EG11_Sci_Cha11 PDF - Physics Electronics - 11
Document Details
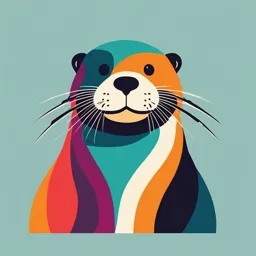
Uploaded by HeavenlyVision1945
Tags
Summary
This document covers the introduction to electronics and the properties of semiconductors, focusing on electron movement and conductivity. It also explains the difference between conductors and insulators. It also provides basic details about intrinsic semiconductors.
Full Transcript
Physics Electronics Physics Electronics 11 11.1 Introduction Electronics has made a huge...
Physics Electronics Physics Electronics 11 11.1 Introduction Electronics has made a huge impact on our daily lives. We use many electronic devices in our day to day activities. Mobile phone, computers, televisions and radios are some examples for such electronic devices. Figure 11.1 Materials that conduct electricity are known as electrical conductors. Conductors (copper, aluminium, iron, lead etc.) and mixed conductors (brass, nychrome, manganin) are examples of these. Materials that do not conduct electricity (ebonite, polythene, plastic, dry wood, asbestos, glass etc) are known as electrical insulators. The reason behind the ability to conduct electricity is the ability of some of the electrons in the atoms of such materials to move freely within the conductor. Electrons in the outer shells of conductors act in this manner since they are not tightly bound to the nucleus. Since inter-atomic bonds (covalent bonds) between the atoms of insulators are strong, there are very few electrons that are free to move. Meanwhile, some materials conduct a small amount of electricity. Such materials are known as semiconductors. Materials such as silicon (Si) and germanium (Ge) in their crystalline form show such properties. These elements belong to the fourth group in the periodic table and have four electrons in their outermost shell. Such elements form crystal lattice structures by sharing the four electrons in their outermost shell to make covalent bonds with four nearby atoms and thereby acquiring a stable electronic configuration having eight electrons in the outermost shell. However, these bonds are rather weak and can be broken from the thermal energy available even at room temperature, releasing electrons. For free distribution 50 Physics Electronics Figure 11.2 shows the covalent bonds of the silicon lattice at 0 K. All the bonds are complete at this temperature. Figure 11.3 shows that some bonds have been broken releasing some free electrons at a temperature higher than 0 K. An electron deficiency can be observed at the positions that the free electrons occupied previously. Such positions with an electron deficiency are known as holes. Due to the positively charged protons in the nucleus, a hole gives rise to a positive charge that has not been neutralized (In a neutral atom, the number of protons in the nucleus is equal to the number of and electrons). Therefore a hole is equivalent to a positive charge. si si si si si si si si si si si si free si si si si electrons si si si si si si si si holes si si si si si si si si Figure 11.2 - A silicon lattice at 0 K Figure 11.3 - A silicon lattice at temperature above 0 K In semiconductors, not only electrons contribute to the conduction of electricity. When an electron in an adjacent atom jumps to an atom with a hole having a positive charge, the position of the hole can change. By changing the position of a hole from one atom to another in this manner, holes can move around in the lattice and contribute in conducting a current. Electrons act as negative charge carriers while holes act as positive charge carriers. Therefore, when an electric potential difference is applied across a semiconductor, holes move from the positive to the negative potential while electrons move from the negative to the positive potential and the (conventional) current flows from the positive to the negative potential. ² In metallic conductors, the charge carriers that conduct electricity are the negatively charged electrons. ² In semiconductors, the negatively charged electrons as well as the positively charged holes act as the charge carriers that contribute in the conduction of electricity. ² Since a hole is generated in the breaking of a bond to release an electron, the number of carrier electrons present in a semiconductor is equal to the number of holes. ² Therefore the semiconductor lattice is electrically neutral. 51 For free distribution Physics Electronics 11.1.1 Intrinsic Semiconductors Pure semiconductor materials such as silicon (Si) and germanium (Ge) that exist in crystaline form as mentioned above are known as intrinsic semiconductors. Effect of Temperature on the Conduction of Electricity Since the random motion of free electrons increases as the temperature is increased, a rise in the temperature inhibits the current flow. Therefore, a temperature rise in conductors causes a decrease in the conductivity (increase in the resistivity). However in semiconductors, a rise in temperature breaks bonds generating more holes and free electrons causing an increase in the conductivity (decrease in the resistivity). 11.1.2 Extrinsic Semiconductors Let us consider what happens when a minute amount of the element phosphorous (P) is mixed (doped) to an intrinsic semiconductor such as Si. Phosphorous is an element in group V of the periodic table and has five electrons in the outermost shell. A phosphorous atom makes the number of electrons in its outermost shell eight by acquiring four electrons from four nearby silicon atoms around it. In the process, one of the five electrons is left behind without taking part in forming a bond. This electron has the opportunity to move about freely in the lattice. si si si si si si si si si P si si Free electron generated by doping si si si si Figure 11.4 - A Si lattice doped by phosphorous Figure 11.4 shows how a phosphorous atom forms bonds with silicon atoms. The electron left behind increases the conductivity of the lattice. Since negatively charged electrons are introduced to the lattice as charge carriers, the semiconductor is known as a negative type or n-type semiconductor. Semiconductors whose For free distribution 52 Physics Electronics carriers have been increased by doping it with another element are known as extrinsic semiconductors. By doping an intrinsic semiconductor with other elements in group V such as arsenic (As) and antimony (Sb) also, n-type extrinsic semiconductors can be formed. Since electrons are donated to the lattice by group V elements, they are known as donor atoms. If Si which is an intrinsic semiconductor is doped with an element in group III such as boron (B), the boron atom forms bonds with nearby silicon atoms. However, since there are only three electrons in the outermost shell of the boron atom, there is a deficiency of one electron in order to form four bonds. Figure 11.5 shows how the atoms and bonds are configured in this case. si si si si si si si si si B si si Hole generated by doping si si si si Figure 11.5 - A Si lattice doped by boron A hole exists at the point where the electron is deficient in the boron atom to form a bond. Since holes can conduct electricity as positive charges, the conductivity of silicon increases. As a hole is equivalent to a positive charge, such extrinsic semiconductors are known as positive or p-type semiconductors. Because the hole concentration in p - type semiconductors is much greater than the electron concentration holes are called majority carriers and electrons are called minority curriers. By doping an intrinsic semiconductor with other elements in group III such as aluminium (Al), gallium (Ga) and indium (In) instead of B also p-type extrinsic semiconductors can be formed. Since holes that can receive electrons are produced by group III elements, they are known as accepter atoms. 11.2 p – n Junction By doping one side of an intrinsic semiconductor such as silicon or germanium with a group III element to form a p-type semiconductor and the other side with a group V element to form an n-type semiconductor, a p-n junction can be formed at the centre of the semiconductor. Such a junction shows an electrical behaviour that is different from normal conductors. 53 For free distribution Physics Electronics p - type n - type Holes Free electrons (a) Diffusion Potential barrier p - region -} + n - region (b) Depletion region Figure 11.6 – p – n junction As shown in Figure 11.6(a) as soon as the p–n junction is formed, the free electrons in the n-region diffuse across the junction towards the p-region and the holes in the p-region diffuse towards the n-region. Due to this diffusion, electrons and holes recombine forming a region devoid of charges near the junction. This region is known as the depletion layer or depletion region. As shown in Figure 11.6(b), extra electrons have entered the p-side of the depletion region giving it a negative charge while extra holes have entered the n-side of the depletion region giving it a positive charge generating a voltage difference across the junction. This potential difference repels the charge carriers impeding the diffusion of charge carriers across the junction. Therefore this potential difference is known as a “potential barrier”. This potential barrier is represented in the above figure as a hypothetical battery. The magnitude of the potential barrier in a p-n junction formed by Si is about 0.7 V while that formed by Ge is about 0.3 V. For free distribution 54 Physics Electronics 11.2.1 Biasing a p-n Junction Applying a potential difference across the p-n junction using an external electric source is known as biasing. Depending on the direction of the bias voltage across the junction, it behaves in one of two ways. Let us engage in activity 11.1 to demonstrate this. Activity 11.1 Apparatus required : IN 4001 diode, A 2.5 V torch bulb, Two 1.5 V dry cell batteries, A switch, A circuit board, Connecting wires + - 3V 3V - + Forward bias Reverse bias Figure 11.7(a) Figure 11.7(b) ² Connect the circuit on the circuit board (a project board/ bread board is better for this purpose) as shown in the Figure 11.7. ² Turn on the switch and observe the bulb. ² Next, disconnect only the battery and reconnect the battery as shown in Figure 11.8. ² Turn on the switch again. Observe the bulb. Determine which of the above biasing methods allows a current to pass through the diode. You will observe that the bulb turns on only when the diode is connected as shown in Figure 11.7. According to this, you can use the junction diode when you need the current to flow only in a desired direction in a circuit. dge For extra knowle In order for the p-n junction to be forward biased and allow a current to pass through it, a positive potential should be connected to the anode and the potential difference applied should be sufficient to overcome the potential barrier across the junction. (For Si diodes this value should be greater than 0.7 V and for Ge diodes greater than 0.3 V). Reverse biased p-n junction Let us consider what happens when a battery is connected to the junction with its negative terminal connected to the p-type semiconductor and its positive terminal connected to the n-type semiconductor. 55 For free distribution Physics Electronics 0.7 V Potential barrier p-type - + n-type p-type n-type Holes electrons } } Depletion region Broadened Depletion region (a) Before biasing (b) After biasing Figure 11.8 – Reverse biased p-n junction In this case, the free electrons in the n-region are attracted towards the positive potential while the holes are attracted towards the negative potential, broadening the depletion layer. There is no carrier (charge) flow across the junction. Only the depletion region broadens depending on the potential difference of the external source. Since there is no charge flow, connecting the external potential in this manner is known as reverse bias. Figure 11.8(a) and (b) shows how the depletion layer behaves when it is reverse biased. Forward biased p-n junction 0.7 V Potential barrier depletion layer narrows down p - + n p - + n + (a) Before biased + very small current flow VB (b) VB < 0'7 V 1 1 no depletion layer p - + n + + a considerably large current flows V B 2 (c) After biased VB > 0'7 V 2 Figure 11.9 – Forward biasing of a p-n junction For free distribution 56 Physics Electronics In this case the external potential source is connected with the positive potential connected to the p-region and the negative potential connected to the n-region. While the holes in the p-region are repelled by the positive potential towards the junction, electrons in the n-region are repelled by the negative potential towards the junction. The depletion region narrows down due to this and if the externally supplied potential difference exceeds the potential barrier across the junction, carriers flow across the junction. When a high potential than 0.7 V is applied depletion layer is very small and a current is flowing through the p-n junction. Then a current flows across the junction. Therefore, connecting the external voltage in this manner is known as forward basing. 11.3 p-n Junction Diode Now you know that a current flows across a p-n junction only if it is forward biased in the manner described above. A component consisting only of such a p-n junction is known as a junction diode. The arrangement of p and n semiconductors inside a junction diode is illustrated in Figure 11.10(a) and the symbol used for a diode is shown in Figure 11.10(b). Terminal A is known as the anode and terminal K is known as the cathode. Electricity is conducted through the junction only when the anode A is connected to the positive terminal of an external voltage supply and the direction of current through the junction is shown with an arrow head in Figure 11.10(c). I p n + - anode A K cathode (a) (b) (c) Figure 11.10 – Junction diode The general outward appearance of a junction diode is shown in Figure 11.11. It has a A K cylindrical shape and a black color. The white or silver colored ring shows the terminal of the white/ silver colored ring cathode. There are large numbers of various Figure 11.11 - General outward appearance different types of diodes and a number is of a junction diode printed on the cylinder in order to identify them. But it should be remembered that the external appearance of a diode can vary widely. 11.4 Rectification of Alternating Currents We know that an alternating current is a current that alternates or changes its direction of flow in a circuit. A direct current is a current that flows only in one 57 For free distribution Physics Electronics direction. The variation of the current or the voltage with time for direct currents and alternating currents are shown in Figure 11.12. Usually, dynamos produce electricity as alternating currents. However, for operating some electronic devices, direct currents are required. Junction diodes that allow the current to flow in one direction can be used for converting an alternating current to a direct current. The task of converting an alternating current into a direct current that flows only in one direction is known as “rectification”. Voltage or current Voltage or current + + 0 time 0 time - - (b) Direct current (a) Alternating current Figure 11.12 – Graphical representation of alternating and direct currents 11.4.1 Half Wave Rectification Figure 11.13 shows a circuit used for half wave rectification. The main power supply is used to obtain the alternating current. Step-down transformer L X Current through R D 3 V AC R I 230 V AC, 50 Hz 50 Hz 0 time Y M Figure 11.13 – Half wave rectification First the voltage is lowered to a desired value using the step-down transformer. The lowered alternating potential difference is obtained from the X, Y terminals of the transformer. Since the current flow through the diode takes place only in the direction XL, the current through the resistance R flows only during the positive half cycle of the alternating current. During the negative half cycle, the current through the resistance is zero (Compare this with the way the diode behaved when the batteries were connected in activity 11.1). Since the output always consists only of half a cycle of the current, this is known as half wave rectification. For free distribution 58 Physics Electronics Exercise 11.1 Plot the current obtained through R when only the terminals of the diode in figure 11.13 are changed (so that X is connected to the cathode) while all other parts of the circuit remain intact. 11.4.2 Full Wave Rectification Activity 11.2 Apparatus required : A bicycle dynamo or an alternating current generator available in the laboratory, Four 1N 4001 diodes, A centre zero galvanometer, A 100 Ω rheostat, Lead solder and a soldering iron, Connecting wires ² Solder the four diodes in the form X of a bridge so that the anodes and 100 Ω cathodes are correctly connected. ² Connect the rheostat and the centre ~ zero galvanometer as shown in the G figure. Y ² Now connect the terminals of the bicycle dynamo or the alternating power source to the terminals X Figure 11.14 and Y and rotate the generator slowly. ² Observe the deflection of the galvanometer. If the deflection is too large adjust the rheostat to lower it. In this activity, you will observe that the deflection in the galvanometers is only in one direction. That means that the current has been converted to a direct current. If four diodes are used in the form of a bridge in an appropriate manner and an alternating current is passed through it instead of the single diode used earlier, both half cycles of the alternating current can be made to flow in the same direction. Figure 11.15 illustrates such a bridge circuit. When a 4.5 V battery and a LED bulb is connected as shown in Figure 11.15(a) the bulb lights up with its normal brightness. Here, the LED is used on a lamp that is turned on when the current is flowing in one direction only. When point X is positive relative to point Y, diodes D2 and D4 are reverse biased while diodes D1 and D3 are forward biased. Then the current flowing through D1 passes through the bulb and reaches the negative terminal of the battery after passing through diode D3. 59 For free distribution Physics Electronics X P D4 D1 + 4.5 V 2.5 V - B D3 D2 Y Q (a) X P D1 D4 - 2.5 V 4.5 V B + D3 D2 Y Q (b) Figure 11.15 - bridge circuit Now if the circuit is reconnected with the negative terminal of the battery connected to point X and the positive terminal connected to point Y as shown in Figure 11.15 (b), the bulb would light up with the same brightness. In this case, the diodes D2 and D4 are forward biased and D1 and D3 are reverse biased. Therefore the current coming from the positive terminal of the battery passes through diode D2, the bulb and diode D4 and flows to the negative terminal of the battery. In both cases, the current through the bulb flows in the same direction. dge For extra knowle In figure 11.15, a 4.5 V battery is used to light a 2.5 V bulb because the current in each case flows through two diodes producing a 2 × 0.7 = 1.4 V voltage drop. Due to this drop across the diodes, the available voltage for the bulb is 4.5 – 1.4 = 3.1 V. If a 3V battery is used instead of the 4.5 V battery, the remaining voltage of 3 - 1.4 = 1.6 V will not be sufficient to light the bulb. Now if an alternating voltage is connected to the circuit in place of the battery, the current flows through the bulb in the same direction (from P to Q). For free distribution 60 Physics Electronics X P voltage voltage + + + + + - + - t t Y - Q Figure 11.16 – Full wave rectification using a bridge circuit Figure 11.16 shows the direction of current flow through the diodes in the two half cycles, positive and negative of the input. Since both cycles of the alternating current through the bulb is made to flow in the same direction in the output, this process is known as full wave rectification. Exercise 11.2 Explain the reasons for your observations on the galvanometer in the two instances in activity 11.2 and show the variations of the current with time graphically for the two cases. 11.4.3 Smoothing A half wave or full wave rectification circuit gives out a current flowing in one direction. However its magnitude (voltage or current) varies between zero and a maximum value. Time variations of the voltage outputs from a battery, the half wave rectified output and the full wave rectified output from an alternating current are shown in Figure 11.17. For the operation of most electronic devices, a constant voltage similar to that obtained from a battery or a constant direct current is more suitable. V V V 0 0 0 time time time From a battery From half wave rectification From full wave rectification Figure 11.17 – Difference between voltage outputs from a battery and rectification 61 For free distribution Physics Electronics By connecting a capacitor with a large capacitance in parallel to the output terminals of the rectifier circuit, the variation in rectified voltage can be reduced. This process is known as smoothing. Figure 11.18 shows the output of a half wave rectified after smoothing it using a capacitor. Rectifier circuit is shown in the figure (a) and output without the capacitor is shown in (b). The figure (c) shows the output with the capacitor. D L + C R 230 V AC, 50 Hz M (a) Half wave rectification circuit current/voltage current/voltage + + 0 t 0 t - - (b) Output before smoothing (c) Output after smoothing Figure 11.18 – Smoothing of a half wave rectified When the voltage supplied by the diode gradually increases from zero, the capacitor gets charged. When the voltage drops back after reaching a maximum value, the charge stored in the capacitor is released. Therefore despite the voltage supplied through the diode being zero, the voltage across the capacitor does not drop down to zero although a small reduction takes place. In addition, because the current across the diode is always in one direction only, the discharge current of the capacitor does not flow through the diode. The time variation of the smoothed output voltage is shown in Figure 11.18(c). The output from full wave rectification too can be smoothed in the same manner. Figure 11.19 shows the circuit diagram and the time variation of the output voltage for such a rectification. + C R 230 V AC (a) Circuit diagram of full wave rectification For free distribution 62 Physics Electronics current/voltage current/voltage + + 0 t 0 t - - (b) Before smoothing (without capacitor) (c) After smoothingq (with capacitor) Figure 11.19 – Smoothing of a full wave rectification output Here the current is even smoother than in a half wave rectification. Capacitors with large capacitances such as 1000 μF, 2000 μF are used for smoothing. Smoothing becomes better with increasing capacitance. Use of a diode in order to prevent the damage caused to a direct current appliance by supplying power with inverted terminals A rectifier diode can be used to prevent the damage caused to a direct current appliance by supplying power from the positive and negative terminals incorrectly connected. current flows no current + + Electric + Electric D D device device - + - (a) (b) Figure 11.20 - Protecting a device from damage due to incorrectly connected terminals Figure 11.20(a) shows the circuit with a diode as a circuit protector and the battery connected correctly. Figure 11.20(b) shows the circuit with the battery connected incorrectly. Since the diode is reverse biased in this case there is no current passing through the device. Therefore no damage is caused to the device and it operates only if it is correctly connected to the battery. 63 For free distribution Physics Electronics dge For extra knowle Try to construct a bridge type rectifier circuit that supplies the voltage correctly to an electronic appliance regardless of how the battery is connected to the circuit. 11.4.5 Light Emitting Diode (LED) When a p-n junction formed with a semiconductor material such as gallium arsenide (GaAs) is forward biased, light is emitted at the junction. Such diodes that are capable of emitting light are known as light emitting diodes (LED). Various types of diodes are available in the market Figure 11.21 and Figure 11.22 shows the outward appearance, the symbol and how to identify the terminals of one of the most popular types, the 5 mm LED. The longer terminal of a diode is its anode. Similarly, if the base of the LED is pointed towards us, the terminal near the cut is its cathode. There are also LED’s that emit red, yellow, green, blue colors as well as infrared (IR) and ultraviolet (UV) rays in the market. Part which acts as the lens edge cut K A cut cathode (K) (c) ^shorter terminal& anode (A) (a) (longer terminal) (b) Figure 11.22 – (a) The symbol (b) Outward appearance of a light emitting diode (c) Base diagram cathode (-) is situated at the side with the cut In the early stages light emitting diodes were mostly used as indicators. At present, light emitting diodes are also used in the construction of large television screens. With the invention of white light LED’s, their use is becoming increasingly popular for lighting up homes, streets and in the construction of torches. The very low power consumption, and the long life times of about 50,000 hours are the reasons for the wide usage of light emitting diodes. For free distribution 64 Physics Electronics dge For extra knowle Minimum potential required for colour LEDs vary. Minimum potential required for some of them are given below. Current flowing through these is 10 – 20 mA. Colour Semiconductor material Minimum bias voltage Red Ga As 1.8 V Orange Ga As P 2V yellow Al In Ga P 1.8 V Green Ga P 2.2 V Blue Ga N 5V Only one colour is given by a LED. The cover is coloured to make it possible to identify its colour when it is not lighted. When the current passing through a light emitting diode is increased its brightness also increases. However, the life time decreases with brightness. 11.4.6 Solar Cells Solar cells are also constructed using p-n junctions. Therefore solar cells are also diodes. They are constructed in such a manner so as to allow light to fall on the junction. When sunlight is incident on these silicon p-n junctions, a small electro motive force (voltage) is generated across the junction. Since such a p-n junction can be used as a source of an electro motive force, they are known as solar cells. By arranging a number of such cells in series and parallel, voltages such as 12 V or 15 V with large currents can be obtained. Such an arrangement is known as a solar panel. Originally such solar panels were developed for the use satellites. They were used instead of batteries to generate electricity. Their cost at that time was very high. Since they can now be produced at a low cost with the development of technology, solar panels are used to light up homes at present. 65 For free distribution Physics Electronics Solar cell panel Main power supply Meter Figure 11.23 - A house with solar panels connected to the mains power supply Solar cells are considered to be a solution to the future power and energy crisis as they operate with the solar radiation we receive at no cost, as they do not emit any substance that is harmful to the environment and as they have a long lifetime (the solar cells produced initially are still in operation). Solar cells are presently used to operate clocks and calculators and they are also used in solar powered motor vehicles. 11.5 Transistors The transistor which is responsible for vast developments in electronics is constructed using two p-n junctions. Three semiconductor regions of type p and n are connected together to form a transistor. There are only two ways in which the three semiconductor regions can be connected in order to form two p-n junctions. Figure 11.24 shows these two ways. They are known as npn and pnp transistors p n p n p n (a) (b) Figure 11.24 – (a) structure of pnp transistor (b) structure of npn transistor For free distribution 66 Physics Electronics Three terminals, one from each of the three semiconductor regions protrude from the transistor. When the transistor is operating, carriers (electrons or holes) are emitted from one of the semiconductor regions at the two ends while the other one collects the carriers. Therefore, the two terminals at the ends are known as the emitter and the collector. The terminal at the middle can control the carriers flowing from the emitter to the collector and it is known as the base. In figures, the three first letters of the three words E, C and B are used to indicate the respective terminals. Structure of a transistor direction of carries and the direction of current are shown in the figure 11.25(a). The standard symbols used to represent transistors are given in the figure 11.25(b) C collector C collector p n Direction of Direction of the current the current B B Base flow p flow n Base Carriers Carriers p (Holes) n ^electrons & E Emitter E Emitter (a) Layout of semiconductors C C B B E E pnp transistor npn transistor (b) Standard symbols Figure 11.25 - Layout of semiconductors and symbols of transistors ♦ An arrow head is used to identify the emitter (E). ♦ The arrow head indicates the direction of current flow from the emitter to the collector of the transistor. 67 For free distribution Physics Electronics dge For extra knowle ² Carriers always flow from the emitter to the collector. ² Since the carriers of a p-type semiconductor are holes (corresponding to a + charge) the current flow in a pnp transistor is from the emitter to the collector (arrow head towards the interior). ² Since the carriers of an n-type semiconductor are electrons, the current flow in an npn transistor is from the collector to the emitter (arrow head towards the exterior). When using a transistor in a circuit, appropriate voltages must be provided to the terminals. This is known as biasing a transistor. The emitter – base junction should be forward biased while the base collector junction should be reverse biased with a higher voltage. For this a voltage should be supplied to the terminals C and E in the direction of current shown by the arrow head. Accordingly, in a npn transistor, C should be connected to the positive (+) terminal and E should the connected to the negative (–) terminal because current always flow from positive to negative. In a pnp transistor E should be connected to the positive terminal while the C should be connected to the negative (–) terminal. B should be supplied a potential difference in the same direction as C but with a smaller magnitude. Then, the base (B) - collector (C) junction becomes reverse biased. dge For extra knowle In all the circuits we discuss in the ordinary level syllabus, we use only npn transistors. There are a large number of various types of transistors in the market and they are constructed with various different outward appearances. In order to identify these transistors they are coded with numbers. Example – 2SC828 (C828), 2SD400 (D400), 2SC1061 (C1061), 2SD313 (D313) For free distribution 68 Physics Electronics dge For extra knowle There is no common or standard method of identifing the terminals of a transistor by viewing it externally. Some transistors used in the experiments of the ordinary level syllabus are shown below. D C 2S 0 2S 8 40 82 C E B C E E E B C B 2SC828 2SD400 C B (All these are silicon npn C B B E transistors). 2SD313 C E 2SC1061 As given in data books, when we turn the terminals towards ourselves and view the transistor, the terminals can be identified as shown above (two dimensional figure). 11.5.1 Amplifying Process of a Transistor ² Current Amplifier Basically a transistor is used as a current amplifier. When a small (DC) current is supplied as the input of a current amplifying transistor circuit, a large current can be obtained as the output of the amplifier. 69 For free distribution Physics Electronics Activity 11.3 Apparatus required : A 2SD400 (D400) transistor, Two 2.5 V torch bulbs, Two 3 V battery covers, six 1.5 V dry cells, Two switches (button switches are more suitable), A 10 kΩ volume controller, A circuit board ² Construct the circuit given in the figure on the circuit board. L2 ² Attach a pair of dry cells to each of the battery covers before connecting them to the circuit. Terminals of the volume C controller (variable resistance) and the L1 B + 3V transistor are indicated in the circuit. 1 1 23 2 E VR C 3 E B + 3V S1 S2 Figure 11.26 VR D400 Figure 11.27 Switch S1, the 3 V battery, the volume controller VR, and the bulb L1 are in the input circuit while the second 6 V battery, switch S2, and the bulb L2 are in the output circuit. The batteries should be correctly connected while the switches S1 and S2 are in the off positions. ² First turn S1 on (close) and adjust the resistance of VR until the bulb L1 just begins to light up. Now turn off (open) switch S1. ² Open and close the switches S1 and S2 as indicated in the table below and observe the brightness of the bulbs and fill in the table. Bulb L1 Bulb L2 S1 S2 Lighting up Brightness Lighting up Brightness off off − − on off less − off on on on (In order to clarify how to record your observations in the table, the first and second columns have been filled with the expected observations). You may assume that the For free distribution 70 Physics Electronics current flow is small if the brightness of the bulbs are low and that the current flow is large if the brightness of the bulbs are high. We can draw the following conclusions from the activity above. ² A current flows in the output circuit only when a current flows in the input circuit. ² Even when a voltage is given to the output circuit, a current does not flow in the output circuit unless there is a current flowing in the input circuit. ² When a small current flows in the input (when L1 lights up with a low brightness) a large current flows in the output (L2 lights up with a higher brightness). (The input current is known as the base current IB and the output current is known as the collector current IC). ² A small current IB in the input can be amplified into a large current IC in the output using a transistor. This is the process known as current amplification. Signal Amplifier The transistor is frequently used not only as a current amplifier but also as a signal amplifier. How a transistor can be used to amplify an audio frequency signal is illustrated in the activity 11.4. Activity 11.4 Apparatus required : A 2SD400 transistor, A 22 kΩ carbon resistor, A 8Ω speaker, A 0.1 μF capacitor, A 3 V battery cover, Two 1.5 V dry cells, A circuit board and connecting wires, Audio frequency generator (found in the laboratory) ² Construct the circuit shown in the LS figure on the circuit board. ² First connect only the AF signal 22 kΩ generator to the speaker and adjust the C output so that a sound could be just B + heard. A 0.1 μF 3V ² Connect a small signal from an audio E frequency (AF) signal generator to the points A and B. ² Now you will hear an amplified output B Figure 11.28 of the sound generated by the audio frequency generator. ² 0.1 μF capacitor has been connected to give only the alternating signal to the base. Base needs a forward biasing voltage of 0.7 V. This is supplied through the 22 kΩ resistor. 71 For free distribution Physics Electronics dge For extra knowle Apparatus required : A UM66 integrated circuit, 220 Ω carbon resistor, A 3 V battery cover, Two 1.5V dry cells, A circuit board and connecting wires A circuit that can produce a “musical” audio frequency wave using an integrated circuit is shown below. This circuit can be constructed on the circuit board to produce a signal for the audio frequency amplifier above. 220 Ω 2 3V + 1 X UM66 66 3 UM S Y 1 - signal 3 2 - positive supply 2 3 - negative supply 1 The signal can be given to the amplifier by connecting the X, Y terminals to the points A and B of the amplifier circuit. 11.5.2 Switching action of a transistor Instead of a mechanical switch, the transistor can be used as an electronic switch that operates according to a certain sensation. In electronics, when digital circuits are constructed, the transistor is often used as a switch. For free distribution 72 Physics Electronics Activity 11.5 Apparatus required : A 2SD313 transistor, A multimeter, A 2.5 V bulb, A 3 V battery cover, Two 1.5 dry cells, A 10 kΩ volume controller (VR), A 10 kΩ resistor, A circuit board, A switch (S) ² Construct the above circuit on the circuit board. Rotate the volume 10 kΩ controller to the extreme right so as to have the minimum resistance. C ² Connect batteries while keeping B + 3V switch S in the off position. 1 ² Select the multimeter range 2.5 V 2 10 kΩ + V E VR (DC) and connect it between the base 3 and emitter of the transistor. (Its S positive probe must be connected to Figure 11.29 the base.) ² Now open switch S. Observe the voltmeter reading and the lighting up and brightness of the bulb. ² Turn the volume controller gradually to the left so as to increase the resistance while observing the voltmeter reading and the bulb. ² Observe that the bulb begins to light up when the voltmeter reading is about 0.7 V and the bulb has the maximum brightness when the voltage is about 0.8 V. We can draw the following conclusions from the above activity. ² When the voltage difference between the emitter and the base is less than 0.7 V, there is no collector current Ic. ² When the voltage difference between the emitter and the base is about 0.7 V, the collector current begins to flow. ² When the voltage difference between the emitter and the base exceeds 0.7 V, (about 0.8 V), the collector current reaches a maximum. ² Therefore, when the voltage difference between B-E terminals is less than 0.7 V, the transistor acts as an open switch (off) and the voltage difference between the B-E terminals exceeds 0.7 V, it acts as a closed switch (on). 73 For free distribution Physics Electronics Let us engage in activity 11.6 to demonstrate how to design a switch circuit that automatically lights up when darkness falls using the above principle. Here we will use a light dependent resistor (LDR) as the light sensor. When light is incident on its front surface, its resistance becomes very low (of the order of 1 Ω) while in the dark it has a very high resistance (of the order of 100 kΩ). Activity 11.6 Apparatus required : A D400 transistor, An LDR, A 10 kΩ volume controller (VR), A 2.5 V bulb, A 3 V battery cover, Circuit board and connecting wires Cover the top surface of the LDR 1 (from light) with your finger tip and 10 kΩ 2 VR adjust the resistance of the VR until the bulb lights up. 3 C + ² Remove your finger tip and allow light B 3V to fall on the LDR. D 400 LDR E or D 313 Figure 11.30 Then the light will go out (Adjust the VR until the bulb lights up upon reducing the amount of incident light). For free distribution 74 Physics Electronics dge For extra knowle In the activity 11.6, the VR acts as a variable resistor and the LDR as a potential divider. (In the activity 11.5, 10 kΩ resistor and 10 kΩ variable resistor). The total potential drop across the two resistors is 3 V. V = IR from Ohm’s law +3 V 3 = I (R1 + R2) I R1 ∴I= 3 VB R1 + R2 R2 If the potential at B is VB, the potential difference across R2 is VB. I V=0 VB = R2 I 3 VB = R2 × R + R 1 2 By keeping R1 constant and varying R2, any potential from 0 to 3 V can be given to that point. If R = 10 kΩ, the value of R2 in order that V= 0.7 V: 3 × R2 0.7 = 10,000 + R2 7000 + 0.7 R2 = 3 × R2 7000 = 3 × R2 - 0.7 R2 = 2.3 R2 ∴ R2 = 7000 = 3043 Ω 2.3 Therefore when R2 is 3043 Ω, the potential at B becomes 0.7 V. When the resistance of the LDR increases upto 3043 Ω by decreasing the light incident on it, the bulb dimly lights up and when the light is further decreased, the potential increases upto 0.7 V and the current Ic increases to a maximum (Switch opens). 75 For free distribution Physics Electronics Summary The charge carriers that conduct electricity in metallic conductors are the negatively charged electrons. In semiconductors, both electrons and holes (corresponding to a positive charge) act as carriers that conduct electricity. Since a hole is formed in a semiconductor when a bond breaks releasing an electron, the number of free carrier electrons in the semiconductor is equal the number of holes in it. Therefore when a potential difference is applied across a semiconductor, holes move from the positive to the negative potential while the electrons move from the negative to the positive potential and the (standard) current flows from the positive to the negative potential. An n-type extrinsic semiconductor can be formed by doping an intrinsic semiconductor with a group V element. A p-type extrinsic semiconductor can be formed by doping an intrinsic semiconductor with a group III element. When an external potential difference is applied across a p-n junction so as to make the p region negative, the depletion region broadens and no current flows across the junction. This is known as “reverse bias”. When an external potential difference is applied across a p-n junction so as to make the p region positive, the depletion region thins down if the external potential difference is sufficiently large to mate the potential barrier, then a current flows across the junction. This is known as “forward bias”. Diodes can be used for the rectification of an alternating current. The potential barrier across a p-n junction for a Si junction is about 0.7 V and for a Ge junction about 0.3 V. Since charge carriers in a p-type semiconductor are holes (corresponding to a positive charge), in a pnp transistor, the current flows from the emitter to the collector (inward arrow head). Since charge carriers in a n-type semiconductor are electrons, in a npn transistor, the current flows from the collector to the emitter (outward arrow head). Always, carriers flow from the emitter to the collector. A transistor can be used as a simple current amplifier, signal (ac) amplifier and a switch. For free distribution 76 Physics Electronics Exercise 11.1 ^1& (i) Explain briefly, how metals and semiconductors conduct electricity. (ii) How does the increase of temperature affects the conduction of electricity? ^2& (i) A LED is not lighted up by one dry cell, but it is lighted when two dry cells are used. Explain why. (ii) Give examples for three instances where we use LEDs in day to day life. (iii) The use of LEDs which give white light, instead of filament bulls is increasing now. Give reasons for this. ^3& It has been stated in the activity, 11.6 that a transistor can be used as a switch to light a bull in the dark. A student plans to modify the above circuit to open the garage door automatically when the head lights of a car falls on it. Design a circuit for building a small model of this system for the school exhibition. Draw the circuit and Name all the components. The student hopes to use a 3 V motor to open the door. And also, indicate to which place that this motor is connected. ^4& The following figure shows a circuit made to study about the rectifier bridge in a school exhibition. All the diodes need in the circuit are 1.8V LEDs. X (i) Which LEDs are lighted – D6 D1 up, when a 6 V battery is connected to the terminal - D2 D3 X and Y? 6V + (ii) Mark the direction of D5 D4 current drawing arrows near the LEDs. Y (iii) What will happen if the Figure 11.31 battery is connected to X and Y in the opposite direction? (iv) What would happen if a 3 V battery is used to supply current instead of a 6 V battery? Give reasons for the above. 77 For free distribution Physics Electronics Technical terms Semiconductors - w¾O ikakdhl & SøÓ PhzvPÒ Intrinsic Semiconductors - ksi. w¾O ikakdhl & EÒÏmk Extrinsic Semiconductors - ndyH w¾O ikakdhl & öÁαmk Charge carriers - wdfrdamK jdyl & HØÓUPõÂPÒ Holes - l=yr & xøÍ Doping - ud;%Kh & ©õ`mhÀ Donor atom - odhl mrudKqj & uõÎ Aq Acceptor atom - m%;s.%dyl mrudKqj & HØ£õß Aq Depletion layer - ydhs; fmfoi ^ySk ia:rh& & ÁÔuõUPÀ £Sv Rectifier diode - Rcqldrl vfhdavh & ^µõUS® C¸Áõ° Bridge Rectifier - Rcqldrl fia;=j & £õ» ^µõUP® Light Emitting Diode - wdf,dal úfudapl vfhdavh & JÎPõ¾® C¸Áõ° Transistor - g%dkaisiagrh & vµõß]ØÓº Collector - ix.%dylh & ÷\P›¨£õß Emitter - úfudaplh & Põ Base - mdou & Ai Current amplifier - Odrd j¾Olh & Kmh ›¯»õUQ Signal amplifier - ix{d j¾Olh & AÔSÔ Â›¯»õUQ Forward bias - fmr keUqrej & ß P÷PõhÀ Reverse bias - miq keUqrej & ¤ß P ÷PõhÀ For free distribution 78