulum (microsomal fraction) of cells (especially liver but also GI tract, lungs, kidney) ‒ P450s have an absolute requirement for molecular oxygen and NADPH ‒ Oxidations include hydroxylations and dealkylations ‒ Multiple CYP families differing by amino acid (AA) composition, by substrate specificity, and by sensitivity to inhibitors and to inducing agents Table I-1-2. Cytochrome P450 Isozymes Genetic CYP450 Substrate Example Inducers Inhibitors Polymorphisms 1A2 Theophylline Aromatic hydrocarbons Quinolones No Acetaminophen (smoke) Macrolides Cruciferous vegetables 2C9 Phenytoin General inducers* — Yes Warfarin 2D6 Many cardiovascular and None known Haloperidol Yes CNS drugs Quinidine SSRIs 3A4 60% of drugs in PDR General inducers* General No inhibitors † Grapefruit juice * General inducers: anticonvulsants (barbiturates, phenytoin, carbamazepine), antibiotics (rifampin), chronic alcohol, St. John’s Wort. † General inhibitors: antiulcer medications (cimetidine, omeprazole), antimicrobials (chloramphenicol, macrolides, ritonavir, ketoconazole), acute alcohol. Nonmicrosomal metabolism ‒ Hydrolysis: phase I reaction involving addition of a water molecule with subsequent bond breakage; includes esterases and amidases ‒ Genetic polymorphism exists with pseudocholinesterases; examples include local anesthetics and succinylcholine ‒ Monoamine oxidases: metabolism of endogenous amine neurotransmitters (dopamine, norepinephrine, and serotonin); metabolism of exogenous compounds (tyramine) ‒ Alcohol metabolism: alcohols are metabolized to aldehydes and then to acids by dehydrogenases (see CNS Pharmacology, part IV); genetic polymorphisms exist Phase II Phase II biotransformation is conjugation with endogenous compounds via the activity of transferases. It may follow phase I or occur directly. Types of conjugation include: Glucuronidation ‒ Inducible; may undergo enterohepatic cycling (drug: glucuronide → intestinal bacterial glucuronidases → free drug) ‒ Reduced activity in neonates, chloramphenicol and gray baby syndrome ‒ Morphine is activated to morphine-6 glucuronide Acetylation ‒ Genotypic variations (fast and slow metabolizers) ‒ Drug-induced SLE by slow acetylators with hydralazine > procainamide > isoniazid (INH) Glutathione (GSH) conjugation ‒ Depletion of GSH in liver is associated with acetaminophen hepatotoxicity Recall Question Which of the following routes of administration has the highest bioavailability? A. Intramuscular B. Intravascular C. Oral D. Subcutaneous E. Sublingual Answer: B ELIMINATION Clinical Correlate Elimination of a drug from the body does not always end the therapeutic effect. Irreversible inhibitors, e.g. aspirin, PPIs, MAOIs, have a therapeutic effect long after the drug is eliminated. Elimination concerns the processes involved in the elimination of drugs from the body (and/or plasma) and their kinetic characteristics. The major modes of drug elimination are: Biotransformation to inactive metabolites Excretion via the kidney Excretion via other modes, including the bile duct, lungs, and sweat The time to eliminate 50% of a given amount (or to decrease plasma level to 50% of a former level) is called the elimination half-life (t1/2). Zero-Order Elimination Rate With zero-order elimination rate, a constant amount of drug is eliminated per unit time. If 80 mg is administered and 10 mg is eliminated every 4 h, the time course of drug elimination is: The rate of elimination is independent of plasma concentration (or amount in the body). Drugs with zero-order elimination have no fixed half-life (t1/2 is a variable) Drugs with zero-order elimination include ethanol (except low blood levels), phenytoin (high therapeutic doses), and salicylates (toxic doses) Figure I-1-9a. Plots of Zero-Order Kinetics First-Order Elimination Rate With first-order elimination rate, a constant fraction of the drug is eliminated per unit time (t1/2 is a constant). Graphically, first-order elimination follows an exponential decay versus time. If 80 mg of a drug is administered and its elimination half-life = 4 h, the time course of its elimination is: The rate of elimination is directly proportional to plasma level (or the amount present), i.e., the higher the amount, the more rapid the elimination. Most drugs follow first-order elimination rates t1/2 is a constant Figure I-1-9b. Plots of First-Order Kinetics Note Elimination Kinetics Most drugs follow first order: rate falls as plasma level falls. Zero order is due to saturation of elimination mechanisms; e.g., drug-metabolizing reactions have reached Vmax. Zero order-elimination rate is constant; t1/2 is a variable. First order-elimination rate is variable; t1/2 is a constant. Graphic Analysis Example of a graphic analysis of t1/2: Figure I-1-10. Plasma Decay Curve—First-Order Elimination The figure shows a plasma decay curve of a drug with first-order elimination plotted on semilog graph paper. The elimination half-life (t1/2) and theoretical plasma concentration at zero time (C0) can be estimated from the graphic relationship between plasma concentrations and time. C0 is estimated by extrapolation of the linear plasma decay curve to intercept with the vertical axis. Renal Elimination The rate of elimination is the glomerular filtration rate (GFR) + active secretion – reabsorption (active or passive). Bridge to Renal Physiology Inulin clearance is used to estimate GFR because it is not reabsorbed or secreted. A normal GFR is close to 120 mL/min. Filtration is a nonsaturable linear function. Ionized and nonionized forms of drugs are filtered, but protein-bound drug molecules are not. Clearance (Cl) is the volume of blood cleared of drug per unit of time ‒ Cl is constant in first-order kinetics ‒ Cl = GFR when there is no reabsorption or secretion and no plasma protein binding ‒ Protein-bound drug is not cleared; Cl = free fraction × GFR STEADY STATE Steady state is reached either when rate in = rate out or when values associated with a dosing interval are the same as those in the succeeding interval. Plateau Principle The time to reach steady state is dependent only on the elimination half-life of a drug. It is independent of dose size and frequency of administration, assuming the drug is eliminated by first-order kinetics. The figure below shows plasma levels (solid lines) achieved following the IV bolus administration of 100 units of a drug at intervals equivalent to every half-life t1/2 = 4 h (τ). With such intermittent dosing, plasma levels oscillate through peaks and troughs, with averages shown in the diagram by the dashed line. Figure I-1-11. Oscillations in Plasma Levels following IV Bolus Administration at Intervals Equal to Drug Half-Life Note: Although it takes >7 t1/2 to reach mathematical steady state, by convention clinical steady state is accepted to be reached at 4–5 t1/2. Note Classic Clues Time and Steady State 50% = 1 × half-life 90% = 3.3 × half-life 95% = 4–5 × half-life “100”% = >7 × half-life Rate of Infusion The figure below shows the increase in plasma level of the same drug infused at five rates. Regardless of the rate of infusion, it takes the same amount of time to reach steady state. Figure I-1-12. Effect of Rate of Infusion on Plasma Rate of infusion (k0) does determine plasma level at steady state. If the rate of infusion is doubled, then the plasma level of the drug at steady state is doubled. A similar relationship can exist for other forms of drug administration (e.g., per oral)—doubling oral doses can double the average plasma levels of a drug. Plotting dose against plasma concentration yields a straight line (linear kinetics). Note Plasma concentration (CSS) is directly proportional to the dose and inversely proportional to the clearance. Effect of Loading Dose It takes 4–5 half-lives to achieve steady state. In some situations, a higher dose (loading dose) may be needed to more rapidly achieve effective blood levels (Cp). Figure I-1-13. Effect of a Loading Dose on the Time Required to Achieve the Minimal Effective Plasma Concentration Such loading doses are often one time only and are estimated to put into the body the amount of drug that should be there at a steady state. Note The loading dose equation can be used to calculate the amount of drug in the body at any time by knowing the Vd and plasma concentration. Note For the exam, if doses are to be administered at each half-life of the drug, and minimum effective concentration is equivalent to CSSmin, then the loading dose is twice the amount of the dose used for maintenance (assuming normal clearance and same bioavailability for maintenance doses). For any other interval of dosing, use the equation listed in the Note above. PHARMACOKINETICS CALCULATIONS The following relationships are important for pharmacokinetic calculations: C0 = conc. at time zero Cl = clearance Cp = conc. in plasma Css = steady state conc. D = dose f = bioavailability τ = dosing interval Single-Dose Equations Multiple Dose or (Infusion Rate) Equations Pharmacodynamics 2 Learning Objectives Differentiate between graded (quantitative) dose-response (D-R), and quantal (cumulative) D-R curves Use knowledge of signaling mechanisms Demonstrate understanding of drug development and testing DEFINITIONS Bridge to Biochemistry Affinity is how well a drug and a receptor recognize each other. Inversely related to Kd of the drug Notice analogy to Km value used in enzyme kinetic studies Potency is the quantity of drug required to achieve a desired effect. In D-R measurements, the chosen effect is usually 50% of maximal effect but clinically any size response can be sought. Efficacy is the maximal effect an agonist can achieve at the highest practical concentration. Notice analogy to Vmax used in enzyme kinetic studies Pharmacodynamics relates to drugs binding to receptors and their effects. A drug is called an agonist when binding to the receptor results in a response. A drug is called an antagonist when binding to the receptor is not associated with a response; the drug has an effect only by preventing an agonist from binding to the receptor. Affinity is the ability of a drug to bind to receptor, shown by the proximity of the curve to the y axis (if the curves are parallel); the nearer the y axis, the greater the affinity. Potency shows relative doses of ≥2 agonists to produce the same magnitude of effect, again shown by the proximity of the respective curves to the y axis (if the curves do not cross). Efficacy is a measure of how well a drug produces a response (effectiveness), shown by the maximal height reached by the curve. GRADED (QUANTITATIVE) DOSE-RESPONSE (D-R) CURVES Plots of dose (or log dose) versus response for drugs (agonists) that activate receptors can reveal information about affinity, potency, and efficacy of these agonists. Parallel and Nonparallel D-R Curves Figure I-2-1. D-R Curves for 2 Drugs Acting on Same (left) and Different (right) Receptors It may be seen from the log dose-response curves above that: When 2 drugs interact with the same receptor (same pharmacologic mechanism), the D-R curves will have parallel slopes. Drugs A and B have the same mechanism; drugs X and Y do not. Affinity can be compared only when 2 drugs bind to the same receptor. Drug A has a greater affinity than drug B. In terms of potency, drug A has greater potency than drug B, and X is more potent than Y. In terms of efficacy, drugs A and B are equivalent. Drug X has greater efficacy than drug Y. Full and Partial Agonists Full agonists produce a maximal response, i.e., they have maximal efficacy. Partial agonists are less effective, i.e., they are incapable of eliciting a maximal response. In the figure below, drug B is a full agonist while drugs A and C are partial agonists. Figure I-2-2. Efficacy and Potency of Full and Partial Agonists Drug A is more potent than drug C, and drug B is more potent than drug C. However, no general comparisons re potency can be made between drugs A and B because the former is a partial agonist and the latter is a full agonist. At low responses, A is more potent than B, but at high responses, the reverse is true. Clinical Correlate Important drugs which are partial agonists include acebutolol, pindolol, buspirone, aripiprazole, buprenorphine, clomiphene, tamoxifen, and raloxifene. Duality of Partial Agonists In the figure below, the lower curve represents effects of a partial agonist when used alone; its ceiling effect is 50% of maximal in this example. Figure I-2-3. Duality of Partial Agonists The upper curve shows the effect of increasing doses of the partial agonist on the maximal response (100%) achieved in the presence of or by pretreatment with a full agonist. As the partial agonist displaces the full agonist from the receptor, the response is reduced—the partial agonist is acting as an antagonist. Antagonism and Potentiation Graded dose-response curves also provide information about antagonists (drugs that interact with receptors to interfere with their activation by agonists). Figure I-2-4. D-R Curves of Antagonists and Potentiators Bridge to Biochemistry Parallels between Receptor Antagonists and Enzyme Inhibitors Competitive antagonists are analogous to competitive inhibitors; they decrease affinity (↑ Km ) but not maximal response (Vmax remains the same). Noncompetitive antagonists decrease Vmax but do not change the Km. Pharmacologic antagonism (same receptor) ‒ Competitive antagonists (cause parallel shift to the right in D-R curve for agonists) Can be reversed by increasing dose of agonist drug Appear to decrease potency of agonist ‒ Noncompetitive antagonists (cause nonparallel shift to the right) Can be only partially reversed by increasing dose of agonist Appear to decrease efficacy of the agonist Physiologic antagonism (different receptor) ‒ Two agonists with opposing action antagonize each other ‒ Example: a vasoconstrictor with a vasodilator Chemical antagonism: ‒ Formation of a complex between effector drug and another compound ‒ Example: protamine binds to heparin to reverse its actions Potentiation ‒ Causes a parallel shift to the left to the D-R curve ‒ Appears to increase potency of agonist QUANTAL (CUMULATIVE) D-R CURVES Quantal D-R curves plot the percentage of a population responding to a specified drug effect versus dose or log dose. They permit estimations of the median effective dose, or effective dose in 50% of a population—ED50. Can reveal the range of intersubject variability in drug response Steep D-R curve reflects little variability Flat D-R curve indicates great variability in patient sensitivity to the effects of a drug Toxicity and Therapeutic Index Comparisons between ED50 and TD50 values permit evaluation of the relative safety of a drug (the therapeutic index, or TI), as would comparison between ED50 and the lethal median dose (LD50) if the latter is known. Figure I-2-5. Quantal D-R Curves of Therapeutic and Toxic Effects of a Drug D-R curves can also show the relationship between dose and toxic effects of a drug. The median toxic dose of a drug (TD50) is the dose that causes toxicity in 50% of a population. From the data above, TI = 10/2 = 5. Such indices are of most value when toxicity represents an extension of the pharmacologic actions of a drug. They do not predict idiosyncratic reactions or drug hypersensitivity. Recall Question Which of the following best describes the effect of a competitive antagonist on the dose-response curve? A. Non-parallel left shift B. Non-parallel right shift C. Parallel left shift D. Parallel right shift Answer: D SIGNALING MECHANISMS The binding of an agonist drug to its receptor activates an effector or signaling mechanism. Several types of drug-responsive signaling mechanisms are known. Intracellular Receptors Intracellular receptors include receptors for steroids. Binding of hormones or drugs to such receptors releases regulatory proteins which permit activation (and in some cases, dimerization) of the hormone-receptor complex. Such complexes translocate to the nucleus, where they interact with response elements in spacer DNA. This interaction leads to changes in gene expression. For example, drugs interacting with glucocorticoid receptors lead to gene expression of proteins that inhibit the production of inflammatory mediators. Other examples of intracellular receptors include intracellular receptors for thyroid hormones, gonadal steroids, and vitamin D. Pharmacologic responses elicited via modification of gene expression are usually slower in onset but longer in duration than many other drugs. Membrane Receptors Directly Coupled to Ion Channels Many drugs act by mimicking or antagonizing the actions of endogenous ligands that regulate flow of ions through excitable membranes via their activation of receptors that are directly coupled (no second messengers) to ion channels. For example, the nicotinic receptor for ACh (present in autonomic nervous system [ANS] ganglia, the skeletal myoneural junction, and the central nervous system [CNS]) is coupled to a Na+/K+ ion channel. The receptor is a target for many drugs, including nicotine, choline esters, ganglion blockers, and skeletal muscle relaxants. Similarly, the GABAA receptor in the CNS, which is coupled to a chloride ion channel, can be modulated by anticonvulsants, benzodiazepines, and barbiturates. Receptors Linked Via Coupling Proteins to Intracellular Effectors Many receptor systems are coupled via GTP-binding proteins (G proteins) to adenyl cyclase, the enzyme that converts ATP to cAMP, a second messenger which promotes protein phosphorylation by activating protein kinase A. These receptors are typically “serpentine,” with 7 transmembrane spanning domains, a third of which is coupled to the G-protein effector mechanism. Protein kinase A serves to phosphorylate a set of tissue-specific substrate enzymes or transcription factors (CREB), thereby affecting their activity. Note Key ANS Receptors M1, M3, α1: Gq activation of phospholipase C M2, α2, D2: Gi inhibition of adenylyl cyclase β1, β2, D1: Gs activation of adenylyl cyclase Gs proteins Binding of agonists to receptors linked to Gs proteins increases cAMP production. Such receptors include those for catecholamines (beta), dopamine (D1), glucagon, histamine (H2), prostacyclin, and some serotonin subtypes. Gi proteins Binding of agonists to receptors linked to Gi proteins decreases cAMP production. Such receptors include adrenoreceptors (alpha2), ACh (M2), dopamine (D2 subtypes), and several opioid and serotonin subtypes. Gq proteins Other receptor systems are coupled via GTP-binding proteins (Gq), which activate phospholipase C. Activation of this enzyme releases the second messengers inositol triphosphate (IP3) and diacylglycerol (DAG) from the membrane phospholipid phosphatidylinositol bisphosphate (PIP2). The IP3 induces release of Ca2+ from the sarcoplasmic reticulum (SR), which, together with DAG, activates protein kinase C. The protein kinase C serves then to phosphorylate a set of tissue-specific substrate enzymes, usually not phosphorylated by protein kinase A, and thereby affects their activity. These signaling mechanisms are invoked following activation of receptors for ACh (M1 and M3), norepinephrine (alpha1), angiotensin II, and several serotonin subtypes. Bridge to Biochemistry See Chapter 9 of the Biochemistry Lecture Notes for additional discussion of signal transduction. Figure I-2-6. Receptors Using Cyclic AMP and IP3 , DAG, Ca2+ as Second Messengers Cyclic GMP and Nitric Oxide Signaling Cyclic GMP (cGMP) is a second messenger in vascular smooth muscle that facilitates dephosphorylation of myosin light chains, preventing their interaction with actin and thus causing vasodilation. Nitric oxide (NO) is synthesized in endothelial cells and diffuses into smooth muscle. NO activates guanylyl cyclase, thus increasing cGMP in smooth muscle. Vasodilators ↑ synthesis of NO by endothelial cells. Clinical Correlate Drugs acting via NO include nitrates (e.g., nitroglycerin) and M-receptor agonists (e.g., bethanechol). Endogenous compounds acting via NO include bradykinin and histamine. Receptors That Function as Enzymes or Transporters There are multiple examples of drug action which depends on enzyme inhibition, including inhibitors of acetylcholinesterase, angiotensin-converting enzyme, aspartate protease, carbonic anhydrase, cyclooxygenases, dihydrofolate reductase, DNA/RNA polymerases, monoamine oxidases, Na/K-ATPase, neuraminidase, and reverse transcriptase. Examples of drug action on transporter systems include the inhibitors of reuptake of several neurotransmitters, including dopamine, GABA, norepinephrine, and serotonin. Receptors That Function as Transmembrane Enzymes These receptors mediate the first steps in signaling by insulin and growth factors, including epidermal growth factor (EGF) and platelet-derived growth factor (PDGF). They are membrane-spanning macro-molecules with recognition sites for the binding of insulin and growth factors located externally and a cytoplasmic domain that usually functions as a tyrosine kinase. Binding of the ligand causes conformational changes (e.g., dimerization) so that the tyrosine kinase domains become activated, ultimately leading to phosphorylation of tissue-specific substrate proteins. Guanyl cyclase−associated receptors: stimulation of receptors to atrial natriuretic peptide activates the guanyl cyclase and ↑ cyclic GMP (cGMP) Clinical Correlate Imatinib is a specific tyrosine-kinase (TK) inhibitor, while sorafenib is a non-specific TK inhibitor. Receptors for Cytokines Receptors for cytokines include the receptors for erythropoietin, somatotropin, and interferons. Their receptors are membrane spanning, and on activation, can activate a distinctive set of cytoplasmic tyrosine kinases (Janus kinases [JAKs]). JAKs phosphorylate signal transducers and activators of transcription (STAT) molecules. STATs dimerize and then dissociate, cross the nuclear membrane, and modulate gene transcription. Practice Questions 3 PRACTICE QUESTIONS 1. A patient was given a 200 mg dose of a drug IV, and 100 mg was eliminated during the first 2 hours. If the drug follows first-order elimination kinetics, how much of the drug will remain 6 hours after its administration? A. None B. 25 mg C. 50 mg D. 75 mg E. 100 mg 2. Drugs that are administered IV are A. Rapidly absorbed B. Subject to first-pass metabolism C. 100% bioavailable D. Rapidly excreted by the kidneys E. Rapidly metabolized by the liver 3. Drugs that are highly bound to albumin: A. Effectively cross the BBB B. Are easily filtered at the glomerulus C. Have a large Vd D. Often contain quaternary nitrogens E. Can undergo competition with other drugs for albumin binding sites 4. Most drugs gain entry to cells by: A. Passive diffusion with zero-order kinetics B. Passive diffusion with first-order kinetics C. Active transport with zero-order kinetics D. Active transport with first-order kinetics E. Passive diffusion through membrane pores 5. A subject in whom the renal clearance of inulin is 120 mL/min is given a drug, the clearance of which is found to be 18 mL/min. If the drug is 40% plasma protein bound, how much filtered drug must be reabsorbed in the renal tubules? A. None B. 18 mL/min C. 36 mL/min D. 54 mL/min E. 72 mL/min 6. If a drug is known to be distributed into total body water, what dose (mg) is needed to obtain an initial plasma level of 5 mg/L in a patient weighing 70 kg? A. 210 B. 150 C. 110 D. 50 E. 35 7. Which of the following is a phase II drug metabolism reaction associated with a genetic polymorphism? A. Acetylation B. Glucuronidation C. Oxidation D. Reduction E. Glutathione conjugation 8. A woman is taking oral contraceptives (OCs). Which of the following drugs is unlikely to reduce the effectiveness of the OCs? A. Carbamazepine B. Phenytoin C. Ketoconazole D. Phenobarbital E. Rifampin 9. The data presented in the figure below show that: A. Drugs A and B have equal efficacy B. Drug B and C have equal efficacy C. Drug B is a partial agonist D. Drugs A and C have the same affinity and efficacy E. Drugs A and B have equal potency 10. A 500-mg dose of a drug has therapeutic efficacy for 6 h. If the half-life of the drug is 8 h, for how long would a 1-g dose be effective? A. 8 h B. 12 h C. 14 h D. 16 h E. 24 h 11. Which statement is accurate for the drug shown in the example below? 100 mg 2hr → 50 mg 2hr → 25 mg 2hr → 12.5 mg A. The rate of elimination is constant B. The elimination half-life varies with the dose C. The volume of distribution varies with the dose D. The clearance varies with the dose E. The rate of elimination varies directly with the dose 12. Normally, gentamicin has a Vd= 20L and C1 = 80 mL/min. If gentamicin was administered to a patient with 50% renal function, what parameter would differ from normal? A. Loading dose would be higher B. Maintenance dose would be lower C. t ½ would be shorter D. Vd would be 35L E. Cl would be 700 mL/min 13. Pharmacokinetic characteristics of propranolol include Vd = 300 L/70 kg, C1 = 700 mL/min, and oral bioavailability f = 0.25. What is the dose needed to achieve a plasma level equivalent to a steady-state level of 20 μg/L? A. 4 mg B. 8 mg C. 12 mg D. 24 mg E. 48 mg 14. With IV infusion, a drug reaches 50% of its final steady state in 6 hours. The elimination half-life of the drug must be approximately: A. 2 h B. 6 h C. 12 h D. 24 h E. 30 h 15. At 6 h after IV administration of bolus dose, the plasma level of a drug is 5 mg/L. If the Vd = 10 L and the elimination half-life = 3 h, what was the dose administered? A. 100 mg B. 150 mg C. 180 mg D. 200 mg E. 540 mg 16. An IV infusion of a drug is started 400 mg/h. If C1 = 50 L/h, what is the anticipated plasma level at steady state? A. 2 mg/L B. 4 mg/L C. 8 mg/L D. 16 mg/L E. 32 mg/L ANSWERS AND EXPLANATIONS 1. Answer: B. One half of the dose is eliminated in the first 2 hours so its elimination half-life equals 2 hours. With the passage of each half-life the amount in the body (or in the blood) will decrease to 50% of a former level. Thus, at 6 hours after administration, 3 half-lives have passed: (1) 200 mg to 100 mg, (2) 100 mg to 50 mg, and (3) 50 mg to 25 mg. 2. Answer: C. By definition, IV administration does not involve absorption because there is no movement from the site of administration into the blood. The IV route avoids first-pass metabolism which is common with orally administered drugs. First-pass greatly reduces the bioavailability of many drugs. Drugs given IV have 100% bioavailability (f = 1) since the entire dose is in the systemic circulation. No conclusions can be drawn about renal or hepatic elimination of a drug knowing only that it was administered IV. 3. Answer: E. Since most drugs are lipid-soluble they will need a carrier in the blood, most commonly albumin. Drugs bound to albumin do not get filtered at the glomerulus or cross the blood-brain barrier. Binding to plasma proteins keeps drugs in the plasma resulting in a lower Vd. Highly protein bound drugs are good candidates for interactions with other drugs that are also highly bound (e.g., warfarin plus sulfonamides). 4. Answer: B. The permeation of most drugs through cellular membranes is by the process of passive diffusion, a nonsaturable process that follows first- order kinetics. Concentration gradient and lipid solubility are important determinants for the rate of diffusion. Only a few drugs are substrates for active transport processes such as active tubular secretion (e.g., penicillins) or penetrate membranes via aqueous pores (ethanol). 5. Answer: D. The formula to use is Cl = ff × GFR. The drug is 40% protein bound so the ff = 60%. 120 mL/min × 60% = 72 mL/min theoretical clearance of the drug. Since only 18 mL/min was actually cleared, there must have been tubular reabsorption of the drug. 72 – 18 = 54 mL/min of reabsorbed drug. 6. Answer: A. This is a “loading dose” question. The equation for loading dose or the volume of distribution equation can be used (LD = Vd × Cp). Since the patient weighs 70 kg and 60% of body weight is water, he has 42 L (70 L × 60%) of total body water. LD = 42 L × 5 mg/L = 210 mg. 7. Answer: A. Phase II drug metabolism involves the transfer of chemical groupings (e.g. acetyl, glucuronide, glutathione) to drugs or their metabolites via conjugation reactions involving transferase enzymes. Acetylation reactions are associated with a genetic polymorphism (slow acetylator). These individuals are slow to metabolize drugs via acetylation and are particularly susceptible to drug-induced SLE when taking hydralazine, procainamide, or isoniazid. Both oxidation and reduction are phase I metabolism reactions. 8. Answer: C. Azole antifungals (e.g. ketoconazole) are inhibitors of cytochrome P450 enzymes, especially CYP3A4, the most abundant isozyme form in the human liver. The 3A4 isozyme metabolizes a wide range of drugs. Ketoconazole would actually raise the plasma levels of oral contraceptives increasing the risk of side effects but it would not reduce their effectiveness. All other drugs listed are P450 inducers. As such, they would tend to lower plasma levels and decrease effectiveness of oral contraceptives. 9. Answer: A. The typical log dose response figure with the parallel nature of the curves suggests that the three drugs are interacting with the same receptor system. Drugs A and B are full agonists because they achieve the maximal response. They have the same efficacy. Drug A is more potent than drugs B or C. Drug B is more potent than drug C. Drug C is a partial agonist with less efficacy than the full agonists. 10. Answer: C. The fact that the drug has therapeutic efficacy for 6 h has no direct relationship to its half-life—it simply means that the drug is above its minimal effective concentration for 6 h. Doubling the dose (to 1 g) means that the drug level will be above the minimum for a longer period. Because the elimination half-life is 8 h, 500 mg of the drug will remain in the body 8 h after a dose of 1 g. Thus, the total duration of effectiveness must be 8 + 6 = 14 h. 11. Answer: E. In first-order kinetics, the elimination rate of a drug is directly proportional to its plasma concentration, which in turn is proportional to the dose. Drugs that follow first-order elimination have a constant elimination half-life similar to the example given in the question. Likewise, clearance and volume of distribution are pharmacokinetic characteristics of a drug that do not routinely change with dose, although they may vary in terms of disease or dysfunction. 12. Answer: B. The patient has renal dysfunction which reduces renal clearance. This would necessitate a lower maintenance dose for medications such as gentamicin. The maintenance dose equation factors in renal clearance while the loading dose equation does not. The t ½ of gentamicin would be increased in this patient due to the decrease in clearance, but the Vd would be unaffected. 13. 14. Answer: B. The rules for time to steady-state are that it takes 4–5 t ½ to reach clinical steady-state. It also takes one t ½ to get half way to steady-state. Since the drug got 50% of the way to steady-state in 6 hours, its t ½ must be 6 hours. 15. Answer: D. At 6 h after IV injection (which corresponds to two half-lives of the drug), the plasma level is 5 mg/L. Extrapolating back to zero time, “doubling” plasma level for each half-life results in an initial plasma level at zero time (C0) = 5 mg/L × 2 × 2 = 20 mg/L. Dose = C0 × Vd = 20 mg/L × 10 L = 200 mg 16. Answer: C. MD = Cl × Css × τ Since the drug was given by constant IV infusion there is no need to consider the dosing interval (τ). Therefore, 400 mg/h = 50 L/h × Css 400 mg/h ÷ 50 L/h = 8 mg/L Alternatively, you could evaluate the question this way: An infusion rate (k0) is given by: k0 = Cl × Css rearrange: Css = k0/Cl PART II Autonomic Pharmacology The Autonomic Nervous System 1 Learning Objectives Explain information related to anatomy of the ANS Solve problems concerning blood pressure control mechanisms Answer questions related to pupillary size and accommodation mechanisms ANATOMY OF THE ANS The autonomic nervous system (ANS) is the major involuntary portion of the nervous system, and is responsible for automatic, unconscious bodily functions (e.g., control of heart rate and blood pressure, and both gastrointestinal and genitourinary functions). It is divided into two subcategories: the parasympathetic autonomic nervous system (PANS) and the sympathetic autonomic nervous system (SANS). Location of ANS Ganglia Both the PANS and SANS have relay stations, or ganglia, between the CNS and the end organ, but the somatic system does not. An important anatomic difference between them is that the ganglia of the SANS lie in 2 paravertebral chains adjacent to the vertebral column, whereas most of the ganglia of the PANS system are located in the organs innervated. ANS and Somatic Innervation The figure below highlights the major features of the ANS and the somatic systems, and also shows the location of the major receptor types. Nicotinic receptors are located on cell bodies in ganglia of both NN PANS and SANS and in the adrenal medulla. Nicotinic receptors are located on the skeletal muscle motor end plate NM innervated by somatic motor nerves. M1– Muscarinic receptors are located on all organs and tissues innervated by postganglionic nerves of the PANS and on thermoregulatory sweat 3 glands innervated by the SANS. Figure II-1-1. Anatomy of the Autonomic Nervous System Neurotransmitters Acetylcholine (ACh) is the neurotransmitter at both nicotinic and muscarinic receptors in tissues that are innervated. Note that all direct transmission from the CNS (preganglionic and motor) uses ACh, but postganglionic transmission in the SANS system may use one of the organ-specific transmitters described below. Norepinephrine (NE) is the neurotransmitter at most adrenoceptors in organs, as well as in cardiac and smooth muscle. Dopamine (DA) activates D1 receptors, causing vasodilation in renal and mesenteric vascular beds. Epinephrine (E, from adrenal medulla) activates most adrenoceptors and is transported in the blood. BLOOD PRESSURE CONTROL MECHANISMS Autonomic Feedback Loop Blood pressure (BP) is the product of total peripheral resistance (TPR) and cardiac output (CO). Both branches of the ANS are involved in the autonomic (or neural) control of blood pressure via feedback mechanisms. Changes in mean BP are detected by baroreceptors, which relay information to the cardiovascular centers in the brainstem controlling PANS and SANS outflow. ‒ For example, an increase in mean BP elicits baroreceptor discharge, resulting in increased PANS activity, leading to bradycardia and decreased SANS activity, which leads, in turn, to decreased heart rate, force of contraction, and vasoconstriction. The resulting decreases in cardiac output and total peripheral resistance contribute to restoration of mean BP toward its normal level. ‒ Conversely, a decrease in BP elicits ANS neural feedback involving decreased PANS outflow and increased SANS activity—actions leading to an increase in cardiac output and total peripheral resistance. Note Baroreceptor reflexes can be blocked at the ganglionic synapse with NN receptor antagonists. Alternatively, a reflex bradycardia can be blocked with muscarinic antagonists; a reflex tachycardia can be blocked with β1 antagonists. Figure II-1-2. Autonomic Feedback Loop Hormonal Feedback Loop Blood pressure is also regulated via the hormonal feedback loop. The system is affected only by decreases in mean BP (hypotension), which results in decreased renal blood flow. Decreased renal pressure causes the release of renin, which promotes formation of the angiotensins. Angiotensin II increases aldosterone release from the adrenal cortex, which, via its mineralocorticoid actions to retain sodium and water, increases blood volume. Increased venous return results in an increase in cardiac output. Angiotensin II also causes vasoconstriction, resulting in an increase in TPR. Figure II-1-3. Hormonal Feedback Loop Note Both the ANS (neural) and endocrine feedback loops are invoked when patients are treated with antihypertensive drugs. Such compensatory mechanisms may result in tachycardia and both salt and water retention. Blood Pressure/Heart Rate Tracings Figure II-1-4. Blood Pressure/Heart Rate Tracings PUPILLARY SIZE AND ACCOMMODATION MECHANISMS Muscarinic stimulation 1. Miosis 2. Accommodation (near vision) Muscarinic antagonism 1. Mydriasis 2. Accommodation to far vision, leading to cycloplegia (paralysis of accommodation) Figure II-1-5. Effect of ANS Drugs on the Eye α1-agonists 1. Mydriasis 2. No cycloplegia Cholinergic Pharmacology 2 Learning Objectives Answer questions about cholinergic neuroeffector junctions Differentiate between muscarinic receptor activators, receptor antagonists, and nicotinic receptor antagonists CHOLINERGIC NEUROEFFECTOR JUNCTIONS Synthesis and Release of ACh 1 Hemicholinium 2 Botulinum toxin 3 Acetylcholinesterase (AChE) inhibitors 4 Receptor agonists and antagonists Figure II-2-1. Cholinergic Neuroeffector Junction Choline is accumulated in cholinergic presynaptic nerve endings via an active transport mechanism linked to a Na+ pump and similar to the sodium-dependent glucose transporter. Choline uptake is inhibited by hemicholinium (① in Figure II-2-1). ACh is synthesized from choline and acetyl-CoA via choline acetyltransferase (ChAT) and accumulates in synaptic vesicles. Presynaptic membrane depolarization opens voltage-dependent Ca2+ channels, and the influx of this ion causes fusion of the synaptic vesicle membranes with the presynaptic membrane, leading to exocytosis of ACh. Botulinum toxin (② in Figure II-2-1) interacts with synaptobrevin and other proteins to prevent ACh release and is used in blepharospasm, strabismus/hyperhidrosis, dystonia, and cosmetics. Some cholinergic nerve endings have presynaptic autoreceptors for ACh that on activation may elicit a negative feedback of transmitter release. Inactivation via acetylcholinesterase (AChE) is the major mechanism of termination of postjunctional actions of ACh. AChE is a target for inhibitory drugs (indirect-acting cholinomimetics). Note that such drugs can influence cholinergic function only at innervated sites where ACh is released. Reversible AChE inhibitors (③ in Figure II-2-1) include edrophonium, physostigmine, and neostigmine. Irreversible AChE inhibitors include malathion, and parathion. Postjunctional receptors (N and M) (④ in Figure II-2-1) activated by ACh are major targets for both activating drugs (direct-acting cholinomimetics) and blocking agents. Note M receptor activation →↓ CV function ↑ secretions and ↑ smooth muscle contraction All M receptor activators and blockers are nonspecific. M Receptor Location and Function Table II-2-1. Muscarinic Receptor Activation Target Receptor Response Eye Sphincter M3 Contraction—miosis Ciliary M3 Contraction—accommodation for near vision muscle Heart SA node M2 ↓Heart rate (HR)—negative chronotropy AV node M2 ↓ Conduction velocity—negative dromotropy No effects on ventricles, Purkinje system Lungs Bronchioles M3 Contraction—bronchospasm Glands M3 ↑ Secretion GI Stomach M3 ↑ Motility—cramps tract Glands ↑ Secretion M1 Intestine Contraction—diarrhea, involuntary defecation M3 Bladder M3 Contraction (detrusor), relaxation (trigone/sphincter), voiding, urinary incontinence Sphincters M3 Relaxation, except lower esophageal, which contracts Glands M3 ↑ Secretion—sweat (thermoregulatory), salivation, and lacrimation Blood vessels M3 Dilation (via NO/endothelium-derived relaxing factor)—no (endothelium) innervation, no effects of indirect agonists Table II-2-2. Nicotinic Receptor Activation Target Receptor Response Adrenal medulla NN Secretion of epinephrine and NE Autonomic ganglia NN Stimulation—net effects depend on PANS/SANS innervation and dominance Neuromuscular NM Stimulation—twitch/hyperactivity of skeletal muscle junction Note: N receptors desensitize very quickly upon excessive stimulation. Table II-2-3. Cholinergic Receptor Mechanisms M1 and M3 Gq coupled ↑ phospholipase C →↑ IP3, DAG, Ca2+ M2 Gi coupled ↓ adenylyl cyclase →↓ cAMP NN and NM No 2nd messengers activation (opening) of Na/K channels MUSCARINIC RECEPTOR ACTIVATORS Muscarinic Agonists Table II-2-4. Properties of Direct-Acting Cholinomimetics AChE Drug Activity Clinical Uses Hydrolysis ACh M and +++ Short half-life—no clinical use N Bethanechol M – Rx—ileus (postop/neurogenic), urinary retention Methacholine M> N + Dx—bronchial hyperreactivity Pilocarpine, M – Rx—xerostomia, glaucoma (pilocarpine) cevimeline Acetylcholinesterase Inhibitors Table II-2-5. Properties of Indirect-Acting Cholinomimetics Drug Characteristics Clinical Uses Edrophonium Short-acting Dx—myasthenia gravis Physostigmine Tertiary amine (enters Rx—glaucoma; antidote in atropine overdose CNS) Neostigmine, Quaternary amines Rx—ileus, urinary retention, myasthenia gravis, reversal pyridostigmine (no CNS entry) of nondepolarizing NM blockers Donepezil, Lipid-soluble (CNS Rx—Alzheimer disease rivastigmine entry) Organophosphates Lipid-soluble, Note: used as insecticides (malathion, parathion) and as irreversible inhibitors nerve gas (sarin) Clinical Correlate Alzheimer disease is late-onset dementia with progressive memory loss and cognitive decline. Neuropathology includes neurofibrillary tangles, amyloid plaques, and loss of ACh neurons in the Meynert nucleus— a rationale for clinical use of AChE inhibitors. Toxicity of AChE Inhibitors Excessive muscarinic and nicotinic stimulations Muscarinic effects: Diarrhea Urination Miosis Bradycardia Bronchoconstriction Lacrimation Salivation Sweating CNS stimulation Nicotinic effects: ‒ Skeletal muscle excitation followed by paralysis ‒ CNS stimulation Management Muscarinic effects: atropine Regeneration of AChE: pralidoxime (2-PAM) Time-dependent aging requires use of 2-PAM as soon as possible Figure II-2-2. Effects of Organophosphate on AChE Classic Clue AChE inhibitor poisoning: “Dumbbeelss” Diarrhea Urination Miosis Bradycardia Bronchoconstriction Emesis Excitation (CNS/muscle) Lacrimation Salivation Sweating MUSCARINIC RECEPTOR ANTAGONISTS Atropine Prototype of the class As a tertiary amine, it enters CNS Other M blockers differ mainly in their pharmacokinetic properties Pharmacologic Effects Atropine effects in order of increasing dose: ‒ Decreased secretions (salivary, bronchiolar, sweat) ‒ Mydriasis and cycloplegia ‒ Hyperthermia (with resulting vasodilation) ‒ Tachycardia ‒ Sedation ‒ Urinary retention and constipation ‒ Behavioral: excitation and hallucinations Other classes of drugs with antimuscarinic pharmacology: ‒ Antihistamines ‒ Tricyclic antidepressants ‒ Antipsychotics ‒ Quinidine ‒ Amantadine ‒ Meperidine Treatment of acute intoxication: symptomatic ± physostigmine Table II-2-6. Clinical Uses and/or Characteristics of M Blockers Drug Clinical Uses and/or Characteristics Atropine Antispasmodic, antisecretory, management of AChE inhibitor OD, antidiarrheal, ophthalmology (but long action) Tropicamide Ophthalmology (topical) Ipratropium, Asthma and COPD (inhalational)—no CNS entry, no change in mucus viscosity tiotropium Scopolamine Used in motion sickness, causes sedation and short-term memory block Benztropine, Lipid-soluble (CNS entry) used in parkinsonism and in acute extrapyramidal trihexyphenidyl symptoms induced by antipsychotics Oxybutynin Used in overactive bladder (urge incontinence) Recall Question The action of botulinum toxin is through which of the following mechanisms? A. Direct action on acetylcholine esterase B. Direct action on muscarinic receptors C. Prevention of acetylcholine release D. Prevention of choline uptake Answer: C Bridge to Physiology ANS Dominance For effector tissues with dual innervation, PANS is dominant. These include the SA and AV nodes of the heart, the pupil, GI and GU muscles, and sphincters. SANS is dominant only in terms of vascular tone and thermoregulatory sweat glands. NICOTINIC RECEPTOR ANTAGONISTS Ganglion Blocking Agents Drugs: hexamethonium and mecamylamine Reduce the predominant autonomic tone Prevent baroreceptor reflex changes in heart rate Table II-2-7. Effects of Ganglion Blocking Agents Effector System Effect of Ganglion Blockade Arterioles SANS Vasodilation, hypotension Veins SANS Dilation, ↓ venous return, ↓ CO Heart PANS Tachycardia Iris PANS Mydriasis Ciliary muscle PANS Cycloplegia GI tract PANS ↓ tone and motility—constipation Bladder PANS Urinary retention Salivary glands PANS Xerostomia Sweat glands SANS Anhydrosis Figure II-2-3. Algorithm: Reflex Control of Heart Rate Neuromuscular Blocking Drugs See CNS Pharmacology, chapter on Drugs Used in Anesthesia. Adrenergic Pharmacology 3 Learning Objectives Answer questions about catecholamine synthesis, action, and degradation Explain how direct-acting adrenoceptor agonists and indirect-acting adrenergic receptor agonists function Differentiate between alpha receptor antagonists and beta receptor antagonists ADRENERGIC NEUROEFFECTOR JUNCTIONS Synthesis and Release of NE The important aspects of the adrenergic neuroeffector junction are summarized below. 1 MAO inhibitors 2 Releasers 3 Reuptake blockers 4 α2 agonists and antagonists 5 Agonists and blockers of α1, β1 receptors Figure II-3-1. Adrenergic Neuroeffector Junction Tyrosine is actively transported into nerve endings and is converted to dihydroxyphenylalanine (DOPA) via tyrosine hydroxylase. This step is rate limiting in the synthesis of NE. DOPA is converted to dopamine (DA) via L-aromatic amino acid decarboxylase (DOPA decarboxylase). DA is taken up into storage vesicles where it is metabolized to NE via DA beta hydroxylase. Inactivation of NE via monoamine oxidase A (MAO-A) (1) may regulate prejunctional levels of transmitter in the mobile pool (2) but not the NE stored in granules. Presynaptic membrane depolarization opens voltage-dependent Ca2+ channels. Influx of this ion causes fusion of the synaptic granular membranes, with the presynaptic membrane leading to NE exocytosis into the neuroeffector junction. NE then activates postjunctional receptors (5), leading to tissue-specific responses depending on the adrenoceptor subtype activated. Termination of NE actions is mainly due to removal from the neuroeffector junction back into the sympathetic nerve ending via an NE reuptake transporter system (3). At some sympathetic nerve endings, the NE released may activate prejunctional alpha adrenoceptors (4) involved in feedback regulation, which results in decreased release of the neurotransmitter. Metabolism of NE is by catechol-O-methyltransferase (COMT) in the synapse or MAOA in the prejunctional nerve terminal. Adrenergic Receptor Location and Function Table II-3-1. Adrenergic Receptor Activation Receptor Response α1 Eye: radial (dilator) muscle Contraction: mydriasis Arterioles (skin, viscera) Contraction: ↑ TPR, ↑ diastolic pressure, ↑ afterload Veins Contraction: ↑ venous return, ↑ preload Bladder trigone and sphincter and Contraction: urinary retention prostatic urethra Male sex organs Vas deferens: ejaculation Liver ↑ glycogenolysis Kidney ↓ renin release α2 Prejunctional nerve terminals ↓ transmitter release and NE synthesis Platelets Aggregation Pancreas ↓ insulin secretion β1 Heart SA node ↑ HR (positive chronotropy) AV node ↑ conduction velocity (positive dromotropy) Atrial and ventricular muscle ↑ force of contraction (positive inotropy), conduction velocity, CO and oxygen c onsumption His-Purkinje ↑ automaticity and conduction velocity Kidney ↑ renin release β2(mostly not innervated) Blood vessels (all) Vasodilation: ↓ TPR: ↓ diastolic pressure, ↓ afterload Uterus Relaxation Bronchioles Dilation Skeletal muscle ↑ glycogenolysis: contractility (tremor) Liver ↑ glycogenolysis Pancreas ↑ insulin secretion β3 Detrusor muscle of the bladder Relaxation D1 (peripheral) Renal, mesenteric, coronary Vasodilation: in kidney ↑ RBF, ↑ GFR, ↑ Na+ secretion vasculature Table II-3-2. Mechanisms Used by Adrenergic Receptors α1 Gq coupled ↑ phospholipase C →↑ IP3, DAG, Ca2+ α2 Gi coupled ↓ adenylyl cyclase → ↓ cAMP β1β2D1 Gs coupled ↑ adenylyl cyclase → ↑ cAMP Note Adrenoceptor Sensitivity Beta receptors are usually more sensitive to activators than alpha receptors. With drugs that exert both effects, the beta responses are dominant at low doses; at higher doses, the alpha responses will predominate. Note Dopamine Use in Shock Fenoldopam is a D1 agonist used for severe hypertension. DIRECT-ACTING ADRENOCEPTOR AGONISTS α1 Agonists Systemically, alpha-1 agonists increase mean BP via vasoconstriction. Increased BP may elicit a reflex bradycardia Cardiac output may be ↓ but also offset by ↑ venous return Drugs and uses: ‒ Phenylephrine: nasal decongestant and ophthalmologic use (mydriasis without cycloplegia), hypotensive states Figure II-3-2. Effect of Alpha Activators on Heart Rate and Blood Pressure α2 Agonists Alpha-2 agonists stimulate prejunctional receptors in the CNS to decrease sympathetic outflow. Their primary use is for mild to moderate HTN. Drugs and uses: clonidine and methyldopa (mild to moderate hypertension) See Cardiovascular section. β Agonists Systemically, beta-agonists decrease mean BP via vasodilation (β2) and and increase HR (β1). Figure II-3-3. Effect of Beta Receptor Activation on Heart Rate and Blood Pressure Drugs and uses: ‒ Isoproterenol (β1 = β2) ‒ Dobutamine (β1 > β2): congestive heart failure ‒ Selective β2 agonists: salmeterol, albuterol, terbutaline (asthma); terbutaline (premature labor) ‒ Selective β3 agonist: mirabegron (overactive bladder) Mixed-Acting Agonists: Norepinephrine vs. Epinephrine Norepinephrine (α1,α2,β1) Figure II-3-4. Effect of Norepinephrine on Heart Rate and Blood Pressure Epinephrine (α1,α2,β1,β2) Figure II-3-5a. Effect of Low-dose Epinephrine on Heart Rate and Blood Pressure Figure II-3-5b. Effect of Medium-Dose Epinephrine on Heart Rate and Blood Pressure Figure II-3-5c. Effect of High-dose Epinephrine Is Similar to Norepinephrine Dose-dependent effects: ‒ Low-dose: β1, β2 stimulation (see Figure II-3-5a) ‒ High-dose: α1, β1 (β2) (see Figure II-3-5c) β2-specific effects: ‒ Smooth muscle relaxation: bronchioles, uterus, blood vessels ‒ Metabolic effects: ↑ glycogenolysis (muscle and liver) ↑ gluconeogenesis ↑ mobilization and use of fat Differentiation of high-dose epinephrine versus norepinephrine: ‒ Epinephrine reversal: Use of α1 blocker to reverse hypertension to hypotension in a patient receiving too much epinephrine ‒ Hypertension was due to predominant α1 tone on the vasculature ‒ Hypotension results from unmasking β2 receptors Uses of Norepinephrine and Epinephrine Cardiac arrest Adjunct to local anesthetic Hypotension Anaphylaxis (epinephrine only) Asthma (epinephrine only) INDIRECT-ACTING ADRENERGIC RECEPTOR AGONISTS Releasers Releasers displace norepinephrine from the mobile pool. Clinical Correlate Indirect-acting adrenoceptor agonists act only on effector tissues innervated by SANS. Denervated effector tissues are nonresponsive because these drugs act either to release transmitter from nerve terminals or to inhibit neurotransmitter reuptake. Note Forms of MAO MAO type A: mainly in liver, but Anywhere (metabolizes NE, 5HT, and tyramine) MAO type B: mainly in Brain (metabolizes DA) Drug interaction: MAOA inhibitors (hypertensive crisis) Tyramine (red wine, cheese) ‒ Oral bioavailability is limited by MAO-A metabolism in gut and liver ‒ MAO-A inhibition ↑ bioavailability, resulting in hypertensive crisis Amphetamines ‒ Clinical use of methylphenidate in narcolepsy and ADHD ‒ Psychostimulant due to central release of DA, NE, 5HT Ephedrine (cold medication) Reuptake Inhibitors Cocaine Tricyclic antidepressant (in part) ADRENERGIC ANTAGONISTS α Receptor Antagonists Alpha-receptor antagonists decrease TPR and decrease mean BP. May cause reflex tachycardia and salt and water retention Major uses: ‒ Hypertension ‒ Pheochromocytoma (nonselective α blocker) ‒ Benign prostatic hyperplasia (BPH; selective α1 blocker) Drugs: ‒ Nonselective blocker: phentolamine (competitive inhibitor), phenoxybenzamine (noncompetitive inhibitor) ‒ Selective α1 blocker: prazosin, doxazosin, terazosin, tamsulosin ‒ Selective α2 blocker: mirtazapine (used as antidepressant) β Receptor Antagonists Clinical Correlate Chronic use of beta blockers (e.g., in angina, HTN) leads to receptor upregulation. During withdrawal from use, it is important to taper dose to avoid excessive cardiovascular effects (rebound effects) of endogenous amines. Clinical Correlate Glucagon and the Heart Positive inotropic and chronotropic, not via activation of β1 receptors, but through glucagon receptors that are Gs -protein linked to adenylyl cyclase → basis for its use in beta-blocker overdose. β1 blockade: ‒ ↓ HR, ↓ SV, ↓ CO ‒ ↓ renin release β2 blockade: ‒ May precipitate bronchospasm (in asthmatics) and vasospasm (in patients with vasospastic disorders) ‒ ↓ aqueous humor production ‒ Metabolic effects Blocks glycogenolysis, gluconeogenesis ↑ LDLs, TGs Table II-3-3. Characteristics of Some Beta Blockers Drugs β1-Selective ISA Sedation Blood Lipids Acebutolol + ++ + – Atenolol + – – ↑↑ Metoprolol + – + ↑↑ Pindolol – ++ + – Propranolol – – +++ ↑↑ Timolol – – ++ ↑↑ Cardioselectivity (β1): ‒ Less effect on vasculature, bronchioles, uterus, and metabolism ‒ Safer in asthma, diabetes, peripheral vascular diseases Intrinsic sympathomimetic activity (ISA): ‒ Act as partial agonists ‒ Less bradycardia (β1) ‒ Slight vasodilation or bronchodilation (β2) ‒ Minimal change in plasma lipids (β2) Pharmacokinetic properties: no CNS entry of atenolol General uses of beta-blockers: ‒ Angina, hypertension, post-MI (all drugs) ‒ Antiarrhythmics (class II: propranolol, acebutolol, esmolol) ‒ Glaucoma (timolol) ‒ Migraine, thyrotoxicosis, performance anxiety, essential tremor (propranolol) Combined alpha-1 and beta blocking activity: ‒ Labetalol and carvedilol ‒ Use in CHF (carvedilol) and in hypertensive emergencies (labetalol) + K -channel blockade and β-blocking activity: sotalol Recall Question Which of the following directly results from activation of the beta 2 receptor? A. Decrease in blood pressure B. Increase in cardiac output C. Increase in heart rate D. Increase in stroke volume Answer: A Autonomic Drugs: Glaucoma Treatment and ANS Practice 4 Problems Learning Objectives Solve problems concerning glaucoma treatment GLAUCOMA TREATMENT Figure II-4-1. Anatomy of the Eye Showing Irido-Corneal Angle Where Aqueous Humor Is Recirculated Open-Angle Glaucoma Open-angle glaucoma is a chronic condition with increased intraocular pressure (IOP) due to decreased reabsorption of aqueous humor. It leads to progressive (painless) visual loss and, if left untreated, blindness. IOP is a balance between fluid formation and its drainage from the globe. Strategies in drug treatment of glaucoma include the use of beta blockers to decrease formation of fluid by ciliary epithelial cells and the use of muscarinic activators to improve drainage through the canal of Schlemm. Closed-Angle Glaucoma Closed-angle glaucoma is an acute (painful) or chronic (genetic) condition with increased IOP due to blockade of the canal of Schlemm. Emergency drug management prior to surgery usually involves cholinomimetics, carbonic anhydrase inhibitors, and/or mannitol. Note Antimuscarinic drugs and α1 agonists are contraindicated in closed-angle glaucoma. Treatment Table II-4-1. Mechanism of Action of Drugs Used to Treat Glaucoma Drug Drug Class Mechanism of Action Pilocarpine Cholinomimetic Activation of M receptors causes contraction of ciliary muscle, which increases flow through the canal of Schlemm Timolol Beta blockers Block actions of NE at ciliary epithelium ↓ aqueous humor formation ANS PRACTICE PROBLEMS 1. R is A. Epinephrine B. Norepinephrine C. Phenylephrine D. Isoproterenol E. Terbutaline 2. U is A. Epinephrine B. Norepinephrine C. Phenylephrine D. Isoproterenol E. Tyramine 3. S is A. Epinephrine B. Norepinephrine C. Phenylephrine D. Isoproterenol E. Terbutaline 4. H is A. Epinephrine B. Norepinephrine C. Phenylephrine D. Isoproterenol E. Albuterol 5. Drug X is most like A. Epinephrine B. Isoproterenol C. Norepinephrine D. Phenylephrine E. Terbutaline 6. X and Y are, respectively: A. Isoproterenol and Propranolol B. Epinephrine and Phenoxybenzamine C. Norepinephrine and Phentolamine D. Terbutaline and Phenylephrine E. Acetylcholine and Hexamethonium 7. What is drug X? A. Hexamethonium B. Neostigmine C. Atropine D. Scopolamine E. Ipratropium 8. Given the following information: Contractile force is measured in an isolated arterial preparation, and heart rate is measured in an isolated heart preparation. One drug is added at each specified time. No washout between drugs A. Bethanechol B. Epinephrine C. Phenoxybenzamine D. Pindolol E. Phenylephrine Time 1: Time 2: Time 3: Time 4: Time 5: 9. The circles below represent the size of the pupils of a patient’s eyes, without treatment and with two different treatments. The responses are compatible with the conclusion that the left eye had: A. been pretreated with atropine. B. been pretreated with prazosin. C. been pretreated with propranolol. D. been pretreated with physostigmine. E. denervation of the radial muscle. 10. A. Acetylcholine B. Hydralazine P is C. Norepinephrine Q is D. Isoproterenol R is E. Edrophonium S is ANSWERS AND EXPLANATIONS 1. Answer: B. The effects of Drug R are changed by treatment with either an alpha or beta-blocker, so Drug R must have activity at both receptors (choices C, D, and E are ruled out). A pressor dose of epinephrine would be “reversed” by an alpha-blocker, not just decreased! Drug R is norepinephrine. 2. Answer: C. The effects of Drug U are changed by treatment with the alpha- blocker, but not by the beta-blocker. Drug U must be an alpha-activator with no beta actions—the only choice is phenylephrine. 3. Answer: D. The effects of Drug S are changed by treatment with the beta- blocker, but not by the alpha blocker (choices A, B, and C are ruled out). Terbutaline is β2 selective and would not increase heart rate directly. Drug S is isoproterenol. Note that option A would have been a possibility but one would have to assume a low-dose of epinephrine. 4. Answer: A. The effects of Drug H are changed by treatment with either an alphaor beta- blocker, so Drug H must have activity at both receptors (choices C, D, and E are ruled out). “Reversal” of a pressor effect can only occur if the drug has β2 activity (choice B is ruled out). Drug H is epinephrine. 5. Answer: E. Mecamylamine blocked reflexed tachycardia induced by Drug X, which dropped blood pressure by vasodilation. Propranolol prevented all responses. Drug X is a β2 agonist (terbutaline). 6. Answer: D. Drug X decreases TPR and BP, eliciting a reflex sympathetic discharge (note delay in response), resulting in increased CO. There is no direct effect on CO (choices A, B, C, and E are ruled out). Drugs X and Y are terbutaline and phenylephrine. Note that the alpha agonist does not antagonize the decrease in respiratory resistance (a β2 response). 7. Answer: A. ACh (used as a drug) decreases blood pressure and heart rate, but the latter effect is overcome and reversed by a sympathetic reflex. Because Drug X abolishes only the reflex tachycardia, it must be the ganglion blocker hexamethonium (choice A). Remember, AChE inhibitors do not vasodilate because there is no parasympathetic innervation of the vasculature! 8. No autonomic reflexes are possible in isolated preparations, so every action observed in this experiment is due to a direct action of the drug. Time 1: Arterial contraction is due to an alpha-1 agonist (choice E phenylephrine). Time 2: Arterial contraction is reversed by an alpha antagonist (choice C phenoxybenzamine). Time 3: Tachycardia and arterial dilation are due to beta-1 and beta-2 actions (choice B epinephrine at a low dose). Time 4: Tachycardia and arterial dilation are reversed by a non-selective beta blocker (choice D). Time 5: Bethanechol (choice A bethanechol) causes both arterial relaxation and bradycardia. 9. Answer: E. Classic example showing that denervated tissues do not respond to indirect-acting agonists. In this case, amphetamine fails to cause mydriasis in the left eye, but this eye is more responsive than the right eye to phenylephrine (denervation supersensitivity). 10. Block of tachycardia due to Drug P by hexamethonium is indicative of a sympathetic reflex that follows a decrease in BP due to a vasodilator (choice B hydralazine). “Reversal” of bradycardia due to Drug Q by hexamethonium indicates a vagal reflex elicited by vasoconstriction (e.g., alpha activation) masking cardiac stimulation (e.g., beta activation) typical of norepinephrine (choice C norepinephrine). Tachycardia due to Drug R is unaffected by any antagonist, indicative of a beta activator (choice D isoproterenol). “Reversal” of tachycardia due to Drug S by hexamethonium indicates a sympathetic reflex masking a vagomimetic action typical of a muscarinic activator (choice A acetylcholine); this is confirmed by the effect of atropine Autonomic Drug List and Practice Questions 5 Cholinergic Receptor Activators Direct activators: bethanechol (M), methacholine (M and N), nicotine (N), pilocarpine (M), cevimeline (M) AChE inhibitors: reversible—edrophonium, physostigmine, neostigmine, pyridostigmine, donepezil, rivastigmine AChE inhibitors: irreversible—malathion, parathion Cholinergic Receptor Antagonists Muscarinic blockers: atropine, benztropine, ipratropium, scopolamine Ganglionic blockers: hexamethonium, mecamylamine Adrenergic Receptor Activators α1 agonists: phenylephrine α2 agonists: clonidine, methyldopa β agonists: isoproterenol, (β1= β2), dobutamine (β1> β2) β2 agonists: albuterol, terbutaline, salmeterol β3 agonist: mirabegron Mixed: dopamine (D1, β1, α1), epinephrine (α1, α2, β1, β2), norepinephrine (α1, α2, β1) Indirect-acting: amphetamine, cocaine, ephedrine, tyramine Adrenergic Receptor Antagonists α1 antagonists: doxazosin, prazosin, terazosin α2 antagonists: mirtazapine Mixed α antagonists: phenoxybenzamine, phentolamine β1 (cardioselective) antagonists: acebutolol, atenolol, metoprolol β1, β2 (nonselective): pindolol, propranolol, timolol α1 and β antagonists: carvedilol, labetalol PRACTICE QUESTIONS 1. Alpha-1 agonists cause reflex bradycardia, which can be blocked by A. atenolol B. atropine C. mirtazapine D. phenylephrine E. propranolol 2. Which one of the following effects is caused by the ingestion of mushrooms that contain pilocarpine? A. Tachycardia B. Bronchodilation C. Diarrhea D. Hypertension E. Hyperthermia 3. Increasing the concentration of norepinephrine in adrenergic synapses leads to A. activation of dopa decarboxylase B. increased release of norepinephrine C. activation of presynaptic Gi coupled receptors D. stimulation of MAO E. activation of tyrosine hydroxylase 4. Urination in the human subject is decreased by A. muscarinic agonists B. muscarinic antagonists C. AChase inhibitors D. Nicotinic agonists E. Spider venom 5. A 5-year-old child becomes ill while visiting relatives who have a farm in Arkansas. His symptoms include severe abdominal cramps with vomiting and diarrhea and profuse lacrimation and salivation. Pupillary constriction is marked. The most likely cause is exposure to A. herbicides B. antifreeze C. lead-based paint D. insecticides E. rat poison 6. The activation of muscarinic receptors in bronchiolar smooth muscle is associated with A. activation of adenylyl cyclase B. decrease in cAMP formation mediated by G-proteins C. increase in IP3 and DAG D. inhibition of protein kinase C E. opening of Na+/K+ cation channels 7. Ganglion blocking agents are of little clinical value today but they are important drugs to know for solving cardiovascular drug problems because they can block A. all muscarinic receptors B. all nicotinic receptors C. all autonomic reflexes D. the direct actions of drugs on blood vessels E. the direct actions of drugs on the heart 8. An 11-year-old boy was brought to the ER by some of his friends because he “started going crazy” after eating seeds from a plant while “trying to get high.” The boy was incoherent; his skin was hot and dry. His pupils were dilated and unresponsive to light. Blood pressure was 180/105 mm Hg, pulse 150/min, and rectal 40 C (104 F). The presumptive diagnosis was drug toxicity due to the ingestion of a compound similar to A. cannabis B. digoxin C. mescaline D. phencyclidine E. scopolamine 9. Reflex tachycardia caused by the systemic administration of albuterol can be blocked by what drug? A. dobutamine B. prazosin C. phenylephrine D. metoprolol E. low-dose epinephrine 10. Cardiovascular effects of a new drug (X) that activates autonomic receptors are shown in the table below: Parameter Control Drug X Systolic BP 120 mm Hg 110 mm Hg Diastolic BP 85 mm Hg 55 mm Hg Heart rate 60/min 120/min The most probable receptor affinities of drug X are A. α1, α2 B. α1, α2, β1 C. β1, β2 D. M2 E. NM 11. Thermoregulatory sweat glands in the body utilize what type of pathway? A. Cholinergic nerves and muscarinic receptors B. Adrenergic nerves and alpha-1 receptors C. Adrenergic nerves and beta-2 receptors D. Cholinergic nerves and NM receptors E. Neurohumorally-released epinephrine 12. Activation of postsynaptic M2 receptors on the heart is associated with A. activation of adenylyl cyclase B. decrease in cAMP formation C. increase in IP3 and DAG D. inhibition of protein kinase C E. opening of Na+/K+ cation channels 13. The data in the table below show the effects of four drugs (#1–4) on mean blood pressure administered as individual agents before and after treatment with prazosin. The arrows denote the direction and intensity of drug actions on blood pressure. Condition Drug #1 Drug #2 Drug #3 Drug #4 Before prazosin ↑↑ ↑↑ ↓↓ ↑ After prazosin ↑ ↑ ↓↓ ↓ The order of drug #1 through drug #4 is best represented by A. epinephrine—tyramine—isoproterenol—norepinephrine B. tyramine—isoproterenol—norepinephrine—epinephrine C. norepinephrine—isoproterenol—epinephrine—tyramine D. isoproterenol—epinephrine—tyramine—norepinephrine E. norepinephrine—tyramine—isoproterenol—epinephrine 14. Prior to an eye exam a patient is given a drug that causes mydriasis but has no effect on accommodation. What is the most likely identity of this drug? A. mecamylamine B. neostigmine C. pilocarpine D. phenylephrine E. tropicamide 15. Following a myocardial infarct, a 40-year-old man is being treated prophylactically with propranolol. You would be concerned about the use of this drug if the patient also had what comorbid condition? A. Essential tremor B. Glaucoma C. Classic/stable angina D. Supraventricular tachycardia E. Diabetes 16. Following pretreatment with a muscarinic receptor blocking agent, the IV administration of norepinephrine is likely to result in A. ↑HR and ↑ BP B. ↑ HR and ↓ BP C. ↓ HR and ↓ BP D. ↓ HR and ↑ BP E. no effect on HR, but ↑ BP 17. A 45-year-old man has recently been the recipient of a heart transplant. Which one of the following drugs is least likely to cause tachycardia in this patient? A. Amphetamine B. Dobutamine C. Epinephrine D. Isoproterenol E. Norepinephrine 18. A colleague with myasthenia gravis wants you to assist him to the ER because he is experiencing muscle weakness and has found it difficult to titrate his drug dosage because he has had the “flu.” You note that he has a slight temperature, shallow respirations, and a gray-blue skin pallor. What would be the most appropriate drug to give to your colleague at this time? A. Albuterol B. Edrophonium C. Propranolol D. Physostigmine E. Scopolamine 19. Carvedilol is an effective antihypertensive agent that, like propranolol, is capable of blocking beta receptors. An important difference between the two drugs is that carvedilol A. is a selective blocker of cardiac β1 receptors B. has intrinsic sympathomimetic activity C. is available only as eye drops D. has α1 receptor blocking actions E. stimulates β2 receptors in bronchioles 20. Neostigmine differs from pilocarpine in having effects on A. bladder tone B. bowel motility C. heart rate D. salivary glands E. skeletal muscle Questions 21–23 The table below shows the effects of three receptor activators on heart rate in anesthetized animals, administered as individual drugs and following pretreatment with one of four different receptor antagonists. The arrows denote the direction of effects on heart rate; the symbol (–) denotes no change from normal HR. Antagonist Pretreatment Agonist 1 Agonist 2 Agonist 3 None ↑ ↓ ↓ Atropine ↑ – ↑ Prazosin ↑ – ↑ Propranolol – ↓ ↓ Mecamylamine ↑ – ↑ Identify the agonist drugs from the following list: A. Acetylcholine B. Low-dose epinephrine C. Norepinephrine D. Phenylephrine E. Physostigmine 21. Agonist 1 22. Agonist 2 23. Agonist 3 ANSWERS AND EXPLANATIONS 1. Answer: B. Bradycardia due to vagal stimulation is elicited by activation of muscarinic receptors in the heart. Atropine, which is an antagonist at M receptors, blocks bradycardia elicited by stimulation of the vagus, including reflex bradycardia due to increases in mean BP caused by vasoconstrictors. 2. Answer: C. Pilocarpine is present in several mushroom species including Amanita muscaria, the ingestion of which is associated with the stimulation of M receptors (parasympathomimetic effects). Activation of muscarinic receptors in the GI tract causes diarrhea. The activation by pilocarpine of M receptors present on vascular endothelial cells would lead to hypotension (not hypertension) via the release of NO. All of the other effects listed are typical of muscarinic antagonists. 3. Answer: C. In sympathetic nerve endings presynaptic α2 receptors are coupled to inhibitory G-proteins. These receptors serve an autoregulatory function to inhibit further neurotransmitter release and also to decrease the synthesis of norepinephrine. 4. Answer: B. Urinary retention is a well known adverse effect of drugs that have antagonist effects on muscarinic receptors. In addition to the prototypic drug atropine, M blockers include drugs used in Parkinson disease, such as benztropine. Acetylcholine directly and AChE inhibitors (edrophonium, physostigmine) indirectly activate M receptors in the GU system, causing bladder contraction with voiding and incontinence. Activation of nicotinic receptors in ANS ganglia would lead to the stimulation of PANS functions. 5. Answer: D. The symptoms of cholinergic excess seen in this child are indicative of exposure to insecticides such as the organophosphate parathion, which cause irreversible inhibition of acetylcholinesterase. Other symptoms may include CNS excitation and stimulation of the skeletal NMJ, ultimately leading to paralysis of respiratory muscles—“DUMBBEELSS.” In addition to symptomatic support, management of AChE inhibitor poisoning involves the use of atropine and 2-PAM. 6. Answer: C. Muscarinic receptors present in bronchiolar smooth muscle are of the M3 subtype coupled via Gq proteins to phospholipase C. Activation of this enzyme causes hydrolysis of phosphatidylinositol bisphosphate, with release of IP3 and DAG (the latter activates protein kinase C). Decreased formation of cAMP mediated via a Gi protein occurs with activation of M2 receptors such as those in the heart. Cation channel opening occurs in response to activation of nicotinic receptors. 7. Answer: C. Ganglion blockers (hexamethonium, mecamylamine) block NN receptors at autonomic ganglia and the adrenal m