Guyton & Hall 14th ed 3.pdf Genetic Control of Protein Synthesis, Cell Function, and Cell Reproduction PDF
Document Details
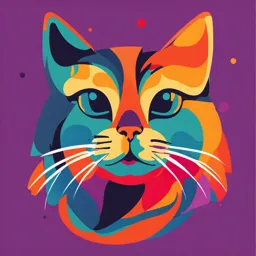
Uploaded by yvesss
Ayura 2027
Tags
Summary
This document details the genetic control of protein synthesis, cell function, and cell reproduction. It explains how genes determine which structures, enzymes, and chemicals are synthesized within the cell. It includes diagrams and figures to illustrate the concepts.
Full Transcript
CHAPTER 3 Genetic Control of Protein Synthesis, Cell...
CHAPTER 3 Genetic Control of Protein Synthesis, Cell UNIT I Function, and Cell Reproduction Genes, which are located in the nuclei of all cells of the Building Blocks of DNA body, control heredity from parents to children, as well Figure 3-3 shows the basic chemical compounds involved as the daily functioning of all the body’s cells. The genes in the formation of DNA. These compounds include the control cell function by determining which structures, following: (1) phosphoric acid; (2) a sugar called deoxyri- enzymes, and chemicals are synthesized within the cell. bose; and (3) four nitrogenous bases (two purines, adenine Figure 3-1 shows the general schema of genetic con- and guanine, and two pyrimidines, thymine and cytosine). trol. Each gene, which is composed of deoxyribonucleic The phosphoric acid and deoxyribose form the two heli- acid (DNA), controls the formation of another nucleic cal strands that are the backbone of the DNA molecule, acid, ribonucleic acid (RNA); this o RNA then spreads and the nitrogenous bases lie between the two strands throughout the cell to control formation of a specific pro- and connect them, as illustrated in Figure 3-2.␣ tein. The entire process, from①transcription of the genetic code in the nucleus to①translation of the RNA code and Nucleotides the formation of proteins in the cell cytoplasm, is often The first stage of DNA formation is to combine one mol- referred to as gene expression. ecule of phosphoric acid, one molecule of deoxyribose, and Because the human body has approximately 20,000 one of the four bases to form an acidic nucleotide. Four to 25,000 different genes that code for proteins in each separate nucleotides are thus formed, one for each of the cell, it is possible to form a large number of different cel- four bases: deoxyadenylic, deoxythymidylic, deoxyguanylic, lular proteins. In fact, RNA molecules transcribed from and deoxycytidylic acids. Figure 3-4 shows the chemical the same segment of DNA—the same gene—can be pro- cessed in more than one way by the cell, giving rise to alternate versions of the protein. The total number of Plasma Nuclear different proteins produced by the various cell types in membrane envelope humans is estimated to be at least 100,000. Some of the cellular proteins are structural proteins, which, in association with various lipids and carbohy- Nucleus drates, form structures of the various intracellular organ- DNA Gene (DNA) elles discussed in Chapter 2. However, most of the proteins DNA Transcription transcription are enzymes thato catalyze different chemical reactions in the cells. For example, enzymes promote all the oxidative RNA RNA formation reactions that supply energy to the cell, along with synthe- sis of all the cell chemicals, such as lipids, glycogen, and RNA splicing adenosine triphosphate (ATP). mRNA Translation Ribosomes RNA transport CELL NUCLEUS GENES CONTROL PROTEIN SYNTHESIS mRNA Protein formation In the cell nucleus, large numbers of genes are attached end Translation of mRNA Cell Cell on end in extremely long, double-stranded helical molecules structure enzymes of DNA having molecular weights measured in the billions. A very short segment of such a molecule is shown in Figure Protein Cytosol 3-2. This molecule is composed of several simple chemical Cell function compounds bound together in a regular pattern, the details Figure 3-1 The general schema whereby genes control cell function. of which are explained in the next few paragraphs. mRNA, Messenger RNA. 31 UNIT I Introduction to Physiology: The Cell and General Physiology Adenine NH2 O Thymine Guanine O H2N Cytosine P A N H T P G H N C Sugar P Sugar P Sugar NH2 O Sugar Sugar-phosphate Sugar-phosphate backbone backbone 5’ 5’ C T G T G C A T C 3’ G C A C G T A G A Base pairs Base pairs 3’ Figure 3-2 The helical double-stranded structure of the gene. The outside strands are composed of phosphoric acid and the sugar deoxyribose. The internal molecules connecting the two strands of the helix are purine and pyrimidine bases, which determine the “code” of the gene. Phosphoric acid O H O P O H O H Deoxyribose H H H O C O H H O C C C H C H H H O H Bases H H N H O N C N C H C N H C O C C C H C C H N N N C H H H H Adenine Thymine H O N H N C N C C N H H C H O C C H C C N N N H N C H H H Guanine Cytosine Purines Pyrimidines Figure 3-3 The basic building blocks of DNA. 32 Chapter 3 Genetic Control of Protein Synthesis, Cell Function, and Cell Reproduction structure of deoxyadenylic acid, and Figure 3-5 shows lines) between the purine and pyrimidine bases, the two simple symbols for the four nucleotides that form DNA.␣ respective DNA strands are held together. Note the fol- lowing caveats, however: A= T G C = Nucleotides Are Organized to Form Two 1. Each purine base adenine of one strand always bonds Strands of DNA Loosely Bound to Each with a pyrimidine base thymine of the other strand. Other 2. Each purine base guanine always bonds with a py- UNIT I Figure 3-2 shows the manner in which multiple nucleo- rimidine base cytosine. tides are bound together to form two strands of DNA. The Thus, in Figure 3-6, the sequence of complementary two strands are, in turn, loosely bonded with each other pairs of bases is CG, CG, GC, TA, CG, TA, GC, AT, and by weak cross-linkages, as illustrated in Figure 3-6 by the AT. Because of the looseness of the hydrogen bonds, the central dashed lines. Note that the backbone of each DNA two strands can pull apart with ease, and they do so many strand is composed of alternating phosphoric acid and times during the course of their function in the cell. deoxyribose molecules. In turn, purine and pyrimidine To put the DNA of Figure 3-6 into its proper physical bases are attached to the sides of the deoxyribose mol- perspective, one could merely pick up the two ends and ecules. Then, by means of loose hydrogen bonds (dashed twist them into a helix. Ten pairs of nucleotides are pres- ent in each full turn of the helix in the DNA molecule.␣ H H N Adenine N C GENETIC CODE C N H C The importance of DNA lies in its ability to control the Phosphate C C H formation of proteins in the cell, which it achieves by N N O H H O means of a genetic code. That is, when the two strands of C H Deoxyribose a DNA molecule are split apart, the purine and pyrimi- H O P O C C C H dine bases projecting to the side of each DNA strand are C O H exposed, as shown by the top strand in Figure 3-7. It is H O H H these projecting bases that form the genetic code. H The genetic code consists of successive “triplets” of bases—that is, each three successive bases is a code word. Figure 3-4. Deoxyadenylic acid, one of the nucleotides that make up DNA. The successive triplets eventually control the sequence of amino acids in a protein molecule that is to be synthe- sized in the cell. Note in Figure 3-6 that the top strand of DNA, reading from left to right, has the genetic code GGC, AGA, CTT, with the triplets being separated from A T one another by the arrows. As we follow this genetic code through Figure 3-7 and Figure 3-8, we see that these P D P D Deoxyadenylic acid Deoxythymidylic acid three respective triplets are responsible for successive placement of the three amino acids, proline, serine, and glutamic acid, in a newly formed molecule of protein.␣ TRANSCRIPTION—TRANSFER OF CELL G C NUCLEUS DNA CODE TO CYTOPLASM P D P D RNA CODE Deoxyguanylic acid Deoxycytidylic acid Because DNA is located in the cell nucleus, yet most of Figure 3-5. Symbols for the four nucleotides that combine to form DNA. Each nucleotide contains phosphoric acid (P), deoxyribose (D), the cell functions are carried out in the cytoplasm, there and one of the four nucleotide bases: adenine (A); thymine (T); gua- must be some means for DNA genes of the nucleus to nine (G); or cytosine (C). control chemical reactions of the cytoplasm. This control P D P D P D P D P D P D P D P D P D P G G C A G A C T T C C G T C T G A A P D P D P D P D P D P D P D P D P D Figure 3-6. Arrangement of deoxyribose nucleotides in a double strand of DNA. 33 UNIT I Introduction to Physiology: The Cell and General Physiology DNA strand D P D P D P D P D P D P D P D P D P G G C A G A C T T C C G U C U G A Figure 3-7. Combination of ribose nucleotides R P R P R P R P R P R P R P R P with a strand of DNA to form a molecule of RNA Triphosphate RNA molecule that carries the genetic code from the gene to the P P cytoplasm. The RNA polymerase enzyme moves P P along the DNA strand and builds the RNA mol- ecule. RNA polymerase C C G U C U G A A Figure 3-8. A portion of an RNA molecule showing three RNA codons—CCG, UCU, and GAA—that control attachment P R P R P R P R P R P R P R P R P R of the three amino acids, proline, serine, and glutamic acid, respectively, to the growing RNA chain. Proline Serine Glutamic acid is achieved through the intermediary of another type of triphosphates (shown in Figure 3-7 by the two RNA nucleo- nucleic acid, RNA, the formation of which is controlled tides to the far right during RNA chain formation). These by DNA of the nucleus. Thus, as shown in Figure 3-7, the last two phosphates are combined with the nucleotide by code is transferred to RNA in a process called transcrip- high-energy phosphate bonds derived from ATP in the cell. tion. The RNA, in turn,①diffuses from the nucleus through The result of this activation process is that large quan- ① nuclear pores into the③cytoplasmic compartment, where tities of ATP energy are made available to each of the it controls protein synthesis. nucleotides. This energy is used to promote chemical reactions that add each new RNA nucleotide at the end of RNA IS SYNTHESIZED IN THE NUCLEUS the developing RNA chain.␣ FROM A DNA TEMPLATE During RNA synthesis, the two strands of DNA separate RNA CHAIN ASSEMBLY FROM ACTIVATED temporarily; one of these strands is used as a template for NUCLEOTIDES USING THE DNA STRAND synthesis of an RNA molecule. The code triplets in the AS A TEMPLATE DNA result in the formation of complementary code trip- As shown in Figure 3-7, assembly of RNA is accomplished lets (called codons) in the RNA. These codons, in turn, under the influence of an enzyme, RNA polymerase. This will control the sequence of amino acids in a protein to be large protein enzyme has many functional properties nec- synthesized in the cell cytoplasm. essary for formation of RNA, as follows: Building Blocks of RNA. The basic building blocks of 1. In the DNA strand immediately ahead of the gene polymerase RNA are almost the same as those of DNA, except for to be transcribed is a sequence of nucleotides called RNA two differences. First, the sugar deoxyribose is not used the promoter. The RNA polymerase has an appro- + promoter in RNA formation. In its place is another sugar of slightly priate complementary structure that recognizes this different composition, ribose, which contains an extra hy- promoter and becomes attached to it, which is the I droxyl ion appended to the ribose ring structure. Second, essential step for initiating the formation of RNA. unwinding thymine is replaced by another pyrimidine, uracil.␣ 2. After the RNA polymerase attaches to the promot- ↓ er, the polymerase causes unwinding of about two · separation Formation of RNA Nucleotides. The basic building turns of the DNA helix and separation of the un- blocks of RNA form RNA nucleotides, exactly as described wound portions of the two strands. previously for DNA synthesis. Here again, four separate 3. The polymerase then moves along the DNA strand, nucleotides are used to form RNA. These nucleotides temporarily unwinding and separating the two contain the bases adenine, guanine, cytosine, and uracil. DNA strands at each stage of its movement. As it Note that these bases are the same as in DNA, except that moves along, at each stage it adds a new activated uracil in RNA replaces thymine in DNA.␣ RNA nucleotide to the end of the newly forming “Activation” of RNA Nucleotides. The next step in the RNA chain through the following steps: synthesis of RNA is “activation” of RNA nucleotides by an a. First, it causes a hydrogen bond to form between enzyme, RNA polymerase. This activation occurs by add- the end base of the DNA strand and the base of ing two extra phosphate radicals to each nucleotide to form an RNA nucleotide in the nucleoplasm. 34 Chapter 3 Genetic Control of Protein Synthesis, Cell Function, and Cell Reproduction b. Then, one at a time, the RNA polymerase breaks structures on which protein molecules are actually two of the three phosphate radicals away from each assembled. of these RNA nucleotides, liberating large amounts 6. MicroRNAs (miRNAs) are single-stranded RNA of energy from the broken high-energy phosphate molecules of 21 to 23 nucleotides that can regulate bonds. This energy is used to cause covalent linkage gene transcription and translation.␣ of the remaining phosphate on the nucleotide with MESSENGER RNA—THE CODONS UNIT I the ribose on the end of the growing RNA chain. c. When the RNA polymerase reaches the end of Messenger RNA molecules are long single RNA strands the DNA gene, it encounters a new sequence of that are suspended in the cytoplasm. These molecules are DNA nucleotides called the chain-terminating composed of several hundred to several thousand RNA sequence, which causes the polymerase and the nucleotides in unpaired strands, and they contain codons newly formed RNA chain to break away from the that are exactly complementary to the code triplets of the DNA strand. The polymerase then can be used DNA genes. Figure 3-8 shows a small segment of mRNA. again and again to form more new RNA chains. Its codons are CCG, UCU, and GAA, which are the d. As the new RNA strand is formed, its weak hy- codons for the amino acids proline, serine, and glutamic drogen bonds with the DNA template break acid. The transcription of these codons from the DNA away because the DNA has a high affinity for molecule to the RNA molecule is shown in Figure 3-7. rebonding with its own complementary DNA strand. Thus, the RNA chain is forced away from RNA Codons for the Different Amino Acids. Table 3-1 the DNA and is released into the nucleoplasm. lists the RNA codons for the 20 common amino acids Therefore, the code that is present in the DNA strand found in protein molecules. Note that most of the amino is eventually transmitted in complementary form to the acids are represented by more than one codon; also, o one RNA chain. The ribose nucleotide bases always combine codon represents the signal “start manufacturing the pro- with the deoxyribose bases in the following combinations: tein molecule,” and o three codons represent “stop manu- facturing the protein molecule.” In Table 3-1, these two DNA Base RNA Base guanine Cytosine cytosine Guanine Table 3-1 RNA Codons for Amino Acids and for Start and Stop adenine Uracil thymine adenine Amino Acid RNA Codons Alanine GCU GCC GCA GCG There Are Several Different Types of RNA. As research Arginine CGU CGC CGA CGG AGA AGG on RNA has continued to advance, many different types of RNA have been discovered. Some types of RNA are in- Asparagine AAU AAC volved in protein synthesis, whereas other types serve gene Aspartic acid GAU GAC regulatory functions or are involved in posttranscription- Cysteine UGU UGC al modification of RNA. The functions of some types of Glutamic acid GAA GAG RNA, especially those that do not appear to code for pro- Glutamine CAA CAG teins, are still mysterious. The following six types of RNA Glycine GGU GGC GGA GGG play independent and different roles in protein synthesis: 1. Precursor messenger RNA (pre-mRNA) is a large, Histidine CAU CAC immature, single strand of RNA that is processed Isoleucine AUU AUC AUA in the nucleus to form mature messenger RNA Leucine CUU CUC CUA CUG UUA UUG (mRNA). The pre-RNA includes two different types Lysine AAA AAG of segments, called introns, which are removed by Methionine AUG a process called splicing, and exons, which are re- Phenylalanine UUU UUC tained in the final mRNA. 2. Small nuclear RNA (snRNA) directs the splicing of Proline CCU CCC CCA CCG pre-mRNA to form mRNA. Serine UCU UCC UCA UCG AGC AGU 3. Messenger RNA (mRNA) carries the genetic code Threonine ACU ACC ACA ACG to the cytoplasm for controlling the type of protein Tryptophan UGG formed. Tyrosine UAU UAC 4. Transfer RNA (tRNA) transports activated amino Valine GUU GUC GUA GUG acids to the ribosomes to be used in assembling the protein molecule. Start (CI) AUG 5. Ribosomal RNA, along with about 75 different pro- Stop (CT) UAA UAG UGA teins, forms ribosomes, the physical and chemical CI, Chain-initiating; CT, chain-terminating. 35 UNIT I Introduction to Physiology: The Cell and General Physiology types of codons are designated CI for “chain-initiating” appropriate sequence of amino acids in the newly form- or “start” codon and CT for “chain-terminating” or “stop” ing protein molecule.␣ codon.␣ RIBOSOMAL RNA TRANSFER RNA—THE ANTICODONS The third type of RNA in the cell is ribosomal RNA, Another type of RNA that is essential for protein synthesis which constitutes about 60% of the ribosome. The remain- is called transfer RNA (tRNA) because it transfers amino der of the ribosome is protein, including about 75 types acids to protein molecules as the protein is being syn- of proteins that are both structural proteins and enzymes thesized. Each type of tRNA combines specifically with needed to manufacture proteins. 1 of the 20 amino acids that are to be incorporated into The ribosome is the physical structure in the cytoplasm proteins. The tRNA then acts as a carrier to transport its on which proteins are actually synthesized. However, it specific type of amino acid to the ribosomes, where pro- always functions in association with the other two types tein molecules are forming. In the ribosomes, each spe- of RNA; tRNA transports amino acids to the ribosome cific type of tRNA recognizes a particular codon on the for incorporation into the developing protein, whereas mRNA (described later) and thereby delivers the appro- mRNA provides the information necessary for sequenc- priate amino acid to the appropriate place in the chain of ing the amino acids in proper order for each specific type the newly forming protein molecule. of protein to be manufactured. Thus, the ribosome acts Transfer RNA, which contains only about 80 nucleo- as a manufacturing plant in which the protein molecules tides, is a relatively small molecule in comparison with are formed. mRNA. It is a folded chain of nucleotides with a cloverleaf appearance similar to that shown in Figure 3-9. At one Formation of Ribosomes in the Nucleolus. The DNA end of the molecule there is always an adenylic acid to genes for the formation of ribosomal RNA are located in which the transported amino acid attaches at a hydroxyl five pairs of chromosomes in the nucleus. Each of these group of the ribose in the adenylic acid. chromosomes contains many duplicates of these particu- Because the function of tRNA is to cause attachment lar genes because of the large amounts of ribosomal RNA of a specific amino acid to a forming protein chain, it is required for cellular function. essential that each type of tRNA also have specificity for As the ribosomal RNA forms, it collects in the nucleo- a particular codon in the mRNA. The specific code in the lus, a specialized structure lying adjacent to the chromo- tRNA that allows it to recognize a specific codon is again somes. When large amounts of ribosomal RNA are being a triplet of nucleotide bases and is called an anticodon. synthesized, as occurs in cells that manufacture large This anticodon is located approximately in the middle of amounts of protein, the nucleolus is a large structure, the tRNA molecule (at the bottom of the cloverleaf con- whereas in cells that synthesize little protein, the nucle- figuration shown in Figure 3-9). During formation of the olus may not even be seen. Ribosomal RNA is specially protein molecule, the anticodon bases combine loosely by processed in the nucleolus, where it binds with ribosomal hydrogen bonding with the codon bases of the mRNA. In proteins to form granular condensation products that this way, the respective amino acids are lined up one after are primordial subunits of ribosomes. These subunits are - - another along the mRNA chain, thus establishing the > then released from the nucleolus and transported through the large pores of the nuclear envelope to almost all parts earns Alanine stage of the cytoplasm. After the subunits enter the cytoplasm, Cysteine they are assembled to form mature functional ribosomes. Forming protein Therefore, proteins are formed in the cytoplasm of the Histidine Alanine cell, but not in the cell nucleus, because the nucleus does Phenylalanine not contain mature ribosomes.␣ Serine Transfer RNA Proline Start codon miRNA AND SMALL INTERFERING RNA Anticodon A fourth type of RNA in the cell is microRNA (miRNA); Codon miRNA are short (21 to 23 nucleotides) single-stranded GGG AUG GCC UGU CAU GCC UUU UCC CCC AAA CAG GAC UAU RNA fragments that regulate gene expression (Figure 3-10). The miRNAs are encoded from the transcribed Ribosome Ribosome DNA of genes, but they are not translated into pro- Messenger teins and are therefore often called noncoding RNA. The RNA movement miRNAs are processed by the cell into molecules that Figure 3-9. A messenger RNA strand is moving through two ribo- are complementary to mRNA and act to decrease gene somes. As each codon passes through, an amino acid is added to the growing protein chain, which is shown in the right-hand ribosome. expression. The generation of miRNAs involves special The transfer RNA molecule transports each specific amino acid to the processing of longer primary precursor RNAs called pri- newly forming protein. miRNAs, which are the primary transcripts of the gene. 36 Chapter 3 Genetic Control of Protein Synthesis, Cell Function, and Cell Reproduction Another type of miRNA is small interfering RNA Protein-coding gene miRNA (siRNA), also called silencing RNA or short interfering RNA. The siRNAs are short, double-stranded RNA mol- ecules, comprised of 20 to 25 nucleotides, that interfere Transcription Transcription with expression of specific genes. siRNAs generally refer of mRNA of pri-miRNA to synthetic miRNAs and can be administered to silence UNIT I pri-miRNA expression of specific genes. They are designed to avoid nuclear processing by the microprocessor complex and, Microprocessor after the siRNA enters the cytoplasm, it activates the RISC complex silencing complex, blocking the translation of mRNA. Because siRNAs can be tailored for any specific sequence Nucleus in the gene, they can be used to block translation of any pre-miRNA mRNA and therefore expression by any gene for which the nucleotide sequence is known. Researchers have pro- Cytoplasm posed that siRNAs may become useful therapeutic tools Transport of pre-miRNA into cytoplasm to silence genes that contribute to the pathophysiology of diseases.␣ Dicer TRANSLATION—FORMATION OF PROTEINS ON THE RIBOSOMES Processing of pre-miRNA into small When a molecule of mRNA comes in contact with a RNA duplexes ribosome, it travels through the ribosome, beginning at a predetermined end of the RNA molecule specified by an appropriate sequence of RNA bases called the chain- RISC initiating codon. Then, as shown in Figure 3-9, while the mRNA travels through the ribosome, a protein molecule is formed, a process called translation. Thus, the ribo- some reads the codons of the mRNA in much the same mRNA way that a tape is read as it passes through the playback RISC-miRNA head of a tape recorder. Then, when a “stop” (or “chain- complex terminating”) codon slips past the ribosome, the end of a protein molecule is signaled, and the protein molecule is freed into the cytoplasm. mRNA degradation Translational repression Polyribosomes. A single mRNA molecule can form protmiRNAsiRNA Figure 3-10. Regulation of gene expression by microRNA (miRNA). protein molecules in several ribosomes at the same time Primary miRNA (pri-miRNA), the primary transcripts of a gene pro- because the initial end of the RNA strand can pass to a cessed in the cell nucleus by the microprocessor complex, are con- verted to pre-miRNAs. These pre-miRNAs are then further processed successive ribosome as it leaves the first, as shown at the bottom left in Figure 3-9 and Figure 3-11. The protein (dicerRNA in the cytoplasm by dicer, an enzyme that helps assemble an RNA- induced silencing complex (RISC) and generates miRNAs. The miRNAs molecules are in different stages of development in each regulate gene expression by binding to the complementary region of ribosome. As a result, clusters of ribosomes frequently the RNA and repressing translation or promoting degradation of the occur, with 3 to 10 ribosomes being attached to a single messenger RNA (mRNA) before it can be translated by the ribosome. mRNA at the same time. These clusters are called polyri- bosomes. The pri-miRNAs are then processed in the cell nucleus An mRNA can cause formation of a protein molecule by the microprocessor complex to pre-miRNAs, which are in any ribosome; there is no specificity of ribosomes for 70-nucleotide, stem loop structures. These pre-miRNAs given types of protein. The ribosome is simply the physi- are then further processed in the cytoplasm by a specific cal manufacturing plant in which the chemical reactions dicer enzyme that helps assemble an RNA-induced silenc- take place.␣ ing complex (RISC) and generates miRNAs. The miRNAs regulate gene expression by binding to the Many Ribosomes Attach to the Endoplasmic complementary region of the RNA and promoting repres- Reticulum. In Chapter 2, we noted that many ribosomes sion of translation or degradation of the mRNA before it become attached to the endoplasmic reticulum. This at- can be translated by the ribosome. miRNAs are believed to tachment occurs because the initial ends of many forming play an important role in normal regulation of cell function, protein molecules have amino acid sequences that imme- and alterations in miRNA function have been associated diately attach to specific receptor sites on the endoplas- with diseases such as cancer and heart disease. mic reticulum, causing these molecules to penetrate the 37 UNIT I Introduction to Physiology: The Cell and General Physiology Messenger Ribosome RNA Small Transfer RNA subunit Figure 3-11. The physical structure of the ribosomes, as well as their functional relationship to messenger Large RNA, transfer RNA, and the endo- subunit Amino acid Endoplasmic Polypeptide plasmic reticulum during the forma- reticulum chain tion of protein molecules. reticulum wall and enter the endoplasmic reticulum ma- chemical events require energy from two additional trix. This process gives a granular appearance to the por- high-energy phosphate bonds, making a total of four tions of the reticulum where proteins are being formed high-energy bonds used for each amino acid added to the and are entering the matrix of the reticulum. protein chain. Thus, the synthesis of proteins is one of the Figure 3-11 shows the functional relationship of most energy-consuming processes of the cell.␣ mRNA to the ribosomes and the manner in which the Peptide Linkage—Combination of Amino Acids. The ribosomes attach to the membrane of the endoplasmic successive amino acids in the protein chain combine reticulum. Note the process of translation occurring in with one another according to the typical reaction. several ribosomes at the same time in response to the same strand of mRNA. Note also the newly forming poly- NH2 O H R peptide (protein) chains passing through the endoplasmic R C C OH + H N C COOH reticulum membrane into the endoplasmic matrix. It should be noted that except in glandular cells, in NH2 O H R which large amounts of protein-containing secretory ves- R C C N C COOH + H2O icles are formed, most proteins synthesized by the ribo- somes are released directly into the cytosol instead of into In this chemical reaction, a hydroxyl radical (OH−) is the endoplasmic reticulum. These proteins are enzymes removed from the COOH portion of the first amino acid, and internal structural proteins of the cell.␣ and a hydrogen (H+) of the NH2 portion of the other amino acid is removed. These combine to form water, and the Chemical Steps in Protein Synthesis. Some of the two reactive sites left on the two successive amino acids chemical events that occur in the synthesis of a protein bond with each other, resulting in a single molecule. This molecule are shown in Figure 3-12. This Fig. shows rep- process is called peptide linkage. As each additional amino resentative reactions for three separate amino acids, AA1, acid is added, an additional peptide linkage is formed.␣ AA2, and AA20. The stages of the reactions are as follows: 1. Each amino acid is activated by a chemical pro- cess in which ATP combines with the amino acid SYNTHESIS OF OTHER SUBSTANCES IN to form an adenosine monophosphate complex with THE CELL the amino acid, giving up two high-energy phos- Many thousand protein enzymes formed in the manner phate bonds in the process. just described control essentially all the other chemical 2. The activated amino acid, having an excess of ener- reactions that take place in cells. These enzymes promote gy, then combines with its specific tRNA to form an synthesis of lipids, glycogen, purines, pyrimidines, and amino acid–tRNA complex and, at the same time, hundreds of other substances. We discuss many of these releases the adenosine monophosphate. synthetic processes in relation to carbohydrate, lipid, and 3. The tRNA carrying the amino acid complex then protein metabolism in Chapters 68 through 70. These sub- comes in contact with the mRNA molecule in the stances each contribute to the various functions of the cells.␣ ribosome, where the anticodon of the tRNA attach- es temporarily to its specific codon of the mRNA, thus lining up the amino acid in the appropriate se- CONTROL OF GENE FUNCTION AND quence to form a protein molecule. BIOCHEMICAL ACTIVITY IN CELLS Then, under the influence of the enzyme peptidyl From our discussion thus far, it is clear that the genes control transferase (one of the proteins in the ribosome), peptide both the physical and chemical functions of the cells. How- bonds are formed between the successive amino acids, ever, the degree of activation of respective genes must also be thus adding progressively to the protein chain. These 38 Chapter 3 Genetic Control of Protein Synthesis, Cell Function, and Cell Reproduction Amino acid AA1 AA2 AA20 + + + ATP ATP ATP Activated amino acid AMP AA1 AMP AA2 AMP AA20 + + + tRNA1 tRNA2 tRNA20 UNIT I RNA–amino acyl complex tRNA1 AA1 tRNA2 AA2 tRNA20 AA20 + + + Messenger RNA GCC UGU AAU CAU CGU AUG GUU GCC UGU AAU CAU CGU AUG GUU Complex between tRNA, tRNA13 tRNA20 tRNA1 tRNA5 tRNA3 tRNA9 tRNA2 messenger RNA, and amino acid AA1 AA5 AA3 AA9 AA2 AA13 AA20 Figure 3-12. Chemical events in the formation of a pro- GTP GTP GTP GTP GTP GTP GTP tein molecule. AMP, Adenosine monophosphate; ATP, adenosine triphosphate; GTP, guanosine triphosphate; Protein chain AA1 AA5 AA3 AA9 AA2 AA13 AA20 tRNA, transfer RNA. controlled; otherwise, some parts of the cell might overgrow Condensed Upstream or some chemical reactions might overact until they kill the chromatin Insulator cell. Each cell has powerful internal feedback control mecha- Enhan cer nisms that keep the various functional operations of the cell in step with one another. For each gene (≈20,000–25,000 Transcription inhibitors genes in all), at least one such feedback mechanism exists. Transcription There are basically two methods whereby the biochem- factors RNA polymerase 2 ical activities in the cell are controlled: (1) genetic regula- tion, in which the degree of activation of the genes and RE RE TATA INR the formation of gene products are themselves controlled, and (2) enzyme regulation, in which the activity levels of Proximal promoter already formed enzymes in the cell are controlled. elements Basal promoter Figure 3-13. Gene transcription in eukaryotic cells. A complex ar- rangement of multiple clustered enhancer modules is interspersed with GENETIC REGULATION insulator elements, which can be located upstream or downstream of Genetic regulation, or regulation of gene expression, covers a basal promoter containing TATA box (TATA), proximal promoter ele- ments (response elements, RE), and initiator sequences (INR). the entire process from transcription of the genetic code in the nucleus to the formation of proteins in the cytoplasm. controlled by regulatory elements found in the promoter Regulation of gene expression provides all living organismsof a gene (Figure 3-13). In eukaryotes, which includes all with the ability to respond to changes in their environment. mammals, the basal promoter consists of a sequence of In animals that have many different types of cells, tissues,bases (TATAAA) called the TATA box, the binding site and organs, differential regulation of gene expression also for the TATA-binding protein and several other impor- permits the different cell types in the body to each performtant transcription factors that are collectively referred to their specialized functions. Although a cardiac myocyte as the transcription factor IID complex. In addition to the contains the same genetic code as a renal tubular epithelial transcription factor IID complex, this region is where cell, many genes are expressed in cardiac cells that are not transcription factor IIB binds to both the DNA and RNA expressed in renal tubular cells. The ultimate measure of polymerase 2 to facilitate transcription of the DNA into gene “expression” is whether (and how much) of the gene RNA. This basal promoter is found in all protein-coding products (proteins) are produced because proteins carry genes, and the polymerase must bind with this basal out cell functions specified by the genes. Regulation of gene promoter before it can begin traveling along the DNA expression can occur at any point in the pathways of tran- strand to synthesize RNA. The upstream promoter is lo- scription, RNA processing, and translation. cated farther upstream from the transcription start site and contains several binding sites for positive or negative The Promoter Controls Gene Expression. Synthesis transcription factors that can affect transcription through of cellular proteins is a complex process that starts with interactions with proteins bound to the basal promoter. transcription of DNA into RNA. Transcription of DNA is The structure and transcription factor binding sites in the 39 UNIT I Introduction to Physiology: The Cell and General Physiology upstream promoter vary from gene to gene to give rise each chromosome, the DNA is wound around small to the different expression patterns of genes in different proteins called histones, which in turn are held tissues. tightly together in a compacted state by still other Transcription of genes in eukaryotes is also influenced proteins. As long as the DNA is in this compacted by enhancers, which are regions of DNA that can bind state, it cannot function to form RNA. However, transcription factors. Enhancers can be located a great multiple control mechanisms are being discovered distance from the gene they act on or even on a differ- that can cause selected areas of chromosomes to ent chromosome. They can also be located upstream or become decompacted one part at a time, so that downstream of the gene that they regulate. Although partial RNA transcription can occur. Even then, enhancers may be located far from their target gene, they specific transcriptor factors control the actual rate may be relatively close when DNA is coiled in the nucleus. of transcription by the promoter in the chromo- It is estimated that there are more than 100,000 gene some. Thus, still higher orders of control are used enhancer sequences in the human genome. to establish proper cell function. In addition, signals In the organization of the chromosome, it is important from outside the cell, such as some of the body’s to separate active genes that are being transcribed from hormones, can activate specific chromosomal areas genes that are repressed. This separation can be challeng- and specific transcription factors, therefore control- ing because multiple genes may be located close together ling the chemical machinery for function of the cell. on the chromosome. The separation is achieved by chro- Because there are many thousands of different genes mosomal insulators. These insulators are gene sequences in each human cell, the large number of ways in which that provide a barrier so that a specific gene is isolated genetic activity can be controlled is not surprising. The against transcriptional influences from surrounding genes. gene control systems are especially important for control- Insulators can vary greatly in their DNA sequence and the ling intracellular concentrations of amino acids, amino proteins that bind to them. One way an insulator activity acid derivatives, and intermediate substrates and prod- can be modulated is by DNA methylation, which is the ucts of carbohydrate, lipid, and protein metabolism.␣ case for the mammalian insulin-like growth factor 2 (IGF- 2) gene. The mother’s allele has an insulator between the CONTROL OF INTRACELLULAR FUNCTION enhancer and promoter of the gene that allows for the BY ENZYME REGULATION binding of a transcriptional repressor. However, the pater- nal DNA sequence is methylated such that the transcrip- In addition to control of cell function by genetic regula- tional repressor cannot bind to the insulator, and the IGF-2 tion, cell activities are also controlled by intracellular gene is expressed from the paternal copy of the gene.␣ inhibitors or activators that act directly on specific intra- cellular enzymes. Thus, enzyme regulation represents Other Mechanisms for Control of Transcription by the a second category of mechanisms whereby cellular bio- Promoter. Variations in the basic mechanism for control chemical functions can be controlled. of the promoter have been discovered in the past three Enzyme Inhibition. Some chemical substances formed decades. Without giving details, let us list some of them: in the cell have direct feedback effects to inhibit the spe- 1. A promoter is frequently controlled by transcrip- cific enzyme systems that synthesize them. Almost al- tion factors located elsewhere in the genome. That is, the regulatory gene causes the formation of a ways, the synthesized product acts on the first enzyme in a sequence, rather than on the subsequent enzymes, regulatory protein that in turn acts as an activator usually binding directly with the enzyme and causing an or repressor of transcription. allosteric conformational change that inactivates it. One 2. Occasionally, many different promoters are con- can readily recognize the importance of inactivating the trolled at the same time by the same regulatory first enzyme because this prevents buildup of intermedi- protein. In some cases, the same regulatory protein ary products that are not used. functions as an activator for one promoter and as a Enzyme inhibition is another example of negative feed- repressor for another promoter. back control. It is responsible for controlling intracellular 3. Some proteins are controlled not at the starting concentrations of multiple amino acids, purines, pyrimi- point of transcription on the DNA strand but far- dines, vitamins, and other substances.␣ ther along the strand. Sometimes, the control is not even at the DNA strand itself but occurs during the Enzyme Activation. Enzymes that are normally inac- processing of the RNA molecules in the nucleus be- tive often can be activated when needed. An example of fore they are released into the cytoplasm. Control this phenomenon occurs when most of the ATP has been may also occur at the level of protein formation in depleted in a cell. In this case, a considerable amount of the cytoplasm during RNA translation by the ribo- cyclic adenosine monophosphate (cAMP) begins to be somes. formed as a breakdown product of ATP. The presence of 4. In nucleated cells, the nuclear DNA is packaged in this cAMP, in turn, immediately activates the glycogen- specific structural units, the chromosomes. Within splitting enzyme phosphorylase, liberating glucose mole- 40 Chapter 3 Genetic Control of Protein Synthesis, Cell Function, and Cell Reproduction cules that are rapidly metabolized, with their energy used Centromere Chromosome for replenishment of the ATP stores. Thus, cAMP acts as Nuclear an enzyme activator for the enzyme phosphorylase and membrane thereby helps control intracellular ATP concentration. Another interesting example of both enzyme inhibi- Nucleolus tion and enzyme activation occurs in the formation of the UNIT I purines and pyrimidines. These substances are needed by Centriole Aster the cell in approximately equal quantities for the formation A B of DNA and RNA. When purines are formed, they inhibit the enzymes that are required for formation of additional purines. However, they activate the enzymes for formation of pyrimidines. Conversely, the pyrimidines inhibit their own enzymes but activate the purine enzymes. In this way, there is continual cross-talk between the synthesizing sys- tems for these two substances, resulting in almost exactly equal amounts of the two substances in the cells at all times.␣ C D Summary. There are two principal mechanisms whereby cells control proper proportions and quantities of different cellular constituents: (1) genetic regulation; and (2) enzyme regulation. The genes can be activated or inhibited, and likewise, the enzyme systems can be activated or inhibited. These regulatory mechanisms usually function as feedback E F control systems that continually monitor the cell’s biochem- ical composition and make corrections as needed. However, on occasion, substances from outside the cell (especially some of the hormones discussed in this text) also control the intracellular biochemical reactions by activating or in- hibiting one or more of the intracellular control systems.␣ G H Figure 3-14. Stages of cell reproduction. A, B, C, Prophase. D, Pro- THE DNA–GENETIC SYSTEM CONTROLS metaphase. E, Metaphase. F, Anaphase. G, H, Telophase. CELL REPRODUCTION Cell reproduction is another example of the ubiquitous uninhibited life cycle of the cell. Therefore, different cells of role that the DNA–genetic system plays in all life pro- the body actually have life cycle periods that vary from as cesses. The genes and their regulatory mechanisms deter- little as 10 hours for highly stimulated bone marrow cells to mine cell growth characteristics and when or whether an entire lifetime of the human body for many nerve cells.␣ cells will divide to form new cells. In this way, the all- important genetic system controls each stage in the devel- Cell Reproduction Begins with Replication opment of the human, from the single-cell fertilized ovum of DNA to the whole functioning body. Thus, if there is any central The first step of cell reproduction is replication (duplica- theme to life, it is the DNA–genetic system. tion) of all DNA in the chromosomes. It is only after this replication has occurred that mitosis can take place. Life Cycle of the Cell The DNA begins to be duplicated 5 to 10 hours before The life cycle of a cell is the period from cell reproduction mitosis, and the duplication is completed in 4 to 8 hours. to the next cell reproduction. When mammalian cells are The net result is two exact replicas of all DNA. These rep- not inhibited and are reproducing as rapidly as they can, licas become the DNA in the two new daughter cells that this life cycle may be as little as 10 to 30 hours. It is termi- will be formed at mitosis. After replication of the DNA, nated by a series of distinct physical events called mito- there is another period of 1 to 2 hours before mitosis begins sis that cause division of the cell into two new daughter abruptly. Even during this period, preliminary changes that cells. The events of mitosis are shown in Figure 3-14 and will lead to the mitotic process are beginning to take place. described later. The actual stage of mitosis, however, lasts for only about 30 minutes, and thus more than 95% of the DNA Replication. DNA is replicated in much the same life cycle of even rapidly reproducing cells is represented way that RNA is transcribed from DNA, except for a few by the interval between mitosis, called interphase. important differences: Except in special conditions of rapid cellular repro- 1. Both strands of the DNA in each chromosome are duction, inhibitory factors almost always slow or stop the replicated, not just one of them. 41 UNIT I Introduction to Physiology: The Cell and General Physiology DNA Replication fork polymerase Leading strand Parent Topoisomerase DNA 5’ strands 5’ 3’ Primase RNA Okazaki DNA ligase primer fragment 3’ 5’ Helicase Parent DNA strands 3’ Newly synthesized strands DNA polymerase Lagging strand Figure 3-15. DNA replication, showing the replication fork and leading and lagging strands of DNA. 2. Both entire strands of the DNA helix are replicated Primers always bind as the starting point for DNA from end to end, rather than small portions of them, replication. as occurs in the transcription of RNA. 6. Elongation. DNA polymerases are responsible for 3. Multiple enzymes called DNA polymerase, which creating the new strand by a process called elon- is comparable to RNA polymerase, are essential for gation. Because replication proceeds in the 5′ to 3′ replicating DNA. DNA polymerase attaches to and direction on the leading strand, the newly formed moves along the DNA template strand, adding nu- strand is continuous. The lagging strand begins cleotides in the 5′ to 3′ direction. Another enzyme, replication by binding with multiple primers that DNA ligase, causes bonding of successive DNA nu- are only several bases apart. DNA polymerase then cleotides to one another, using high-energy phos- adds pieces of DNA, called Okazaki fragments, to phate bonds to energize these attachments. the strand between primers. This process of repli- 4. Replication fork formation. Before DNA can be cation is discontinuous because the newly created replicated, the double-stranded molecule must be Okazaki fragments are not yet connected. An en- “unzipped” into two single strands (Figure 3-15). zyme, DNA ligase, joins the Okazaki fragments to Because the DNA helixes in each chromosome are form a single unified strand. approximately 6 centimeters in length and have mil- 7. Termination. After the continuous and discontinu- lions of helical turns, it would be impossible for the ous strands are both formed, the enzyme exonu- two newly formed DNA helixes to uncoil from each clease removes the RNA primers from the original other were it not for some special mechanism. This strands, and the primers are replaced with appro- uncoiling is achieved by DNA helicase enzymes that priate bases. Another exonuclease “proofreads” the break the hydrogen bonding between the base pairs newly formed DNA, checking and clipping off any of the DNA, permitting the two strands to separate mismatched or unpaired residues. into a Y shape known as the replication fork, the Another enzyme, topoisomerase, can transiently break area that will be the template for replication to be- the phosphodiester bond in the backbone of the DNA gin. strand to prevent the DNA in front of the replication fork DNA is directional in both strands, signified by a 5′ from being overwound. This reaction is reversible, and the and 3′ end (see Figure 3-15). Replication progresses phosphodiester bond reforms as the topoisomerase leaves. only in the 5′ to 3′ direction. At the replication fork Once completed, the parent strand and its comple- one strand, the leading strand, is oriented in the 3′ mentary DNA strand coils into the double helix shape. to 5′ direction, toward the replication fork, while the The process of replication therefore produces two DNA lagging strand is oriented 5′ to 3′, away from the rep- molecules, each with one strand from the parent DNA lication fork. Because of their different orientations, and one new strand. For this reason, DNA replication is the two strands are replicated differently. often described as semiconservative; half of the chain is 5. Primer binding. Once the DNA strands have been part of the original DNA molecule and half is brand new.␣ separated, a short piece of RNA called an RNA primer binds to the 3′ end of the leading strand. DNA Repair, DNA “Proofreading,” and “Mutation.” Primers are generated by the enzyme DNA primase. During the hour or so between DNA replication and 42 Chapter 3 Genetic Control of Protein Synthesis, Cell Function, and Cell Reproduction the beginning of mitosis, there is a period of active re- for mitosis) at a point called the centromere located near pair and “proofreading” of the DNA strands. Wherever their center. These duplicated but still attached chromo- inappropriate DNA nucleotides have been matched up somes are called chromatids.␣ with the nucleotides of the original template strand, spe- cial enzymes cut out the defective areas and replace them CELL MITOSIS with appropriate complementary nucleotides. This repair UNIT I process, which is achieved by the same DNA polymerases The actual process whereby the cell splits into two new and DNA ligases that are used in replication, is referred to cells is called mitosis. Once each chromosome has been as DNA proofreading. replicated to form the two chromatids, mitosis follows Because of repair and proofreading, mistakes are automatically within 1 or 2 hours in many cells. rarely made in the DNA replication process. When a mis- take is made, it is called a mutation. The mutation may Mitotic Apparatus: Function of the Centrioles. One of cause formation of some abnormal protein in the cell the first events of mitosis takes place in the cytoplasm in rather than a needed protein, which may lead to abnor- or around the small structures called centrioles during the mal cellular function and sometimes even cell death. latter part of interphase. As shown in Figure 3-14, two Given that many thousands of genes exist in the human pairs of centrioles lie close to each other near one pole genome, and that the period from one human generation of the nucleus. These centrioles, like the DNA and chro- to another is about 30 years, one would expect as many as mosomes, are also replicated during interphase, usually 10 or many more mutations in the passage of the genome shortly before replication of the DNA. Each centriole is from parent to offspring. As a further protection, how- a small cylindrical body about 0.4 micrometer long and ever, each human genome is represented by two separate about 0.15 micrometer in diameter, consisting mainly of sets of chromosomes, one derived from each parent, with nine parallel tubular structures arranged in the form of a almost identical genes. Therefore, one functional gene of cylinder. The two centrioles of each pair lie at right angles each pair is almost always available to the child, despite to each other. Each pair of centrioles, along with attached mutations.␣ pericentriolar material, is called a centrosome. Shortly before mitosis takes place, the two pairs of cen- trioles begin to move apart from each other. This move- CHROMOSOMES AND THEIR REPLICATION ment is caused by polymerization of protein microtubules The DNA helixes of the nucleus are packaged in chro- growing between the respective centriole pairs and actu- mosomes. The human cell contains 46 chromosomes ally pushing them apart. At the same time, other microtu- arranged in 23 pairs. Most of the genes in the two chro- bules grow radially away from each of the centriole pairs, mosomes of each pair are identical or almost identical to forming a spiny star called the aster, in each end of the each other, so it is usually stated that the different genes cell. Some of the spines of the aster penetrate the nuclear also exist in pairs, although occasionally this is not the membrane and help separate the two sets of chromatids case. during mitosis. The complex of microtubules extending In addition to DNA, there is a large amount of pro- between the two new centriole pairs is called the spindle, tein in the chromosome, composed mainly of many small and the entire set of microtubules plus the two pairs of molecules of electropositively charged histones. The his- centrioles is called the mitotic apparatus.␣ tones are organized into vast numbers of small, bobbin- Prophase. The first stage of mitosis, called prophase, is like cores. Small segments of each DNA helix are coiled shown in Figure 3-14 A, B, and C. While the spindle is sequentially around one core after another. forming, the chromosomes of the nucleus (which in in- The histone cores play an important role in regulation terphase consist of loosely coiled strands) become con- of DNA activity because as long as the DNA is packaged densed into well-defined chromosomes.␣ tightly, it cannot function as a template for formation of RNA or replication of new DNA. Furthermore, some of Prometaphase. During the prometaphase stage (see Fig- the regulatory proteins decondense the histone packaging ure 3-14D), the growing microtubular spines of the aster of the DNA and allow small segments at a time to form fragment the nuclear envelope. At the same time, multi- RNA. ple microtubules from the aster attach to the chromatids Several nonhistone proteins are also major components at the centromeres, where the paired chromatids are still of chromosomes, functioning as chromosomal structural bound to each other. The tubules then pull one chromatid proteins and, in connection with the genetic regulatory of each pair toward one cellular pole and its partner to- machinery, as activators, inhibitors, and enzymes. ward the opposite pole.␣ Replication of the chromosomes in their entirety occurs Metaphase. During the metaphase stage (see Figure during the next few minutes after replication of the DNA 3-14E), the two asters of the mitotic apparatus are pushed helixes has been completed; the new DNA helixes collect farther apart. This pushing is believed to occur because new protein molecules as needed. The two newly formed the microtubular spines from the two asters, where they chromosomes remain attached to each other (until time interdigitate with each other to form the mitotic spindle, 43 UNIT I Introduction to Physiology: The Cell and General Physiology push each other away. Minute contractile protein mol- parts of the body. Some of these growth factors circulate ecules called “molecular motors,” which may be composed in the blood, but others originate in adjacent tissues. For of the muscle protein actin, extend between the respective example, the epithelial cells of some glands, such as the spines and, using a stepping action as in muscle, actively pancreas, fail to grow without a growth factor from the slide the spines in a reverse direction along each other. underlying connective tissue of the gland. Second, most Simultaneously, the chromatids are pulled tightly by their normal cells stop growing when they have run out of attached microtubules to the very center of the cell, lining space for growth. This phenomenon occurs when cells are up to form the equatorial plate of the mitotic spindle.␣ grown in tissue culture; the cells grow until they contact a solid object, and then growth stops. Third, cells grown in Anaphase. During the anaphase stage (see Figure tissue culture often stop growing when minute amounts 3-14 F), the two chromatids of each chromosome are of their own secretions are allowed to collect in the culture pulled apart at the centromere. All 46 pairs of chromatids medium. This mechanism, too, could provide a means for are separated, forming two separate sets of 46 daughter negative feedback control of growth. chromosomes. One of these sets is pulled toward one mi- totic aster, and the other is pulled toward the other aster, Telomeres Prevent the Degradation of Chromo- as the two respective poles of the dividing cell are pushed somes. A telomere is a region of repetitive nucleotide se- still farther apart.␣ quences located at each end of a chromatid (Figure 3-16). Telomeres serve as protective caps that prevent the chro- Telophase. In the telophase stage (see Figure 3-14G and mosome from deterioration during cell division. During H), the two sets of daughter chromosomes are pushed cell division, a short piece of “primer” RNA attaches to the completely apart. Then, the mitotic apparatus dissipates, DNA strand to start the replication. However, because the and a new nuclear membrane develops around each set of primer does not attach at the very end of the DNA strand, chromosomes. This membrane is formed from portions the copy is missing a small section of the DNA. With each of the endoplasmic reticulum that are already present in cell division, the copied DNA loses additional nucleotides the cytoplasm. Shortly thereafter, the cell pinches in two, from the telomere region. The nucleotide sequences pro- midway between the two nuclei. This pinching is caused vided by the telomeres therefore prevent the degradation by the formation of a contractile ring of microfilaments of genes near the ends of chromosomes. Without telom- composed of actin and probably myosin (the two contrac- eres, the genomes would progressively lose information tile proteins of muscle) at the juncture of the newly devel- and be truncated after each cell division. Thus, the tel- oping cells that pinches them off from each other.␣ omeres can be considered to be disposable chromosomal buffers that help maintain stability of the genes but are CONTROL OF CELL GROWTH AND CELL gradually consumed during repeated cell divisions. REPRODUCTION Some cells grow and reproduce all the time, such as the blood-forming cells of the bone marrow, the germinal layers of the skin, and the epithelium of the gut. Many Telomere other cells, however, such as smooth muscle cells, may Nonreplicating Normal DNA cell not reproduce for many years. A few cells, such as the neurons and most striated muscle cells, do not reproduce during the entire life of a person, except during the origi- nal period of fetal life. In certain tissues, an insufficiency of some types of cells causes them to grow and reproduce rapidly until appropriate numbers of these cells are again available. For example, in some young animals, seven-eighths of Cancerous cells the liver can be removed surgically, and the cells of the remaining one-eighth will grow and divide until the liver mass returns to almost normal. The same phenomenon occurs for many glandular cells and most cells of the bone Cancerous DNA marrow, subcutaneous tissue, intestinal epithelium, and almost any other tissue except highly differentiated cells Telomerase enzyme such as nerve and muscle cells. Figure 3-16. Control of cell replication by telomeres and telomerase. The mechanisms that maintain proper numbers of the The cells’ chromosomes are capped by telomeres, which, in the ab- different types of cells in the body are still poorly under- sence of telomerase activity, shorten with each cell division until the cell stops replicating. Therefore, most cells of the body cannot repli- stood. However, experiments have shown at least three cate indefinitely. In cancer cells, telomerase is activated, and telomere ways in which growth can be controlled. First, growth length is maintained so that the cells continue to replicate themselves often is controlled by growth factors that come from other uncontrollably. 44 Chapter 3 Genetic Control of Protein Synthesis, Cell Function, and Cell Reproduction Each time a cell divides, an average person loses 30 Therefore, it has become clear that differentiation to 200 base pairs from the ends of that cell’s telomeres. results not from loss of genes but from selective repres- In human blood cells, the length of telomeres ranges sion of different gene promoters. In fact, electron micro- from 8000 base pairs at birth to as low as 1500 in older graphs suggest that some segments of DNA helixes that people. Eventually, when the telomeres shorten to a criti- are wound around histone cores become so condensed cal length, the chromosomes become unstable, and the that they no longer uncoil to form RNA molecules. One UNIT I cells die. This process of telomere shortening is believed explanation for this is as follows. It has been supposed that to be an important reason for some of the physiological the cellular genome begins at a certain stage of cell differ- changes associated with aging. Telomere erosion can also entiation to produce a regulatory protein that forever after occur as a result of diseases, especially those associated represses a select group of genes. Therefore, the repressed with oxidative stress and inflammation. genes never function again. Regardless of the mechanism, In some cells, such as stem cells of the bone marrow mature human cells each produce a maximum of about or skin that must be replenished throughout life, or germ 8000 to 10,000 proteins rather than the potential 20,000 to cells in the ovaries and testes, the enzyme telomerase adds 25,000 or more that would be produced if all genes were bases to the ends of the telomeres so that many more gen- active. erations of cells can be produced. However, telomerase Embryological experiments have shown that certain activity is usually low in most cells of the body, and after cells in an embryo control differentiation of adjacent cells. many generations the descendent cells will inherit defec- For example, the primordial chordamesoderm is called tive chromosomes, become senescent, and cease dividing. the primary organizer of the embryo because it forms a This process of telomere shortening is important in regu-