Module 1 - Basics of Semiconductors PDF
Document Details
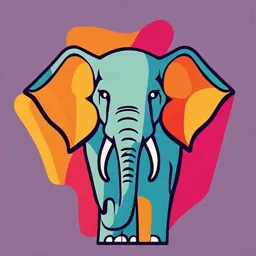
Uploaded by TopnotchTone
ICFAI University
Tags
Summary
This document provides a foundational overview of semiconductors, including their properties, atomic structure, and applications in electronics. It details the concepts of conductors and insulators, and describes different types of semiconductors. The text is well-structured and easily understandable, making the information accessible to readers at the undergraduate level.
Full Transcript
Chapter 1: Basics of Semiconductors What are conductors and non-conductors? In terms of electricity there are two main classes of material: namely: conductors and non-conductors (or insulators). From their names it can be gathered that conductors will co...
Chapter 1: Basics of Semiconductors What are conductors and non-conductors? In terms of electricity there are two main classes of material: namely: conductors and non-conductors (or insulators). From their names it can be gathered that conductors will conduct electricity freely, whereas non- conductors act as insulators preventing the flow of an electric current. An electric current is made up of the flow of electrons. This means that for a current to flow, the electrons must be able to move freely within the material. In some materials electrons are moving freely around the lattice, not particularly attached to a given electron. At any instance electrons are moving freely and randomly. By placing a potential difference across the conductor the electrons can be made to drift in one direction and this constitutes an electric current. Metals are all conductors of electricity, and a number of other substances also conduct it to varying degrees. Other substances do not have electrons moving freely around the lattice. Electrons are firmly held within their molecules and cannot escape easily. Accordingly when a potential is placed across the substance very few electrons will move and very little or no current will flow. These substances are called non-conductors or insulators. They include most plastics, ceramics and many naturally occurring substances like wood. What are Semiconductors? As the name suggests a semiconductor is neither a true conductor nor an insulator, but half way between. A number of materials exhibit this property, and they include germanium, silicon, gallium arsenide, and a variety of other substances. To understand how it acts as a semiconductor it is necessary to first look at the atomic structure of pure silicon, a good insulator. It consists of a nucleus with three rings or orbits containing electrons, each of which has a negative charge. The nucleus consists of neutrons that are neutral and have no charge, and protons that have a positive charge. In the atom there are the same number of protons and electrons so the whole atom has no overall charge. The electrons are arranged in rings with strict numbers of electrons. The first ring can only contain two, and the second has eight. The third and outer ring has four. The electrons in the outer shell are shared with those from adjacent atoms to make up a crystal lattice. When this happens there are no free electrons in the lattice, making silicon a good insulator. A similar picture can be seen for germanium. It has two electrons in the inner most orbit, eight in the next, 18 in the third, and four in the outer one. Again it shares its electrons with those from adjacent atoms to make a crystal lattice without any free electrons. Fig. 1: Atomic Structure of Silicon Fig. 2: Lattice Structure of Silicon atoms A semiconductor is a substance whose resistivity lies between the conductors and insulators. The property of resistivity is not the only one that decides a material as a semiconductor, but it has few properties as follows: Semiconductors have the resistivity which is less than insulators and more than conductors. Semiconductors have negative temperature co-efficient. The resistance in semiconductors increases with the decrease in temperature and vice versa. The Conducting properties of Semiconductor changes, when a suitable metallic impurity is added to it, which is a very important property. Semiconductor devices are extensively used in the field of electronics. The transistor has replaced the bulky vacuum tubes, from which the size and cost of the devices got decreased and this revolution has kept on increasing its pace leading to the new inventions like integrated electronics. The following (Fig. 3) illustration shows the classification of semiconductors. Fig. 3: Classification of Semiconductors Intrinsic Semiconductors A Semiconductor in its extremely pure form is said to be an intrinsic semiconductor. The properties of this pure semiconductor are as follows − The electrons and holes are solely created by thermal excitation. The number of free electrons is equal to the number of holes. The conduction capability is small at room temperature. Conduction in Semiconductors As we know that the outermost shell has the valence electrons which are loosely attached to the nucleus. Such an atom, having valence electrons when brought close to the other atom, the valence electrons of both these atoms combine to form “Electron pairs”. This bonding is not so very strong and hence it is a Covalent bond. For example, a germanium atom has 32 electrons. 2 electrons in first orbit, 8 in second orbit, 18 in third orbit, while 4 in last orbit. These 4 electrons are valence electrons of germanium atom. These electrons tend to combine with valence electrons of adjoining atoms, to form the electron pairs, as shown in figure 4. Fig. 4: Covalent bond in Germanium Fig. 5: Creation of Holes in Ge Lattice Creation of Hole Due to the thermal energy supplied to the crystal, some electrons tend to move out of their place and break the covalent bonds. These broken covalent bonds result in free electrons which wander randomly. But the moved away electrons create an empty space or valence behind, which is called as a hole. This hole which represents a missing electron can be considered as a unit positive charge while the electron is considered as a unit negative charge. The liberated electrons move randomly but when some external electric field is applied, these electrons move in opposite direction to the applied field. But the holes created due to absence of electrons, move in the direction of applied field. Hole Current It is already understood that when a covalent bond is broken, a hole is created. Actually, there is a strong tendency of semiconductor crystal to form a covalent bond. So, a hole doesn’t tend to exist in a crystal. This can be better understood by figure 5, showing a semiconductor crystal lattice. An electron, when gets shifted from a place A, a hole is formed. Due to the tendency for the formation of covalent bond, an electron from B gets shifted to A. Now, again to balance the covalent bond at B, an electron gets shifted from C to B. This continues to build a path. This movement of hole in the absence of an applied field is random. But when electric field is applied, the hole drifts along the applied field, which constitutes the hole current. This is called as hole current but not electron current because, the movement of holes contribute the current flow. Electrons and holes while in random motion, may encounter with each other, to form pairs. This recombination results in the release of heat, which breaks another covalent bond. When the temperature increases, the rate of generation of electrons and holes increase, thus rate of recombination increases, which results in the increase of densities of electrons and holes. As a result, conductivity of semiconductor increases and resistivity decreases, which means the negative temperature coefficient. Generation and recombination of electron hole pairs in semiconductors Semiconductors are characterized by two types of mobile carriers, electrons in the conduction band and holes in the valence band. Both bands are separated by the energy gap. This energy gap is about 1.1 eV in silicon. There is a continuous transition of electrons between the two bands. When an electron falls from the conduction band into the valence band, into a hole, a recombination process occurs and an electron hole pair disappears. The energy of recombination will be emerged as a photon of light. Inversely, when a valence electron is given energy equal or greater than the energy gap it will be transferred to the conduction band and an electron hole pair will be generated. There are different types of e-h generation: the thermal generation, the photo generation, the impact ionization according to the energy supplied in the process. The recombination is always present and sets up an equilibrium in steady state. Recombination rate is controlled by the minority carrier lifetime Doping In order to increase the conduction capability of intrinsic semiconductor, it is better to add some impurities. This process of adding impurities is called as Doping. Now, this doped semiconductor is called as an Extrinsic Semiconductor. The impurities added, are generally pentavalent and trivalent impurities. Pentavalent Impurities The pentavalent impurities are the ones which have five valence electrons in the outer most orbit. Example: Bismuth, Antimony, Arsenic, Phosphorus The pentavalent atom is called as a donor atom because it donates one electron to the conduction band of pure semiconductor atom. Trivalent Impurities The trivalent impurities are the ones which has three valence electrons in the outer most orbit. Example: Gallium, Indium, Aluminum, Boron The trivalent atom is called as an acceptor atom because it accepts one electron from the semiconductor atom. Extrinsic Semiconductor An impure semiconductor, which is formed by doping a pure semiconductor, is called as an extrinsic semiconductor. There are two types of extrinsic semiconductors depending upon the type of impurity added. They are N-type extrinsic semiconductor and P-Type extrinsic semiconductor. N-Type Extrinsic Semiconductor A small amount of pentavalent impurity is added to a pure semiconductor to result in Ntype extrinsic semiconductor. The added impurity has 5 valence electrons. For example, if Arsenic atom is added to the germanium atom, four of the valence electrons get attached with the Ge atoms while one electron remains as a free electron. This is as shown in figure 6. Fig. 6: Formation of n-type Semiconductor Fig. 7: Formation of p-type Semiconductor All of these free electrons constitute electron current. Hence, the impurity when added to pure semiconductor provides electrons for conduction. ۞ In N-type extrinsic semiconductor, as the conduction takes place through electrons, the electrons are majority carriers and the holes are minority carriers. ۞ As there is no addition of positive or negative charges, the electrons are electrically neutral. ۞ When an electric field is applied to an N-type semiconductor, to which a pentavalent impurity is added, the free electrons travel towards positive electrode. This is called as negative or N-type conductivity. P-Type Extrinsic Semiconductor A small amount of trivalent impurity is added to a pure semiconductor to result in P-type extrinsic semiconductor. The added impurity has 3 valence electrons. For example, if Boron atom is added to the germanium atom, three of the valence electrons get attached with the Ge atoms, to form three covalent bonds. But, one more electron in germanium remains without forming any bond. As there is no electron in boron remaining to form a covalent bond, the space is treated as a hole. This is as shown in the following figure. The boron impurity when added in a small amount provides a number of holes which helps in the conduction. All of these holes constitute hole current. ۞ In P-type extrinsic semiconductor, as the conduction takes place through holes, the holes are majority carriers while the electrons are minority carriers. ۞ The impurity added here provides holes which are called as acceptors, because they accept electrons from the germanium atoms. ۞ As the number of mobile holes remains equal to the number of acceptors, the Ptype semiconductor remains electrically neutral. ۞ When an electric field is applied to a P-type semiconductor, to which a trivalent impurity is added, the holes travel towards negative electrode, but with a slow pace than electrons. This is called as P-type conductivity. ۞ In this P-type conductivity, the valence electrons move from one covalent bond to another, unlike N-type. Why Silicon is Preferred in Semiconductors? Among the semiconductor materials like germanium and silicon, the extensively used material for manufacturing various electronic components is Silicon (Si). Silicon is preferred over germanium for many reasons such as − ۞ The energy band gap is 0.7ev, whereas it is 0.2ev for germanium. ۞ The thermal pair generation is smaller. ۞ The formation of SiO2 layer is easy for silicon, which helps in the manufacture of many components along with integration technology. ۞ Si is easily found in nature than Ge. ۞ Noise is less in components made up of Si than in Ge. Hence, Silicon is used in the manufacture of many electronic components, which are used to make different circuits for various purposes. These components have individual properties and particular uses. The main electronic components include — Resistors, variable resistors, Capacitors, variable capacitors, Inductors, diodes, Tunnel diodes, Varactor diodes, Transistors, BJTs, UJTs, FETs, MOSFETs, LDR, LED, Solar cells, Thermistor, Varistor, Transformer, switches, relays, etc. Comparison between P Type and N Type Semiconductor Parameters P Type N Type Type Extrinsic semiconductor Extrinsic semiconductor A type of extrinsic semiconductor that A type of extrinsic semiconductor that carries Description carries positive charge and has improved negative charge and has improved conductivity conductivity Hole concentration Larger hole concentration Less hole concentration Electron concentration Less electron concentration Larger electron concentration Charge Positive charge of the hole Negative charge of the electron Holes are the majority carriers and Electrons are the majority carriers and holes Carriers electrons are the minority carriers are the minority carriers Created by doping an intrinsic Created by doping an intrinsic semiconductor Creation semiconductor with acceptor impurities with donor impurities Common p-type dopant is boron. Others Common dopant for n-type is phosphorus. Dopant include aluminum or gallium. Others include antimony and arsenic. Effect of doping Acceptor impurity creates a hole. Donor impurities contributes free electrons Contains Trivalent impurities Pentavalent impurities Fermi level is below the intrinsic Fermi Fermi level is greater than that of the intrinsic Fermi level level and lies closer to the valence band semiconductor and lies closer to the than the conduction band conduction band than the valence band p–n junction A p–n junction is a boundary or interface between two types of semiconductor material, p-type and n-type, inside a single crystal of semiconductor. The "p" (positive) side contains an excess of holes, while the "n" (negative) side contains an excess of electrons. p–n junctions are elementary "building blocks" of most semiconductor electronic devices such as diodes, transistors, solar cells, LEDs, and integrated circuits; they are the active sites where the electronic action of the device takes place. For example, a common type of transistor, the bipolar junction transistor, consists of two p–n junctions in series, in the form n–p–n or p–n–p. The p–n junction possesses some interesting properties that have useful applications in modern electronics. A p- doped semiconductor is relatively conductive. The same is true of an n-doped semiconductor, but the junction between them can become depleted of charge carriers, and hence non-conductive, depending on the relative voltages of the two semiconductor regions. By manipulating this non-conductive layer, p–n junctions are commonly used as diodes: circuit elements that allow a flow of electricity in one direction but not in the other (opposite) direction. Bias is the application of a voltage across a p–n junction; forward bias is in the direction of easy current flow, and reverse bias is in the direction of little or no current flow. Equilibrium (zero bias) In a p–n junction, without an external applied voltage, an equilibrium condition is reached in which a potential difference is formed across the junction. This potential difference is called built-in potential. After joining p-type and n-type semiconductors, electrons from the n region near the p–n interface tend to diffuse into the p region leaving behind positively charged ions in the n region and being recombined with holes, forming negatively charged ions in the p region. Likewise, holes from the p-type region near the p–n interface begin to diffuse into the n-type region, leaving behind negatively charged ions in the p region and recombining with electrons, forming positive ions in the n region. The regions near the p–n interface lose their neutrality and most of their mobile carriers, forming the space charge region or depletion layer. The electric field created by the space charge region opposes the diffusion process for both electrons and holes. There are two concurrent phenomena: the diffusion process that tends to generate more space charge; and the electric field generated by the space charge that tends to counteract the diffusion. The carrier concentration profile at equilibrium is shown in figure 8. Also shown are the two counter-balancing phenomena that establish equilibrium. Fig. 8: p–n junction in thermal equilibrium Fig. 9: Movement of holes and electrons Drift and diffusion currents The net current that flows through a (PN junction diode) semiconductor material has two components Drift current Diffusion current Drift current When an electric field is applied across the semiconductor material, the charge carriers attain a certain drift velocity Vd , which is given by; Drift velocity Vd = mobility of the charge carriers X Applied Electric field intensity E Holes move towards the negative terminal of the battery and electrons move towards the positive terminal of the battery as shown in figure 9. This combined effect of movement of the charge carriers constitutes a current known as “drift current”. Thus the drift current is defined as the flow of electric current due to the motion of the charge carriers under the influence of an external electric field. Drift current due to the charge carriers such as free electrons and holes are the current passing through a square centimeter perpendicular to the direction of flow. Drift current density Jn , due to free electrons is given by Jn = q n μn E (A / cm2) Drift current density JP, due to holes is given by JP = q p μp E (A / cm2) Where, n - Number of free electrons per cubic centimeter. P - Number of holes per cubic centimeter μn - Mobility of electrons in cm2 / Vs μp - Mobility of holes in cm2 / Vs E - Applied Electric filed Intensity in V /cm q - Charge of an electron = 1.6 x 10-19 coulomb. Diffusion current It is possible for an electric current to flow in a semiconductor even in the absence of the applied voltage provided a concentration gradient exists in the material. A concentration gradient exists if the number of either elements or holes is greater in one region of a semiconductor as compared to the rest of the Region. In a semiconductor material the change carriers have the tendency to move from the region of higher concentration to that of lower concentration of the same type of charge carriers. Thus the movement of charge carriers takes place resulting in a current called diffusion current.