Hematopoietic Introduction.pdf
Document Details
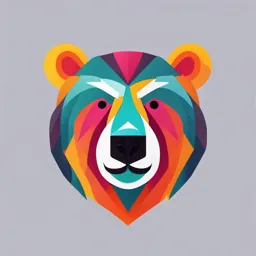
Uploaded by WellBacklitAppleTree
Tags
Full Transcript
CH APTER 13 Bone Marrow, Blood Cells, and the Lymphoid/Lymphatic Systema Amy C. Durham and Katie M. Boes Key Readings Index Bone Marrow and Blood Cells, 809 Structure and Function, 809 Dysfunction/Responses to Injury, 815 Portals of Entry/Pathways of Spread, 828 Defense Mechanisms/Barrier Systems, 8...
CH APTER 13 Bone Marrow, Blood Cells, and the Lymphoid/Lymphatic Systema Amy C. Durham and Katie M. Boes Key Readings Index Bone Marrow and Blood Cells, 809 Structure and Function, 809 Dysfunction/Responses to Injury, 815 Portals of Entry/Pathways of Spread, 828 Defense Mechanisms/Barrier Systems, 828 Diseases Affecting Multiple Species of Domestic Animals, 829 Diseases of Horses, 843 Diseases of Ruminants (Cattle, Sheep, and Goats), 843 Diseases of Pigs, 844 Diseases of Dogs, 844 Diseases of Cats, 845 Lymphoid/Lymphatic System, 846 Structure and Function, 846 Dysfunction/Responses to Injury, 858 Portals of Entry/Pathways of Spread, 863 This chapter discusses (1) the structure, function, and diseases of the bone marrow and bone marrow–derived blood cells, such as erythrocytes, granulocytes (e.g., neutrophils, eosinophils, and basophils), circulating lymphocytes (e.g., B lymphocytes, T lymphocytes, and natural killer [NK] cells), monocytes, and platelets, and (2) the structure, function, and diseases of the lymphoid/lymphatic organs consisting of the primary lymphoid organs (e.g., bone marrow and thymus) and secondary lymphoid organs (e.g., lymph and hemal nodes, spleen, and mucosa-associated lymphoid tissues [MALT]). The afferent and efferent lymphatic circulation supporting these organs are discussed in Chapter 10, Cardiovascular System, Pericardial Cavity, and Lymphatic Vessels. Bone Marrow and Blood Cellsb Hematopoiesis, from haima (Greek [Gr.], blood) and poiein (Gr., to make), is the production of blood cells, including erythrocytes, leukocytes, and platelets. Also known as hemopoiesis, hematopoiesis first occurs in the blood islands of the yolk sac and then transitions to the liver and spleen during gestation. After birth, the primary hematopoietic site is the central cavities of bone, termed bone marrow (Fig. 13.1). Hematopoiesis occurring elsewhere is called extramedullary hematopoiesis (EMH), which is most common in the spleen. Structure and Function Stromal Compartment Bone. The bone marrow is supported by an anastomosing network of trabecular bone that radiates centrally from the compact bone of the cortex. Trabecular bone is covered by periosteum, consisting of an inner osteogenic layer of endosteal cells, osteoblasts, aFor a glossary of abbreviations and terms used in this chapter, see E-Glossary 13.1. bFor tests to evaluate platelet function or immune-mediated thrombocytopenia see E-Appendix 13.1. Defense Mechanisms/Barrier Systems, 864 Diseases Affecting Multiple Species of Domestic Animals, 864 Diseases of Horses, 880 Diseases of Ruminants (Cattle, Sheep, and Goats), 881 Diseases of Pigs, 883 Diseases of Dogs, 884 Diseases of Cats, 889 and osteoclasts, and an outer fibrous layer that anchors the stromal scaffolding of the marrow spaces. Reticular Cells (Fibroblasts). Within the marrow spaces, a network of stromal cells and extracellular matrix provides metabolic and structural support to hematopoietic cells. These stromal cells consist of adipocytes and specialized fibroblasts, called reticular cells. The latter provides structural support by producing a fine network of a type of collagen, called reticulin, and by extending long cytoplasmic processes around other cells and structures. Both reticulin and cytoplasmic processes are not normally visible with light microscopy but are visible with silver reticulin stains (e.g., Gordon and Sweet’s and sometimes with periodic acid–Schiff). Adipose Tissue (Adipocytes). Marrow adipocytes store or provide energy, secrete adipose-derived hormones, termed adipokines, and help regulate the marrow environment by paracrine signaling and cytokine secretion. Leptin and adiponectin are adipokines that regulate appetite, insulin sensitivity and secretion, the cardiovascular and immune systems, the thyroid glands, and bone mass. The composition of the hematopoietic cells and adipose tissue changes with age. The general pattern is that hematopoietic tissue (red marrow) regresses and is replaced with nonhematopoietic tissue, mainly fat (yellow marrow). Thus, in newborns and very young animals the bone marrow consists largely of hematopoietically active tissue, with relatively little fat, whereas in geriatric individuals the marrow consists largely of fat. In adults, hematopoiesis occurs primarily in the pelvis, sternum, ribs, vertebrae, and the proximal ends of humeri and femora. Even within these areas of active hematopoiesis, fat may constitute a significant proportion of the marrow volume. Blood Vessels and Endothelium. Bone marrow is highly vascularized but does not have lymphatic drainage. Marrow of long bones receives part of its blood supply from the nutrient artery, which enters the bone via the nutrient canal at midshaft. The remaining arterial supply enters the marrow through an anastomosing array 809 CHAPTER 13 Bone Marrow, Blood Cells, and the Lymphoid/Lymphatic System 809.e1 E-Glossary 13.1 Glossary of Abbreviations and Terms AA amyloidosis Serum amyloid A amyloidosis ACT Activated clotting time ADP Adenosine diphosphate AL amyloidosis Amyloid light chain amyloidosis ALL Acute lymphoblastic leukemia AML Acute myeloid leukemia AP3 Adaptor protein complex ATALT Auditory tube–associated lymphoid tissue ATP Adenosine triphosphate BALT Bronchus-associated lymphoid tissue BCR-ABL Breakpoint cluster region-Abelson BLAD Bovine leukocyte adhesion deficiency BLL Burkitt-like lymphoma BLP B lymphocyte progenitor BLV Bovine leukemia virus BNP Bovine neonatal pancytopenia BVD Bovine viral diarrhea BVDV Bovine viral diarrhea virus CalDAG-GEFI Calcium diacylglycerol guanine nucleotide exchange factor I CALT Conjunctiva-associated lymphoid tissue CBC Complete blood count C-bilirubin Conjugated bilirubin CD Cluster of differentiation CH Cutaneous histiocytosis CHS Chédiak-Higashi syndrome CLAD Canine leukocyte adhesion deficiency CLL Chronic lymphocytic leukemia CLP Common lymphoid progenitor CML Chronic myeloid leukemia CMP Common myeloid progenitor CPV-2 Canine parvovirus type 2 CTCL Cutaneous T cell lymphoma DC Dendritic cell DIC Disseminated intravascular coagulation DLBCL Diffuse large B cell lymphoma DNA Deoxyribonucleic acid DNA-PKcs DNA-dependent protein kinase catalytic subunit 2,3-DPG 2,3-diphosphoglycerate EATCL Enteropathy-associated T cell lymphoma EBL Enzootic bovine leukosis EBV Epstein-Barr virus EHV-1 Equine herpesvirus 1 EHV-5 Equine herpesvirus 5 EIAV Equine infectious anemia virus EMH Extramedullary hematopoiesis EMP Extramedullary plasmacytoma EP Erythroid progenitor Epo Erythropoietin FAD Flavin adenine dinucleotide FAE Follicle-associated epithelium FcaGHV1 Felis catus gammaherpesvirus 1 Fe3+ Ferric iron FeLV Feline leukemia virus FIV Feline immunodeficiency virus FL Follicular lymphoma FPV Feline parvovirus GALT Gut-associated lymphoid tissue GMP Granulocyte-macrophage progenitor GP Granulocyte progenitor G6PD Glucose-6-phosphate dehydrogenase Gr. Greek GSH Reduced glutathione GT Glanzmann thrombasthenia H&E Hematoxylin and eosin HEV High endothelial venule Hemoglobin Hgb Hpt Haptoglobin Hpx Hemopexin HS Histiocytic sarcoma HSC Hematopoietic stem cell IBD Inflammatory bowel disease iDC Ig IgA IgG IgM IL IMHA IMTP IFN IRF4 LAD LALT LBL LC LGL LYST MAC MALT MAP M cell MCF MCH MCHC MCL MCP MCT MCV MDS MEP MetHgb MHC miRNA MKP MM MP MPV MUM1 MZL NADH NADPH NALT NCI NI NK cell NKP nRBC PALS PAMS PARR PCR PCV2 PCVAD PFK PHA PK PL PMWS PPP PRCA PRDC PrPSc PRRS PT PTCL PTT RBC REAL rhEpo SCID Interstitial dendritic cell Immunoglobulin Immunoglobulin A Immunoglobulin G Immunoglobulin M Interleukin Immune-mediated hemolytic anemia Immune-mediated thrombocytopenia Interferon Interferon regulatory factor 4 Leukocyte adhesion deficiency Larynx-associated lymphoid tissue Lymphoblastic lymphoma Langerhans cell Large granular lymphocyte Lysosomal trafficking regulator Membrane attack complex Mucosa-associated lymphoid tissue Mycobacterium avium ssp. paratuberculosis Microfold cell Malignant catarrhal fever Mean cell hemoglobin Mean cell hemoglobin concentration Mantle cell lymphoma Mast cell progenitor Mast cell tumor Mean cell volume Myelodysplastic syndrome Megakaryocyte-erythroid progenitor Methemoglobin Major histocompatibility complex microRNA Megakaryocyte progenitor Multiple myeloma Macrophage progenitor Mean platelet volume Melanoma-associated antigen (mutated) 1 Marginal zone lymphoma Reduced nicotinamide adenine dinucleotide Reduced nicotinamide adenine dinucleotide phosphate Nasal-associated lymphoid tissue National Cancer Institute Neonatal isoerythrolysis Natural killer cell Natural killer cell progenitor Nucleated red blood cell Periarteriolar lymphoid sheath Periarteriolar macrophage sheath Polymerase chain reaction for antigen receptor rearrangement Polymerase chain reaction Porcine circovirus type 2 Porcine circovirus–associated disease Phosphofructokinase Pelger-Huët anomaly Pyruvate kinase Persistent lymphocytosis Postweaning multisystemic wasting syndrome Pentose phosphate pathway Pure red cell aplasia Porcine respiratory disease complex Scrapie prion protein Porcine reproductive and respiratory syndrome Prothrombin time Peripheral T cell lymphoma Partial thromboplastin time Red blood cell Revised European-American Classification of Lymphoid Neoplasms Recombinant human erythropoietin Severe combined immunodeficiency disease (Continued) 809.e2 SECTION II Pathology of Organ Systems E-Glossary 13.1 Glossary of Abbreviations and Terms—cont’d SFHN SH SPF TCRLBCL TGF-β TLP TNF Splenic fibrohistiocytic nodule Systemic histiocytosis Specific pathogen–free T cell–rich large B cell lymphoma Transforming growth factor-β T lymphocyte progenitor Tumor necrosis factor TNKP Tpo TZL U-bilirubin vWD vWF WHO E-Appendix 13.1 Tests to Evaluate Platelet Function or Immune-Mediated Thrombocytopenia Bleeding time (template bleeding time, buccal mucosal bleeding time). This assay assesses primary hemostasis (platelet plug formation) by measuring the time interval between inflicting of standardized wound and cessation of bleeding. Sedation may be required. In small animals the test is usually performed on the buccal mucosa; in large animals it may be performed on the distal limb. Prolonged bleeding time may be because of a platelet function defect, von Willebrand disease, or a vascular defect. The sensitivity of this test is low; reference intervals are species and site dependent (can perform test on a normal animal as a control). This test is contraindicated in cases of thrombocytopenia because significant thrombocytopenia can cause a prolonged bleeding time (invalidates interpretation of test results). Clot retraction test. This assay assesses retraction of a clot, in which platelets play an essential role. This is a crude test that is rarely performed. Different protocols are described. Significant thrombocytopenia invalidates interpretation of test results. Tests to characterize platelet function abnormalities more specifically are available through specialized laboratories. A ggregometry—To assess platelet aggregation in response to different physiologic agonists A dhesion assays—To assess the ability of platelets to adhere to a substrate (e.g., collagen) F low cytometry—To assay for expression of surface molecules P FA-200—An instrument that simulates a damaged blood vessel, by measuring time for a platelet plug to occlude an aperture; to date, this instrument has mainly been used in research applications T hromboelastography (TEG)—Global assessment of hemostasis (platelets, coagulation, and fibrinolysis) based on viscoelastic analysis of whole blood Tests for immune-mediated thrombocytopenia (IMTP) F low cytometry—To detect immunoglobulin bound to the platelet surface, using a fluorescent-labeled antibody B one marrow immunofluorescent antibody (IFA) test—To detect bound immunoglobulin. Sometimes referred to as the “antimegakaryocyte antibody test,” this assay actually detects the presence of immunoglobulin nonspecifically: a smear of a bone marrow aspirate is incubated with a fluorescent-labeled antibody to species-specific immunoglobulin Tests for Evaluating the Coagulation System A ctivated partial thromboplastin time (aPTT or PTT) Required sample: citrated plasma or whole blood (analyzer dependent) Measures time for fibrin clot formation after addition of a contact activator, calcium, and a substitute for platelet phospholipid T lymphocyte–natural killer cell progenitor Thrombopoietin T zone lymphoma Unconjugated bilirubin von Willebrand disease von Willebrand factor World Health Organization D eficiencies/dysfunction in intrinsic and/or common coagulation pathway (all factors except for VII and XIII) causes prolongation of PTT Insensitive test—Prolongation requires 70% deficiency Other causes of prolongation include polycythemia (less plasma per unit volume, so excess amount of citrate is available to chelate calcium) and heparin therapy Activated clotting time (ACT) Required sample—Nonanticoagulated whole blood in special ACT tube (diatomaceous earth as contact activator) Used in practice setting—Performed by warming sample to body temperature, monitoring for clot formation; normal clotting times are within 60 to 90 seconds in dogs, 165 seconds in cats Less sensitive version of PTT—Prolongation requires 95% deficiency Severe thrombocytopenia may cause prolongation One-stage prothrombin time (OSPT or PT) Required sample—Citrated plasma or whole blood (analyzer dependent) Measures time for fibrin clot formation after addition of tissue factor (TF; thromboplastin), calcium, and a substitute for platelet phospholipid Deficiencies/dysfunction in extrinsic (factor VII) and/or common coagulation pathway cause prolongation of PT Insensitive test—Prolongation requires 70% deficiency Proteins induced by vitamin K antagonism or absence (PIVKA) test Required sample—Citrated plasma Essentially a version of the PT using an especially sensitive thromboplastin reagent PIVKA are inactive (uncarboxylated) vitamin K–dependent factors; an increase in PIVKA is not specific for vitamin K antagonism but may be an earlier and more sensitive detector than PT or PTT Thrombin time (TT) Required sample—Citrated plasma Measures time for fibrin clot formation after thrombin (factor IIa) is added Defects directly involving formation and/or polymerization of fibrin prolong this test (i.e., if the lesion is upstream of the conversion of fibrinogen to fibrin, the TT will be normal). Hypofibrinogenemia or dysfibrinogenemia causes prolongation of the TT Fibrinogen Required sample—Citrated plasma Fibrinogen concentration measured based on time to clot formation after addition of thrombin; this is essentially the same as the TT mentioned earlier and is a more accurate method than the heat precipitation method Decreased fibrinogen may be because of increased consumption (disseminated intravascular coagulation) or decreased production (liver disease) CHAPTER 13 Bone Marrow, Blood Cells, and the Lymphoid/Lymphatic System I ncreased fibrinogen is associated with inflammation, renal disease, and dehydration Fibrin degradation products (FDPs) Required sample—Special FDP tube Historically used in the practice setting Performed by adding blood to a special tube containing thrombin and a trypsin inhibitor (sample clots almost instantly in normal dogs and cats) and incubating two dilutions of serum (1:5 and 1:20) with polystyrene latex particles coated with sheep anti-FDP antibodies (should be negative in normal dogs and cats, but positive results have been reported in normal cats) D-dimer Required sample—Citrated plasma Latex agglutination test using monoclonal antibodies To date, only validated in dogs, cats, and horses 809.e3 A ssay detects a specific type of FDP resulting from breakdown of cross-linked fibrin; concentration of plasma D-dimer indicates the degree of fibrinolysis; often used as part of a disseminated intravascular coagulation panel; can be used as a negative predictor to rule out pathologic thrombosis (e.g., pulmonary thromboembolism); also increased when there is appropriate clotting Antithrombin (antithrombin III [ATIII]) Required sample—Citrated plasma Decreased because of decreased production (liver disease), loss (protein-losing nephropathy or enteropathy), consumption (disseminated intravascular coagulation), and chronic heparin therapy Specific factor assays Required sample—Citrated plasma Performed at specialized laboratories 810 SECTION II Pathology of Organ Systems and cell-matrix interactions and by soluble mediators, such as cytokines and hormones, that interact with cells and with matrix proteins. Cells localize to specific niches within the hematopoietic microenvironment via adhesion molecules, such as integrins, immunoglobulins, lectins, and other receptors, which recognize ligands on other cells or matrix components. Cells also express receptors for soluble molecules such as chemokines (chemoattractant cytokines) and hormones that influence cell trafficking and metabolism. Monocyte-Macrophage System. Other components of the marrow include low numbers of resident macrophages, lymphocytes, and plasma cells. Of note, the macrophages play an important role in iron storage and erythrocyte maturation. Hematopoietic Compartment Erythroid and myeloid precursors (hematopoietic cells) undergo differentiation and maturation in marrow spaces before their release into vascular sinusoids. Vascular sinusoids are entered by hematopoietic cells via diapedesis or proplatelet shearing. Trabecular bone structurally supports the marrow. Osteoblasts produce trabecular bone. Endothelial cells sit on a basal lamina and separate the vascular sinusoidal lumens from marrow hematopoietic and stromal cells. Megakaryocytes line vascular sinusoids and release cytoplasmic fragments (platelets) into sinusoidal lumens. Stromal cells provide structural and metabolic support to hematopoietic cells. Adipocytes constitute 25% to 75% of the total marrow space. The proportion of adipocytes increases with age. Figure 13.1 Structure of Bone Marrow. (Courtesy Dr. K.M. Boes, College of Veterinary Medicine, Virginia Polytechnic Institute and State University; and Dr. J.F. Zachary, College of Veterinary Medicine, University of Illinois.) of vessels that arise from the periosteal arteries and penetrate the cortical bone. Vessels from the nutrient and periosteal arteries converge and form an interweaving network of venous sinusoids that permeates the marrow. These sinusoids not only deliver nutrients and remove cellular waste but also act as the entry point for hematopoietic cells into blood circulation. Sinusoidal endothelial cells function as a barrier and regulate traffic of chemicals and particles between the intravascular and extravascular spaces. Venous drainage parallels that of the nutrient artery and its extensions. Extracellular Matrix. Hematopoiesis occurs in the interstitium between the venous sinusoids in the so-called hematopoietic spaces. There is a complex functional interplay among hematopoietic cells with the supporting connective tissue cells, extracellular matrix, and soluble factors, which form the hematopoietic microenvironment. Behavior of hematopoietic cells is influenced by direct cell-to-cell The hematopoietic compartment consists of immature erythroid, myeloid, megakaryocytic, and lymphocytic cells that undergo proliferation and maturation within the bone marrow. The mature forms of these cells are ultimately released into blood circulation by traversing the marrow sinusoidal endothelial cells. The following basic concepts provide a framework for understanding the mechanisms of injury and diseases presented later in the chapter. Hematopoietic tissue is highly proliferative. Billions of cells per kilogram of body weight are produced each day. Pluripotent hematopoietic stem cells (HSCs) are a self-renewing population, giving rise to cells with committed differentiation programs, and are common ancestors of all blood cells. The process of hematopoietic differentiation is shown in Fig. 13.2. Hematopoietic cells undergo sequential divisions as they develop, so there are progressively higher numbers of cells as they mature. Cells also continue to mature after they have stopped dividing. Conceptually, it is helpful to consider cells in the bone marrow as belonging to mitotic and postmitotic compartments. Examples of developing hematopoietic cells are shown in Fig. 13.3. Mature cells released into the blood circulation have different normal life spans, varying from hours (neutrophils), to days (platelets), to months (erythrocytes), and to years (some lymphocytes). The hematopoietic system is under exquisite local and systemic control and responds rapidly and predictably to various stimuli. Production and turnover of blood cells are balanced so that numbers are maintained within normal ranges (steady-state kinetics) in healthy individuals. Normally the bone marrow releases mostly mature cell types (and very low numbers of cells that are almost fully mature) into the circulation. In response to certain physiologic or pathologic stimuli, however, the bone marrow releases immature cells that are further back in the supply “pipeline.” The composition of the marrow changes with age. The general pattern is that hematopoietic tissue (red marrow) regresses and is replaced with nonhematopoietic tissue, mainly fat (yellow marrow). Thus, in newborns and very young animals the bone marrow consists largely of hematopoietically active tissue, with relatively little fat, whereas in geriatric individuals the marrow consists largely of fat. In adults, hematopoiesis occurs primarily in the pelvis, sternum, ribs, vertebrae, and the proximal ends of humeri and femora. Even within these areas of active hematopoiesis, fat may constitute a significant proportion of the marrow volume. Hematopoiesis. Immature hematopoietic cells can be divided into three stages: stem cells, progenitor cells, and precursor cells. HSCs have the capacity to self-renew, differentiate into mature cells, and repopulate the bone marrow after it is obliterated. Progenitor cells and precursor cells cannot self-renew; with each cell division, they 811 CHAPTER 13 Bone Marrow, Blood Cells, and the Lymphoid/Lymphatic System Bone marrow Thymus Hematopoetic stem cell Self-Renewal Progenitor cells CMP CLP ? MEP BLP GMP TNKP ? MKP MP EP GP MCP Monocyte Megakaryocyte Polychromatophil NKP B lymphocyte NK cell TLP T lymphocyte Neutrophil, basophil, eosinophil Blood Platelets Erythrocytes Tissues B lymphocyte Macrophage Neutrophil, basophil, eosinophil NK cell Mast cell T lymphocyte Plasma cell Figure 13.2 Classic and Spatial Model of Hematopoietic Cell Differentiation, Canine Blood Smears, and Bone Marrow Aspirate. The bone marrow consists of (1) hematopoietic stem cells, pluripotent cells capable of self-renewal; (2) progenitor cells that evolve into more differentiated cells with each cell division; (3) precursor cells that can be identified by light microscopy (not shown, see Fig. 13.3); and (4) mature hematopoietic cells awaiting release into the blood vasculature. The earliest lineage commitment is to either the common myeloid progenitor (CMP), which produces platelets, erythrocytes, and nonlymphoid leukocytes, or the common lymphoid progenitor (CLP), which differentiates into various lymphocytes and plasma cells. The cell origin of mast cells is unclear, but they may originate from a stem cell or a myeloid progenitor. Megakaryocytes remain in the bone marrow and release cytoplasmic fragments, or platelets, into blood sinusoids. T lymphocyte progenitor (TLP) cells travel from the bone marrow to the thymus during normal T lymphocyte maturation. During homeostasis, platelets and erythrocytes remain in circulation, but the leukocytes leave blood vessels to enter the tissues, where they actively participate in immune responses. In particular, monocytes and B lymphocytes undergo morphologic and immunologic changes to form macrophages and plasma cells, respectively. Macrophages, granulocytes, and mast cells migrate unidirectionally into tissues, but lymphoid cells can recirculate between the blood, tissues, and lymphatic vessels. BLP, B lymphocyte progenitor; EP, erythroid progenitor; GMP, granulocyte-macrophage progenitor; GP, granulocyte progenitor; MCP, mast cell progenitor; MEP, megakaryocyte-erythroid progenitor; MKP, megakaryocyte progenitor; MP, macrophage progenitor; NK cell, natural killer cell; NKP, natural killer cell progenitor; TLP, T lymphocyte progenitor; TNKP, T lymphocyte–natural killer cell progenitor. (Courtesy Dr. K.M. Boes, College of Veterinary Medicine, Virginia Polytechnic Institute and State University; and Dr. J.F. Zachary, College of Veterinary Medicine, University of Illinois.) evolve into more differentiated cells. Later-stage precursors cannot divide. Stem cells and progenitor cells require immunochemical stains for identification, but precursor cells can be identified by their characteristic morphologic features (see Fig. 13.3). Control of hematopoiesis is complex, with many redundancies, feedback mechanisms, and pathways that overlap with other physiologic and pathologic processes. Many cytokines influence cells of different lineages and stages of differentiation. Primary growth factors for primitive cells are interleukin (IL)-3, produced by T lymphocytes, and stem cell factor, produced by monocytes, macrophages, fibroblasts, endothelial cells, and lymphocytes. IL-7 is an early lymphoid growth factor. Lineage-specific growth factors are discussed in their corresponding sections. Erythropoiesis. Erythropoiesis—from erythros (Gr., red)—refers to the production of red blood cells (RBCs), or erythrocytes, whose primary function is gas exchange; oxygen is delivered from the lungs to the tissues, and carbon dioxide is transported from the tissues to the lungs. During maturation, erythroid precursors synthesize a large quantity of a metalloprotein, called hemoglobin (Hgb), to facilitate gas transportation. Erythrocytes have secondary functions, such as blood acid-base buffering. 812 SECTION II Pathology of Organ Systems Rubriblast Prorubricyte Basophilic rubricyte Polychromatophilic rubricyte Metarubricyte Polychromatophil (left) Mature erythrocyte (right) Myeloblast Promyelocyte Neutrophilic myelocyte Neutrophilic metamyelocyte Band neutrophil Segmented neutrophil Eosinophilic myelocyte Eosinophilic metamyelocyte Band eosinophil Segmented eosinophil Basophilic myelocyte Basophilic metamyelocyte Band basophil Segmented basophil Monoblast Promonocyte Monocyte Figure 13.3 Hematopoietic Cell Morphology, Feline (Erythroid and Granulocyte Lineages) and Canine (Monocyte Lineage) Blood Smears and Bone Marrow Aspirates. As erythroid cells mature from a rubriblast to a mature erythrocyte, their nuclei become smaller and more condensed. The nucleus is eventually extruded to form a polychromatophil. Erythroid cells also become less basophilic and more eosinophilic as more hemoglobin (Hgb) is produced and as RNA-rich organelles are lost during maturation. (Hemoglobin stains eosinophilic, and RNA stains basophilic with routine Romanowsky stains.) As granulocytes (e.g., neutrophils, eosinophils, and basophils) mature from a myeloblast to their mature forms, their nuclei become dense and segmented. Granulocytes acquire their secondary or specific granules during the myelocyte stage and can be morphologically differentiated starting at this stage. Neutrophils have neutral-staining secondary granules, eosinophil secondary granules have an affinity for acidic or eosin dyes, and basophil secondary granules have an affinity for basic dyes. Monoblasts differentiate into promonocytes with ruffled nuclear borders and then into monocytes. (Courtesy Dr. K.M. Boes, College of Veterinary Medicine, Virginia Polytechnic Institute and State University; and Dr. J.F. Zachary, College of Veterinary Medicine, University of Illinois.) The dominant regulator of erythropoiesis is a glycoprotein aptly named erythropoietin (Epo). Other direct or indirect stimulators of erythropoiesis include ILs (e.g., IL-3, IL-4, and IL-9), colony-stimulating factors (e.g., granulocyte-macrophage colony-stimulating factor and granulocyte colony-stimulating factor), and hormones (e.g., growth hormone, insulin-like growth factor, testosterone, and thyroid hormone). Epo is synthesized primarily in the kidney and exerts its effects by promoting proliferation and inhibiting apoptosis of developing erythroid cells. The stimulus for increased Epo production is hypoxia. Within the bone marrow, erythroid precursors surround a central macrophage in specialized niches, termed erythroblastic islands (Fig. 13.4). The central macrophage, also known as a nurse cell, anchors the precursors within the island niche, regulates erythroid proliferation and differentiation, transfers iron to the erythroid progenitors (EPs) for Hgb synthesis, and phagocytizes extruded metarubricyte nuclei. Although erythroblastic islands occur throughout the marrow, those with more differentiated erythroid cells neighbor sinusoids, whereas nonadjacent islands contain mostly undifferentiated precursors. Iron is essential to Hgb synthesis and function. It is acquired through the diet and is transported to the bone marrow via the iron transport protein, transferrin. Central macrophages either store iron as ferritin or hemosiderin or transfer the iron to erythroid precursors for Hgb synthesis. Hemosiderin is identifiable in routinely stained CHAPTER 13 Bone Marrow, Blood Cells, and the Lymphoid/Lymphatic System marrow preparations as an intracellular brown pigment. However, Perls’ Prussian blue stain is more sensitive and specific for iron detection. The earliest erythroid precursor identifiable by routine light microscopy is the rubriblast, which undergoes maturational division to produce 8 to 32 progeny cells. Late-stage erythroid precursors, known as metarubricytes, extrude their nuclei and become reticulocytes, and subsequently mature erythrocytes. The normal transit time from rubriblast to mature erythrocyte is approximately 1 week. Reticulocytes start maturing in the bone marrow but finish their maturation in the blood circulation and spleen. Horses are an exception in that they do not release reticulocytes into circulation, even in situations of increased demand. Unlike mature erythrocytes, which lack organelles, reticulocytes still contain ribosomes and mitochondria, mainly to support completion of Hgb synthesis. These remaining organelles impart a bluish-purple cast (polychromasia) to reticulocytes on routine blood smear examination. The resultant cells are termed polychromatophils. Because older reticulocytes do not exhibit polychromasia, more sensitive laboratory techniques must be used for accurate reticulocyte quantification. When a blood sample is incubated with new methylene blue stain, Figure 13.4 Erythroblastic Island, Canine Splenic Aspirate. Erythroid precursors surround and adhere to a central macrophage, or nurse cell (arrow), which regulates the erythroid cell’s maturation and iron acquisition. (Courtesy Dr. K.M. Boes, College of Veterinary Medicine, Virginia Polytechnic Institute and State University.) A 813 the reticulocytes’ ribosomal RNA precipitates to form irregular, dark aggregates (Fig. 13.5). Cats also have a more mature form of reticulocyte, termed punctate reticulocyte, which is stippled when stained with new methylene blue. Punctate reticulocytes indicate prior, not active, regeneration and do not appear polychromatophilic on routine blood smear evaluation. In most mammals, mature erythrocytes have a biconcave disk shape, called a discocyte. Microscopically, these cells are round and eosinophilic with a central area of pallor. However, the central concavity may not be microscopically apparent in species other than the dog. Camelids normally have oval erythrocytes, termed ovalocytes or elliptocytes, which facilitate better gas exchange at high altitudes. The erythrocytes of some animals are prone to in vitro shape change, including those of cervids, pigs, and some goat breeds (e.g., Angora). Erythrocyte size during health depends on the species, breed, and age of the animal. In dogs, some breeds have relatively smaller (e.g., Akitas and Shibas) or larger (e.g., some poodles) erythrocytes. Akitas and Shibas also have a high concentration of potassium, unlike erythrocytes in other dogs. Juvenile animals may have larger erythrocytes because of the persistence of fetal erythrocytes, which is followed by a period of relatively smaller cells before reaching adult reference intervals. Mature mammalian erythrocytes lack nuclei and organelles and are thus incapable of transcription, translation, and oxidative metabolism. However, they do require energy for various functions, including maintenance of shape and deformability, active transport, and prevention of oxidative damage. RBCs generate this energy entirely through glycolysis (also known as the Embden-MeyerhofParnas pathway). Except in pigs, glucose enters erythrocytes from the plasma through an insulin-independent, integral membrane glucose transporter. Within circulation the erythrocyte mean life span varies between species and is related to body weight and metabolic rate: approximately 150 days in horses and cattle, 100 days in dogs, and 70 days in cats. When erythrocytes reach the end of their life span, they are destroyed in a process termed hemolysis. Hemolysis may occur within blood vessels (intravascular hemolysis) or by sinusoidal macrophages (extravascular hemolysis). During intravascular hemolysis, erythrocytes release their contents, mostly Hgb, directly into blood. However, during extravascular hemolysis, macrophages phagocytize entire erythrocytes, leaving little or no Hgb in the blood. Normal turnover of erythrocytes occurs mainly by extravascular hemolysis within the spleen, and to a lesser extent in other organs such as the liver and bone marrow. The exact controls are B Figure 13.5 Reticulocytosis, Canine Blood Smears. A, Reticulocytes (arrows) appear polychromatophilic with routine staining. Wright’s stain. B, Reticulocytes. Precipitated aggregates of RNA are stained blue (arrows) with special staining. New methylene blue stain. (Courtesy Dr. M.M. Fry, College of Veterinary Medicine, University of Tennessee.) 814 SECTION II Pathology of Organ Systems not clear, but factors that likely play a role in physiologic hemolysis include the following: Exposure of membrane components normally sequestered on the inner leaflet of the erythrocyte membrane, particularly phosphatidylserine Decreased erythrocyte deformability Binding of immunoglobulin G (IgG) and/or complement to erythrocyte membranes. Complement binding may be secondary clustering of the membrane anion exchange protein, band 3 Oxidative damage to erythrocytes Macrophages degrade erythrocytes into reusable components, such as iron and amino acids, and the waste product bilirubin. Bilirubin is then exported into circulation, where it is transported to the liver by albumin. The liver conjugates and subsequently excretes bilirubin into bile for elimination from the body. Intravascular hemolysis normally occurs at only extremely low levels. Hgb is a tetramer that, when released from the erythrocyte into the blood, splits into dimers that bind to a plasma protein called haptoglobin (Hpt). The Hpt-Hgb complex is taken up by hepatocytes and macrophages. This is the major pathway for handling free Hgb. However, free Hgb may also oxidize to form methemoglobin (MetHgb), which dissociates to form metheme and globin. Metheme binds to a plasma protein called hemopexin (Hpx), which is taken up by hepatocytes and macrophages in a similar manner to Hpt-Hgb complexes. Free heme in the reduced form binds to albumin, from which it is taken up in the liver and converted into bilirubin. The concentration of circulating erythrocytes typically decreases postnatally and remains below normal adult levels during the period of rapid body growth. The age at which erythrocyte numbers begin to increase and the age at which adult levels are reached vary among species. In dogs, adult values are usually reached between 4 and 6 months of age; in horses, this occurs at approximately 1 year of age. These granulocytic precursors are conceptually divided into those stages that can divide, including myeloblasts, promyelocytes, and myelocytes (proliferation pool), and those that cannot, including metamyelocytes, and band and segmented forms (maturation pool). Within the neutrophil maturation pool is a subpool, termed the storage pool, which consists of a reserve of fully mature neutrophils. The size of the storage pool varies by species; it is large in the dog, but small in ruminants. In homeostasis, mostly mature segmented granulocytes are released from the marrow into the blood. The first monocytic precursor identifiable by morphologic features is the monoblast, which develops into promonocytes and subsequently monocytes (see Fig. 13.3). Unlike granulocytes, monocytes do not have a marrow storage pool; they immediately enter venous sinusoids upon maturation. After migrating into the tissues, monocytes undergo morphologic and immunophenotypic maturation into macrophages. Within blood vessels there are two pools of leukocytes: the circulating pool and the marginating pool. Circulating cells are free flowing in blood, whereas marginating cells are temporarily adhered to endothelial cells by selectins. In most healthy mammals, there are typically equal numbers of neutrophils in the circulating and marginal pools. However, there are threefold more marginal neutrophils relative to circulating neutrophils in cats. Only the circulating leukocyte pool is sampled during phlebotomy. The concentration of myeloid cells in blood depends on the rate of production and release from the bone marrow, the proportions of cells in the circulating and marginating pools, and the rate of migration from the vasculature into tissues. The fate of neutrophils after they leave the bloodstream in normal conditions (i.e., not in the context of inflammation) is poorly understood. They migrate into the gastrointestinal and respiratory tracts, liver, and spleen and may be lost through mucosal surfaces or undergo apoptosis and be phagocytized by macrophages. Granulopoiesis and Monocytopoiesis (Myelopoiesis). Granulopoiesis is the production of neutrophils, eosinophils, Lymphopoiesis. Lymphopoiesis—from lympha (Latin, water)— refers to the production of new lymphocytes, including B lymphocytes, T lymphocytes, and natural killer (NK) cells. B lymphocytes primarily produce immunoglobulins, also known as antibodies, and are key effectors of humoral immunity. They are distinguished by the presence of an immunoglobulin receptor complex, termed the B lymphocyte receptor. Plasma cells are terminally differentiated B lymphocytes that produce abundant immunoglobulin. T lymphocytes, effectors of cell-mediated immunity, possess T lymphocyte receptors that bind antigens prepared by antigen-presenting cells. A component of innate immunity, NK cells kill a variety of infected and tumor cells in the absence of prior exposure or priming. Main growth factors for B lymphocytes, T lymphocytes, and NK cells are IL-4, IL-2, and IL-15, respectively. Lymphocytes are derived from HSCs within the bone marrow. B lymphocyte development occurs in two phases, first in an antigen-independent phase in the bone marrow and ileal Peyer’s patches (the site of B lymphocyte development in ruminants), then in an antigen-dependent phase in peripheral lymphoid tissues (e.g., the spleen, lymph nodes, and MALT). T lymphocyte progenitors (TLPs) migrate from the bone marrow to the thymus, where they undergo differentiation, selection, and maturation processes before migrating to the peripheral lymphoid tissue as effector cells. Unlike granulocytes, which circulate only in blood vessels and migrate unidirectionally into target tissues, lymphocytes travel in both blood and lymphatic vessels and continually circulate between blood, tissues, and lymphatic vessels. Also, in contrast to nonlymphoid hematopoietic cells, blood lymphocyte concentrations in and basophils, whereas monocyte production is termed monocytopoiesis. Granulocytic and monocytic cells are sometimes collectively referenced as myeloid cells. However, the term myeloid and the prefix myelo- can be confusing because they have other meanings; they may reference the bone marrow, all nonlymphoid hemic cells (erythrocytes, leukocytes, and megakaryocytes), only granulocytes, or the spinal cord. The main purpose of granulocytes and monocytes is to migrate to sites of tissue inflammation and function in host defense (see Chapter 3, Inflammation and Healing, and Chapter 5, Diseases of Immunity). Briefly, these cells have key immunologic functions, including phagocytosis and microbicidal activity (neutrophils and monocyte-derived macrophages), parasiticidal activity and participation in allergic reactions (eosinophils and basophils), antigen processing and presentation, and cytokine production (macrophages). Neutrophils are the predominant leukocyte type in blood of most domestic species. Primary stimulators of granulopoiesis and monocytopoiesis are granulocyte-macrophage colony-stimulating factor and IL-1, IL-3, and IL-6 (granulocytes and monocytes), granulocyte colony-stimulating factor (granulocytes), and macrophage colony-stimulating factor (monocytes). In general, these cytokines are produced by various inflammatory cells, with or without contribution from stromal cells. The earliest granulocytic precursor identifiable by routine light microscopy is the myeloblast, which undergoes maturational division over 5 days to produce 16 to 32 progeny cells (see Fig. 13.3). CHAPTER 13 Bone Marrow, Blood Cells, and the Lymphoid/Lymphatic System Box 13.1 815 Mechanisms of Disease in Bone Marrow and Blood Cells BONE MARROW Hypoplasia Hyperplasia Dysplasia Aplasia Neoplasia Myelophthisis (fibrosis, metastatic neoplasia) Necrosis Inflammation BLOOD CELLS Figure 13.6 Megakaryocyte, Canine Bone Marrow Aspirate. Note the cell’s very large size, lobulated nucleus, and abundant granular cytoplasm. Wright’s stain. (Courtesy Dr. M.M. Fry, College of Veterinary Medicine, University of Tennessee.) adult animals are primarily dependent upon extramedullary lymphocyte production and kinetics, and not lymphopoiesis by the marrow. In healthy nonruminant mammals, lymphocytes are the second most numerous blood leukocyte. According to conventional wisdom, cattle normally have higher numbers of lymphocytes than neutrophils in circulation. However, recent studies suggest that is no longer the case, most likely because of changes in genetics and husbandry. In most species, the majority of lymphocytes in blood circulation are T lymphocytes. The concentration of blood lymphocytes decreases with age. Increased destruction Hemorrhage (especially erythrocytes) Consumption (platelets) Neoplasia Altered distribution Abnormal function binding sites for the extrinsic tenase (factors III, VII, and X), intrinsic tenase (factors IX, VIII, and X), and prothrombinase (factors X, V, and II) coagulation complexes. Platelet glycoprotein surface receptors include those for binding vWF (GPIb-IX-V), collagen (GPVI), and fibrinogen (GPIIb-IIIa), which facilitate platelet aggregation and adherence to subendothelial collagen. Expansion of surface area and release of granule contents are aided by a network of membrane invaginations known as the open canalicular system. This system is not present in horses, cattle, and camelids. Methods for Examination of the Bone Marrow Gross and Microscopic Examination Thrombopoiesis. Thrombopoiesis—from thrombos (Gr., clot)— refers to the production of platelets, which are small (2 to 4 μm), round to ovoid, anucleate cells within blood vessels. Platelets have a central role in primary hemostasis but also participate in secondary hemostasis (coagulation) and inflammatory pathways (see Chapter 2, Vascular Disorders and Thrombosis, and Chapter 3, Inflammation and Healing). Thrombopoietin (Tpo) is the primary regulator of thrombopoiesis. The liver and renal tubular epithelial cells constantly produce Tpo, which is then cleared and destroyed by platelets and their precursors. Therefore, plasma Tpo concentration is inversely proportional to platelet and platelet precursor mass. If the platelet mass is decreased, less Tpo is cleared, and there is subsequently more free plasma Tpo to stimulate thrombopoiesis. The earliest morphologically identifiable platelet precursor is the megakaryoblast, which undergoes nuclear reduplications without cell division, termed endomitosis, to form a megakaryocyte with 8 to 64 nuclei. As the name suggests, megakaryocytes are very large cells, much larger than any other hematopoietic cell (Fig. 13.6; also see Fig. 13.1). Megakaryocytes neighbor venous sinusoids, extend their cytoplasmic processes into vascular lumens, and shed membrane-bound cytoplasmic fragments (platelets) into blood circulation. Orderly platelet shedding is partially facilitated by β1-tubulin microtubules within megakaryocytes. Platelets circulate in a quiescent form and become activated by binding platelet agonists, including thrombin, adenosine diphosphate (ADP), and thromboxane. Platelet activation causes shape change, granule release, and relocation of procoagulant phospholipids and glycoproteins to the outer cell membrane. Specific procoagulant actions include release of calcium, von Willebrand factor (vWF), factor V, and fibrinogen, as well as providing phosphatidylserine-rich Information on this topic is available at www.expertconsult.com. Complete Blood Count Information on this topic is available at www.expertconsult.com. Additional Tests Information on biopsies, direct antiglobulin test, flow cytometry, immunophenotyping, and polymerase chain reaction (PCR) is available at www.expertconsult.com. Hemostasis Testing Information on this topic is available at www.expertconsult.com. Dysfunction/Responses to Injury Bone Marrow Mechanisms of bone marrow disease are summarized in Box 13.1. Hematopoietic cells’ response to injury is dependent upon whether the insult is on the marrow or within extramarrow tissues. In general, marrow-directed injury or disturbances result in production of abnormal hematopoietic cells (dysplasia), fewer hematopoietic cells (hypoplasia),c or a failure of hematopoietic cell development (aplasia). Dysplasia, hypoplasia, and aplasia may be specific for one cThe term “hypoplasia” is used differently with regard to the bone marrow versus nonmarrow sites. Outside of the marrow, hypoplasia is used to indicate that a tissue or organ failed to initially develop. Marrow hypoplasia indicates that hematopoietic cells decreased production after normal development. This would be termed “atrophy” in nonmarrow sites.