Course Package in Bridge Design - Chapter 1 PDF
Document Details
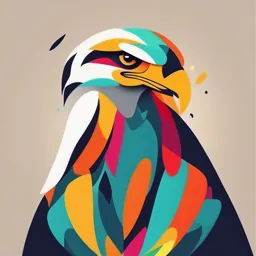
Uploaded by TranquilHawkSEye
Cebu Technological University
Tags
Related
- Bridge Engineering Module 1 PDF
- CETS463/464 Bridge Engineering PDF
- Bridge Construction Methods and Equipment PDF
- EGB123 Bridge Approaches & Abutments 2024 QUT PDF
- CIVL3811 Introduction to Bridge Engineering (Part 1) Lecture Slides - Week 6 2022 PDF
- CIVL3811 Bridge Engineering Lecture Slides (Week 7) 2022 PDF
Summary
This document provides an introduction to bridge design, covering the evolution of bridge design from ancient times to modern constructions. It examines the different types of bridges throughout history, focusing on ancient examples, Roman arches, and modern materials.
Full Transcript
1 Chapter 1 Introduction to Bridge Design Presentation / Discussion: History and Evolution of Bridge Design - The history and evolution of b...
1 Chapter 1 Introduction to Bridge Design Presentation / Discussion: History and Evolution of Bridge Design - The history and evolution of bridge design are a testament to human ingenuity, technological advancement, and the continuous quest for connectivity. - Bridges have played a crucial role in the development of civilizations by facilitating the movement of people, goods, and ideas across natural and man-made obstacles. - Over the millennia, bridge design has evolved from simple, primitive structures to complex engineering marvels that span vast distances and withstand immense forces. - This explores the historical progression of bridge design, from ancient times to the modern era, highlighting key developments, materials, and techniques. 1. Ancient Bridges and Historical Examples The earliest bridges were simple structures built using natural materials such as wood, stone, and rope. These primitive bridges were primarily functional, providing basic crossings over rivers, streams, and valleys. a. Earliest Bridges Log Bridges: The simplest form of bridge was likely the log bridge, created by placing a tree trunk across a stream or gap. These early bridges were straightforward, but they were limited in span and durability due to the natural properties of wood. Clapper Bridges: In regions where stone was abundant, early bridge builders used flat stones laid across supports to create clapper bridges. These structures, which date back thousands of years, can still be found in parts of Europe, such as the Tarr Steps in England. Rope Bridges: Indigenous cultures, particularly in mountainous regions like the Andes, developed rope bridges made from natural fibers. The Inca rope bridges in Peru are famous examples, showcasing the use of tensile materials to create flexible, yet functional, crossing points. b. Roman Arch Bridges The Romans were pioneers in the development of durable and aesthetically pleasing bridges. They introduced the use of arches, which became a defining feature of bridge design for centuries. The Arch as a Structural Element: The Roman arch was a key innovation in bridge design. By using a curved structure, the Romans were able to distribute loads more efficiently, allowing for longer spans and greater durability. The arch bridge works by transferring the load from the center of the bridge (keystone) to the abutments on either side, which then transmit the load into the ground. Notable Roman Bridges: o Pont du Gard (France): A Roman aqueduct bridge that exemplifies the use of stone arches in large-scale infrastructure. Built in the 1st century AD, it features three tiers of arches and was used to transport water across the Gardon River. o Alcántara Bridge (Spain): Another iconic Roman bridge, completed around 106 AD. It spans the Tagus River with a series of stone arches, demonstrating the Romans' mastery of masonry and engineering. c. Medieval and Renaissance Bridges During the Middle Ages and the Renaissance, bridge design saw improvements in both functionality and aesthetics. Bridges began to serve not only as transportation routes but also as centers of commerce and social activity. 2 o Stone Arch Bridges: The use of stone arches continued to dominate bridge construction during this period. Bridges like the Ponte Vecchio in Florence, Italy (built in 1345), and the Rialto Bridge in Venice (completed in 1591) are prime examples of how bridges became integral parts of urban infrastructure, combining utility with artistic expression. o Covered Bridges: In Europe and later in North America, covered bridges became popular. These timber structures were covered with roofs to protect the wooden trusses from the elements, thereby extending their lifespan. The Kapellbrücke in Lucerne, Switzerland, built in 1333, is one of the oldest surviving covered bridges. 2. The Industrial Revolution and the Advent of Metal Bridges The Industrial Revolution in the 18th and 19th centuries marked a significant turning point in bridge design. The introduction of new materials, particularly iron and steel, and advances in construction techniques allowed for the creation of longer, stronger, and more complex bridges. a. Iron Bridges The Iron Bridge (England): The world's first cast iron bridge, completed in 1779, spans the River Severn in Shropshire, England. Designed by Abraham Darby III, the Iron Bridge demonstrated the potential of iron as a bridge- building material. Its success paved the way for the widespread use of iron in bridge construction. Truss Bridges: The truss bridge design became popular during the Industrial Revolution. The truss, a framework of triangular units, provided excellent load distribution and allowed for longer spans. Iron trusses were particularly useful in railway bridges, where the need for strong, durable structures was paramount. b. Steel Bridges Advantages of Steel: Steel, with its superior strength-to-weight ratio compared to iron, quickly became the material of choice for bridge construction. Steel's ductility and tensile strength allowed for innovative designs, including longer spans and lighter structures. Notable Steel Bridges: o Brooklyn Bridge (USA): Completed in 1883, the Brooklyn Bridge in New York City was one of the first steel-wire suspension bridges. Designed by John A. Roebling, it became an iconic symbol of engineering prowess, with its steel cables and gothic-style stone towers. o Forth Bridge (Scotland): Completed in 1890, the Forth Bridge is a cantilever railway bridge that spans the Firth of Forth in Scotland. Its use of steel in a cantilever design allowed for a span of over 518.16 meters making it one of the longest and most impressive bridges of its time. 3. The 20th Century: Concrete and the Modern Era The 20th century saw the widespread adoption of concrete as a primary material in bridge construction, as well as significant advancements in design and construction techniques. a. Concrete Bridges Reinforced Concrete: The introduction of reinforced concrete, which uses steel bars embedded within the concrete to provide tensile strength, revolutionized bridge design. Reinforced concrete bridges could be molded into various shapes, allowing for more creative and efficient designs. Prestressed Concrete: Prestressed concrete involves placing the concrete under compression before applying the load. This technique, developed in the early 20th century, further improved the strength and durability of concrete bridges, enabling longer spans and thinner bridge decks. Notable Concrete Bridges: 3 o Sydney Harbour Bridge (Australia): Completed in 1932, this iconic arch bridge is made of steel but incorporates extensive use of reinforced concrete in its approach spans and pylons. It remains one of the most recognizable bridges in the world. o Glen Canyon Dam Bridge (USA): Completed in 1959, this concrete arch bridge spans the Colorado River in Arizona. It demonstrates the capability of concrete in constructing long-span arch bridges. b. Modern Bridge Types and Innovations Bridge design has evolved significantly over the centuries, but the pace of innovation in recent decades has accelerated due to advances in materials science, engineering techniques, and technology. These innovations have enabled the construction of more durable, efficient, and aesthetically pleasing bridges while addressing challenges such as sustainability, safety, and maintenance. Modern innovations in bridge design: 1. Advanced Materials a. High-Performance Concrete (HPC) and Ultra-High-Performance Concrete (UHPC): o Description: High-Performance Concrete (HPC) and Ultra-High- Performance Concrete (UHPC) represent significant advancements in concrete technology. HPC has enhanced durability, strength, and longevity compared to conventional concrete, while UHPC is characterized by its exceptionally high compressive strength and durability, often incorporating fibers for additional reinforcement. o Applications: These materials are used in bridge decks, piers, and beams to reduce maintenance needs and extend the lifespan of bridges. UHPC is particularly useful in reducing the size of structural elements, allowing for more slender and elegant designs. o Example: The Mars Hill Bridge in Iowa, USA, uses UHPC to achieve a thinner deck while maintaining structural integrity. b. Fiber-Reinforced Polymers (FRP): o Description: FRP materials are composites made from a polymer matrix reinforced with fibers, typically glass, carbon, or aramid. These materials are lightweight, have high tensile strength, and are resistant to corrosion. o Applications: FRP is used in bridge components like decks, reinforcing bars, and cables. It is especially beneficial in corrosive environments, such as coastal regions or locations with heavy use of de-icing salts. o Example: The Broadway Bridge in Kansas City, Missouri, USA, uses FRP for its deck panels, offering reduced weight and increased durability. c. Self-Healing Materials: o Description: Self-healing materials have the ability to repair cracks and damage autonomously, without the need for external intervention. This innovation often involves microcapsules containing healing agents or bacteria that activate when cracks form. o Applications: Used in concrete to extend the lifespan of bridge structures by reducing maintenance and preventing the propagation of cracks. o Example: Experimental projects in the Netherlands and the UK have tested self-healing concrete in bridges to reduce the need for repairs. 2. Innovative Structural Designs a. Cable-Stayed Bridges with Fan and Harp Designs: o Description: Cable-stayed bridges have become increasingly popular due to their aesthetic appeal and structural efficiency. Innovations in cable arrangement, such as the fan and harp designs, allow for better load distribution and more striking visual effects. 4 o Applications: These designs are used in bridges that require long spans but need to maintain a slim, elegant profile. o Example: The Millau Viaduct in France, one of the tallest bridges in the world, uses a cable-stayed design with a fan arrangement to achieve its remarkable span and height. b. Extradosed Bridges: o Description: Extradosed bridges are a hybrid between cable-stayed and girder bridges. They feature a lower tower height compared to traditional cable-stayed bridges, with the cables functioning more like external tendons. o Applications: These bridges are suitable for medium spans and are often used where aesthetic considerations and cost savings are important. o Example: The Pont de Normandie in France is one of the most well- known extradosed bridges. c. Floating Bridges: o Description: Floating bridges, or pontoon bridges, rest on the surface of a body of water, supported by buoyant structures. Modern innovations have improved their stability and durability, making them viable for permanent installations. o Applications: Used in locations with deep water or soft soil where traditional bridge foundations would be difficult or expensive to construct. o Example: The Evergreen Point Floating Bridge in Washington, USA, is the world’s longest floating bridge, designed to withstand harsh weather and heavy traffic. 3. Smart Bridges and Monitoring Systems a. Structural Health Monitoring (SHM) Systems: o Description: SHM systems use a network of sensors embedded in the bridge structure to continuously monitor its health. These sensors can detect strain, vibrations, temperature changes, and other factors that could indicate potential structural issues. o Applications: SHM systems are used to provide real-time data on the condition of bridges, allowing for proactive maintenance and reducing the risk of catastrophic failures. o Example: The Queensferry Crossing in Scotland is equipped with an extensive SHM system, including over 2,000 sensors that monitor the bridge's health and performance. b. Drones and UAVs for Inspection: o Description: Unmanned Aerial Vehicles (UAVs) or drones are increasingly used for bridge inspections, allowing engineers to assess the condition of hard-to-reach areas safely and efficiently. o Applications: Drones are used for routine inspections, post-disaster assessments, and detailed surveys, significantly reducing the time and cost associated with traditional inspection methods. o Example: Drones are regularly used to inspect the Golden Gate Bridge, providing high-resolution images and data to engineers without disrupting traffic. c. Adaptive Structures and Responsive Design: o Description: Adaptive bridges use smart materials and actuators to respond to changing loads and environmental conditions. These bridges can alter their structural behavior in real-time to optimize performance. o Applications: These innovations are used in bridges located in areas prone to earthquakes, high winds, or variable traffic loads. o Example: The Kurilpa Bridge in Brisbane, Australia, incorporates an adaptive structure with a dynamic lighting system that responds to environmental conditions. 5 4. Sustainability in Bridge Design a. Green Bridges and Eco-Viaducts: o Description: Green bridges, also known as wildlife overpasses or eco- viaducts, are designed to allow wildlife to safely cross over highways or railways. These bridges are often covered with vegetation and are integrated into the surrounding landscape. o Applications: Used in areas where infrastructure intersects with natural habitats, helping to reduce wildlife-vehicle collisions and preserve biodiversity. o Example: The Banff Wildlife Overpass in Canada is a green bridge that helps animals cross over the Trans-Canada Highway. b. Recycling and Reusing Materials: o Description: The use of recycled materials in bridge construction, such as recycled steel, concrete, and plastics, reduces the environmental impact of new bridges. This practice aligns with sustainability goals and reduces the carbon footprint of construction projects. o Applications: Recycled materials are used in bridge components such as decks, railings, and barriers. o Example: The Recycled Plastic Bridge in Peeblesshire, Scotland, is made entirely from recycled plastic materials, demonstrating the potential for sustainable construction. c. Low-Carbon Construction Techniques: o Description: Low-carbon construction techniques focus on reducing greenhouse gas emissions during the construction process. This includes the use of low-carbon concrete, energy-efficient construction methods, and minimizing waste. o Applications: These techniques are increasingly mandated in public infrastructure projects to meet environmental regulations and sustainability targets. o Example: The construction of the Queensferry Crossing in Scotland incorporated low-carbon techniques to reduce the environmental impact of the project. 5. Architectural Innovations and Aesthetic Considerations a. Iconic Design and Landmarks: o Description: Modern bridge design often goes beyond functionality, with architects and engineers creating structures that become iconic landmarks. These designs incorporate artistic elements and innovative forms that enhance the visual appeal of the bridge and its surroundings. o Applications: Iconic bridges are often located in urban areas or significant cultural sites, where they contribute to the city's identity and tourism. o Example: The Helix Bridge in Singapore is a pedestrian bridge with a unique double-helix structure inspired by DNA, making it an architectural and engineering marvel. b. Integration with Urban Spaces: o Description: Modern bridge design increasingly focuses on integrating bridges with urban spaces, creating multifunctional structures that serve as public spaces, parks, or cultural venues in addition to their primary function of transportation. o Applications: These designs are often seen in pedestrian bridges or viaducts that span over urban areas, providing green spaces and gathering places for the community. o Example: The High Line in New York City is a repurposed railway viaduct that has been transformed into an elevated park, blending infrastructure with urban greenery. 6 4. The Future of Bridge Design As we move further into the 21st century, bridge design continues to evolve, driven by advancements in technology, materials, and environmental considerations. a. Smart Bridges The integration of smart technologies into bridge design allows for real-time monitoring of structural health. Sensors embedded in the bridge can monitor stress, strain, temperature, and other factors, providing data that can be used to ensure the bridge’s safety and extend its lifespan. b. Modular and Prefabricated Construction Modular construction techniques, where large sections of the bridge are prefabricated off-site and then assembled on-site, have become increasingly popular. This method reduces construction time, minimizes disruption, and improves quality control. c. Resilience and Adaptability Modern bridge design increasingly focuses on resilience, ensuring that bridges can withstand extreme events such as earthquakes, hurricanes, and floods. Adaptable designs that allow for easy maintenance, upgrades, and retrofitting are also becoming more common, ensuring that bridges remain safe and functional throughout their service lives. Bridge Classification - The classification of bridges is a fundamental aspect of civil engineering that provides a structured framework for understanding the diverse range of bridge types, each designed to meet specific functional, environmental, and aesthetic requirements. - The classification system for bridges is not merely a matter of categorization; it reflects the complex interplay between engineering principles, material science, environmental constraints, and socio-economic considerations. 1. Classification by Structural Form Bridges can first and foremost be classified based on their structural form, which directly relates to how they manage and distribute loads. The primary categories include: Beam Bridges o Structural Form: Beam bridges are the simplest form of bridge structure, where the load is carried by a horizontal beam that rests on supports at either end. The beam must resist bending forces caused by the weight of the load on the bridge. o Load Distribution: The load on a beam bridge is transferred directly to the supports (abutments or piers) through vertical forces. The forces generate bending moments and shear forces within the beam, requiring the beam to be strong in both tension and compression. o Materials: Historically, timber was commonly used for beam bridges, but modern versions typically use steel, reinforced concrete, or pre-stressed concrete due to their superior strength and durability. o Span: Beam bridges are generally used for short to medium spans, typically up to 250 meters. Longer spans are possible but require additional support piers. o Advantages: Simple design, relatively easy to construct, and cost-effective for short spans. o Disadvantages: Limited span length, as the bending forces become excessive for longer spans. o Examples: The Lake Pontchartrain Causeway in Louisiana, USA, and many overpasses and highway bridges around the world. 7 Arch Bridges o Structural Form: Arch bridges use a curved arch as the primary structural element. The arch shape naturally transfers the load to the supports (known as abutments) at either end of the bridge, which bear the horizontal thrust exerted by the arch. o Load Distribution: The load on an arch bridge is transferred as compressive forces along the curve of the arch, pushing outward toward the abutments. This compressive force makes arch bridges inherently strong and capable of supporting significant loads. o Materials: Stone was traditionally used for arch bridges, particularly in ancient and medieval times. Modern arch bridges often use steel, reinforced concrete, or a combination of materials. o Span: Arch bridges are suitable for medium spans, typically ranging from 100 to 500 meters. The design can be adapted for longer spans, particularly with the use of modern materials and techniques. o Advantages: Strong and durable, with a design that efficiently distributes loads. Aesthetically pleasing due to the natural curve. o Disadvantages: Requires strong abutments to resist the horizontal thrust, making construction more complex and costly, especially in areas with poor foundation conditions. o Examples: The Pont du Gard in France, a Roman aqueduct, and the Sydney Harbour Bridge in Australia. Truss Bridges o Structural Form: Truss bridges are composed of a series of connected elements, usually forming triangular units. The truss design distributes the forces throughout the structure, making it highly efficient in carrying loads. o Load Distribution: In a truss bridge, the load is transferred through the truss members, which are typically in tension or compression. The triangular configuration ensures that the structure is stable and distributes the forces evenly. o Materials: Steel is the most common material for truss bridges due to its high tensile strength. In some cases, wood or aluminum may also be used. 8 o Span: Truss bridges can span medium to long distances, typically ranging from 50 to 550 meters. Cantilever truss bridges can cover even longer spans. o Advantages: Efficient use of materials, strong and stable, and can span longer distances without requiring intermediate supports. o Disadvantages: Complex design and construction process, and the extensive use of materials can make it relatively expensive. o Examples: The Forth Bridge in Scotland and the Quebec Bridge in Canada. Suspension Bridges o Structural Form: Suspension bridges feature a deck that is suspended from cables that hang between two tall towers. The main cables are anchored at both ends of the bridge, and vertical suspenders connect these cables to the deck, holding it in place. 9 o Load Distribution: The weight of the bridge deck and any additional load is transferred to the main cables through the vertical suspenders. The main cables then transfer the load to the towers, which bear most of the weight, and to the anchorages, which handle the horizontal forces. o Materials: The primary materials used in suspension bridges are steel for the cables and towers, and concrete or steel for the deck. o Span: Suspension bridges are ideal for long spans, often exceeding 1,000 meters, and can span up to 2,000 meters or more. o Advantages: Capable of spanning very long distances, flexible design allows for movement and adaptation to wind and seismic forces, and aesthetically iconic. o Disadvantages: High cost of construction, significant maintenance requirements, and sensitivity to dynamic loads such as wind and traffic vibrations. o Examples: The Golden Gate Bridge in San Francisco, USA, and the Akashi Kaikyō Bridge in Japan, the world’s longest suspension bridge. Cable-Stayed Bridges o Structural Form: Cable-stayed bridges feature one or more towers (or pylons) from which cables are attached directly to the bridge deck. Unlike suspension bridges, where cables are draped over towers, in cable-stayed bridges, the cables run in straight lines from the tower to the deck. o Load Distribution: The load is transferred directly from the deck to the towers via the cables, which are in tension. The towers, in turn, carry the load to the foundations. This direct load path makes cable-stayed bridges very efficient. o Materials: Steel is commonly used for the cables, while the deck and towers are often constructed from steel or reinforced concrete. o Span: Cable-stayed bridges are suitable for medium to long spans, typically ranging from 200 to 1,000 meters. o Advantages: Efficient use of materials, capable of spanning longer distances without intermediate supports, and often less expensive than suspension bridges for medium spans. They also offer a visually striking design. 10 o Disadvantages: Complex design and construction process, particularly for the cables and towers. They are also more sensitive to dynamic forces than some other types of bridges. o Examples: The Millau Viaduct in France and the Øresund Bridge between Denmark and Sweden. 2. Classification by Material Bridges can also be classified according to the materials used in their construction, which directly impacts their strength, durability, and suitability for different environments: Timber Bridges: One of the earliest materials used in bridge construction, timber is still used today in rural and low-traffic areas. Timber bridges are relatively easy and quick to construct, but they are less durable than those made of modern materials and require more maintenance. Stone Bridges: Used extensively in ancient times, stone bridges, particularly arch bridges, are known for their durability and ability to blend with natural surroundings. While stone is less common today due to the advent of modern materials, many historical stone bridges remain in use, demonstrating their longevity. Steel Bridges: Steel revolutionized bridge construction in the 19th century, allowing for longer spans and more complex designs. Steel’s high strength-to- weight ratio makes it ideal for large-scale bridges, including truss, suspension, and cable-stayed types. Concrete Bridges: Concrete is now one of the most commonly used materials in bridge construction, often reinforced with steel to improve its tensile strength. Pre-stressed and post-tensioned concrete allows for longer spans and more varied design possibilities, making it suitable for modern bridge construction. Composite Bridges: The use of composite materials, which combine two or more materials with different properties, is a growing trend in bridge construction. These materials can be tailored to specific needs, such as reducing weight, increasing durability, or improving resistance to environmental conditions. 3. Classification by Use and Function Bridges can be classified not only by their structural forms but also by their intended usage. The purpose for which a bridge is built significantly influences its design, materials, construction techniques, and maintenance requirements. This classification helps in understanding how different types of traffic and 11 environmental factors impact bridge design and functionality. Classification of bridges based on usage: 1. Highway Bridges a. Purpose and Functionality: o Highway bridges are designed primarily to carry road traffic, including cars, trucks, motorcycles, and sometimes bicycles. These bridges are crucial components of the transportation infrastructure, facilitating the movement of people and goods over obstacles like rivers, valleys, or other roads and railways. o They are built to accommodate various types of vehicular traffic, from light passenger vehicles to heavy commercial trucks. Depending on the location, highway bridges may also include lanes for cyclists and pedestrians. b. Design Considerations: o Load Capacity: Highway bridges must be designed to handle heavy loads, especially when considering the weight of large trucks and the potential for traffic congestion. The design must account for dynamic loads, including the impact of braking, acceleration, and lateral forces from vehicles. o Width and Clearance: The bridge deck must be wide enough to accommodate multiple lanes of traffic, including breakdown lanes and shoulders. Adequate vertical clearance must also be provided for vehicles passing underneath, if applicable. o Safety Features: Highway bridges often include guardrails, barriers, and lighting to ensure the safety of motorists. The design must also consider factors such as skid resistance and drainage to prevent accidents during adverse weather conditions. o Durability: These bridges are exposed to constant vehicular traffic, environmental wear, and sometimes harsh weather conditions. They require robust construction materials like reinforced concrete, steel, and sometimes composite materials to ensure long-term durability. c. Common Types of Highway Bridges: o Beam Bridges: Simple and cost-effective, often used for short spans on highways. o Arch Bridges: Used in scenic or environmentally sensitive areas, providing aesthetic appeal as well as structural strength. o Truss Bridges: Suitable for longer spans, particularly in rural or less densely populated areas. o Suspension and Cable-Stayed Bridges: Used for long spans in major highways, often in urban or densely populated areas. d. Examples: o Golden Gate Bridge (USA): A suspension bridge that is part of U.S. Route 101 and California State Route 1, accommodating heavy highway traffic. o Brooklyn Bridge (USA): A hybrid cable-stayed/suspension bridge that serves both vehicular and pedestrian traffic. 2. Railway Bridges a. Purpose and Functionality: o Railway bridges are specifically designed to carry trains across obstacles. These bridges must accommodate the unique demands of railway traffic, which includes heavy loads and dynamic forces due to the movement of trains. o Railway bridges can carry freight trains, passenger trains, or both. Some railway bridges are also designed to carry high-speed trains, which requires even more precise engineering due to the higher forces involved. b. Design Considerations: o Load Capacity: Railway bridges must support very heavy loads due to the weight of trains, which can exert significant forces on the bridge structure. The design must consider static loads (from stationary trains) and dynamic loads (from moving trains). 12 o Vibration and Deflection: Trains moving at high speeds can cause significant vibrations and deflection in the bridge structure. The design must minimize these effects to ensure the safety and comfort of passengers and the integrity of the cargo. o Track Alignment: Railway bridges must ensure precise track alignment to prevent derailments. This includes considerations for expansion and contraction due to temperature changes, which can affect track geometry. o Durability and Maintenance: Railway bridges are subject to continuous heavy loads and must be constructed from durable materials like steel and reinforced concrete. Regular maintenance is critical to ensure safety and prevent structural failures. c. Common Types of Railway Bridges: o Truss Bridges: Commonly used in railway applications due to their ability to efficiently distribute heavy loads. o Arch Bridges: Often used for railways, especially in hilly or mountainous regions, due to their ability to handle large compressive forces. o Beam Bridges: Used for shorter railway spans, particularly in flat terrains. o Cantilever Bridges: Suitable for long spans where intermediate supports are not feasible, often used in major railway routes. d. Examples: o Forth Bridge (Scotland): A cantilever railway bridge that is a UNESCO World Heritage site, known for its structural efficiency and durability. o Hogwarts Express Bridge (Scotland): A curved arch bridge famously known as the Glenfinnan Viaduct, supporting railway traffic in a scenic location. 3. Pedestrian Bridges a. Purpose and Functionality: o Pedestrian bridges are designed exclusively for foot traffic and, in some cases, cyclists. These bridges are often used to provide safe passage over busy roads, railways, rivers, or other obstacles where it is not practical or safe to cross at grade level. o Pedestrian bridges are also common in parks, recreational areas, and urban environments, where they serve both functional and aesthetic purposes. b. Design Considerations: o Load Capacity: Although pedestrian bridges carry lighter loads than highway or railway bridges, they still need to be designed to support the weight of people, as well as any additional loads from maintenance vehicles or emergency services. o Width and Accessibility: The width of the bridge must accommodate the expected pedestrian traffic, including wheelchairs, strollers, and bicycles. Accessibility features like ramps and elevators may be required to comply with regulations such as the Persons With Disability Act (PWDA). o Safety Features: Pedestrian bridges must include safety features such as handrails, guardrails, and non-slip surfaces. Lighting and surveillance may also be necessary for security, especially in urban areas. o Aesthetics: Pedestrian bridges are often designed with aesthetics in mind, particularly in parks, campuses, and urban settings. The design may include architectural features that blend with or enhance the surrounding environment. c. Common Types of Pedestrian Bridges: o Beam Bridges: Simple, cost-effective solutions for pedestrian crossings, often used in parks and recreational areas. o Arch Bridges: Used for pedestrian crossings where aesthetics and integration with the landscape are important. o Suspension Bridges: Used for longer spans, particularly in scenic areas or over wide obstacles like rivers. 13 o Cable-Stayed Bridges: Sometimes used for pedestrian crossings in urban areas, providing a modern and sleek design. d. Examples: o Millennium Bridge (London, UK): A modern pedestrian suspension bridge spanning the River Thames, known for its iconic design. o The Peace Bridge (Calgary, Canada): A tubular pedestrian bridge that crosses the Bow River, recognized for its striking architectural design. 4. Environmental and Contextual Classification Finally, bridges are classified by the environmental and geographical context in which they are constructed: o Urban Bridges: These are typically designed with both functionality and aesthetics in mind, often becoming landmarks within cities. Urban bridges must navigate space constraints and blend with the existing infrastructure. o Rural Bridges: In contrast, rural bridges are often more utilitarian, designed primarily for functionality with less emphasis on aesthetics. They frequently span natural obstacles like rivers and ravines and must be resilient to local environmental conditions. o Marine and Coastal Bridges: These bridges face unique challenges such as corrosive environments, tides, and waves. Special materials and construction techniques are often required to ensure longevity and safety in these harsh conditions. Bridge and Its Components - A bridge is a complex structure composed of various interconnected components, each playing a critical role in ensuring the stability, functionality, and durability of the entire system. The design and construction of these components are governed by fundamental principles of structural engineering, material science, and environmental considerations, making the study of bridge components a multidisciplinary endeavor. 1. Superstructure Components The superstructure of a bridge refers to all parts of the bridge that are above the supporting piers or abutments. These components are crucial for bearing the loads imposed by traffic and other external forces, and they are designed to efficiently transfer these loads to the substructure. Deck: The deck is the surface of the bridge on which vehicles and pedestrians travel. It is typically made of concrete or steel, though composite materials are becoming increasingly popular. The design of the deck must account for both static and dynamic loads, including the weight of the bridge itself (dead load) and the loads imposed by traffic, wind, and seismic activity (live loads). The deck's design also includes considerations for drainage, expansion joints, and surface treatments to enhance safety and durability. Girders and Beams: Girders and beams are horizontal structural elements that support the deck. Girders are often the primary load-bearing components and are typically made of steel or reinforced concrete. Beams are smaller and are used in conjunction with girders to distribute loads more evenly across the bridge. The design of these elements involves careful analysis of bending moments, shear forces, and deflections to ensure that the bridge can carry the intended loads without excessive deformation or failure. Trusses: In some bridges, especially those spanning longer distances, trusses are used to support the deck. Trusses are frameworks of interconnected triangular units that distribute loads efficiently across the structure. This design allows for greater spans with less material, making truss bridges both strong and economical. The geometric configuration of trusses is critical, as it determines the distribution of forces and the overall stability of the bridge. Cables and Towers (in Cable-Stayed and Suspension Bridges): In cable-stayed and suspension bridges, cables and towers are key components of the superstructure. Cables, typically made of high-strength steel, carry the deck's weight and transfer it to the towers, which then transfer the loads to the foundations. The design and arrangement of these cables are crucial for 14 maintaining the bridge's balance and stability, especially in dynamic conditions such as wind or seismic events. 2. Substructure Components The substructure of a bridge includes all the elements that support the superstructure and transfer its loads to the ground. These components must be designed to withstand not only the loads from the bridge but also environmental factors such as soil conditions, water flow, and seismic activity. Piers: Piers are vertical structures that support the bridge deck and transfer loads from the superstructure to the foundations. They are typically made of reinforced concrete or steel and must be designed to resist bending, shear, and axial forces. The height and spacing of piers are determined by the span length, the type of superstructure, and the environmental conditions at the bridge site. Abutments: Abutments are the end supports of a bridge, which also serve as retaining walls to hold back the earth at the bridge approaches. They transfer loads from the deck to the ground and provide stability to the entire bridge structure. Abutments must be carefully designed to prevent settlement, rotation, and sliding, which could compromise the bridge's integrity. Foundations: Foundations are the lowest part of the bridge substructure, and they transfer loads from the piers and abutments to the ground. Foundations can be shallow (spread footings) or deep (piles or caissons), depending on the soil conditions and the load-bearing requirements. The design of foundations is critical to the overall stability of the bridge, as inadequate foundations can lead to settlement, tilting, or even collapse. 3. Additional Components In addition to the primary structural components, bridges include various other elements that contribute to their functionality, safety, and longevity. Bearings: Bearings are devices placed between the bridge deck and the piers or abutments. They allow for controlled movement of the deck due to thermal expansion, contraction, or seismic activity, while also transferring loads to the substructure. Bearings are typically made of steel or elastomeric materials and are designed to accommodate both vertical and horizontal movements. Expansion Joints: Expansion joints are placed at intervals along the bridge deck to accommodate movement caused by temperature changes, load variations, and other factors. These joints prevent cracking and other damage by allowing the bridge components to expand and contract without imposing additional stresses on the structure. Railings and Parapets: Railings and parapets are safety features designed to prevent vehicles and pedestrians from falling off the bridge. They also contribute to the aesthetic appearance of the bridge. The design of these elements must consider both safety standards and the bridge’s overall aesthetic integration with its surroundings. 4. Environmental and Functional Considerations In addition to structural components, the design of a bridge must take into account environmental and functional factors. These include considerations for water flow in the case of river or coastal bridges, wind forces, seismic activity, and the impact of the bridge on local ecosystems. Modern bridge design often incorporates features such as drainage systems, lighting, and monitoring equipment to enhance the bridge's safety and longevity. Types of Bridges and Their Characteristics - Bridges are vital infrastructure components that facilitate the crossing of physical obstacles such as rivers, valleys, or roads. The design and type of a bridge depend on various factors including geographic conditions, the purpose of the bridge, available materials, and economic constraints. Below is a discussion on the types of bridges and their characteristics, with an emphasis on the use of SI units. 1. Beam Bridges 15 Beam bridges are one of the most basic types of bridges. They consist of a horizontal beam supported at each end by piers or abutments. The weight of the bridge and any load it carries are directly transferred to the piers. Characteristics: o Span: Beam bridges are typically used for short to medium spans, generally up to about 60 meters. o Construction: These bridges are relatively straightforward and economical to construct. o Materials: Common materials include steel, concrete, or wood. o Load Distribution: The load is distributed vertically to the piers, with the maximum bending moment occurring at the center of the beam. o Examples: Simple highway overpasses and pedestrian bridges. 2. Arch Bridges Arch bridges are distinguished by their curved shape. The structure is inherently stable, as the arch transfers the weight of the bridge and its load to the abutments at either end. Characteristics: o Span: Suitable for spans ranging from 30 meters to 240 meters. o Aesthetics: Arch bridges are often selected for their visual appeal. o Strength: The curved design provides high strength, making them ideal for locations with significant loads or challenging terrains. o Materials: Traditionally constructed from stone, but modern versions use steel or reinforced concrete. o Load Distribution: The load is transferred along the curve of the arch to the abutments, effectively handling compressive forces. o Examples: The Pont du Gard in France and the Hell Gate Bridge in New York. 3. Suspension Bridges Suspension bridges have a deck suspended below suspension cables that are anchored at both ends of the bridge. These cables support the majority of the load. Characteristics: o Span: Suspension bridges are ideal for very long spans, sometimes exceeding 2,000 meters, making them suitable for crossing large water bodies. o Flexibility: These bridges are flexible, allowing them to endure strong winds and seismic activity. o Materials: Typically constructed using high-tensile steel. o Load Distribution: The main cables distribute the load to the towers, which then transfer it to the ground through the anchorages. o Examples: The Golden Gate Bridge in San Francisco and the Akashi Kaikyō Bridge in Japan. 4. Cable-Stayed Bridges Cable-stayed bridges feature one or more towers (or pylons) from which cables directly support the bridge deck. Unlike suspension bridges, the cables are attached directly to the towers. Characteristics: o Span: Ideal for medium to long spans, generally between 150 meters and 850 meters. o Aesthetics: The fan-like or harp-like cable arrangement provides an elegant and modern appearance. o Construction: These bridges are generally easier and less expensive to construct than suspension bridges for similar spans. o Materials: Typically made from steel or concrete. o Load Distribution: The load is directly transferred from the cables to the towers, which then distribute it to the foundations. o Examples: The Millau Viaduct in France and the Øresund Bridge between Denmark and Sweden. 16 5. Truss Bridges Truss bridges are characterized by a framework of interconnected elements that form triangular units. This design is highly efficient in distributing loads. Characteristics: o Span: Truss bridges can span distances from 15 meters to 450 meters. o Load Distribution: The triangular units distribute the load evenly, making the bridge capable of supporting substantial loads. o Materials: Often constructed from steel, which provides high tensile strength. o Construction: Although complex to design and build, truss bridges offer significant strength and efficiency. o Examples: The Quebec Bridge in Canada and the Forth Bridge in Scotland. 6. Cantilever Bridges Cantilever bridges are constructed using structures that project horizontally into space, supported only on one end. These bridges typically consist of two cantilever arms extending from opposite sides and meeting in the middle. Characteristics: o Span: Suitable for spans between 45 meters and 550 meters. o Load Distribution: The cantilever arms distribute the load back to the piers, which must be firmly anchored. o Materials: Typically constructed using steel or prestressed concrete. o Flexibility: Cantilever bridges are flexible in design and construction, especially in locations where it is difficult to build support piers. o Examples: The Quebec Bridge in Canada and the Forth Bridge in Scotland. Selection of Bridge Type - The selection of a bridge type is a critical decision in the field of civil engineering and infrastructure development. This decision has far-reaching implications on the bridge's structural integrity, cost-effectiveness, aesthetic value, and environmental impact. The process of selecting the most appropriate bridge type for a particular site involves a careful analysis of various factors, including the physical and environmental conditions of the site, the intended function of the bridge, material availability, economic constraints, and long-term maintenance considerations. 1. Site Conditions The physical and environmental conditions of the site are often the most significant determinants in the selection of a bridge type. Key considerations include the following: Topography: The topography of the site, including the presence of valleys, rivers, and other obstacles, directly influences the choice of bridge. For example, a suspension bridge may be suitable for spanning wide bodies of water, while an arch bridge might be preferred for crossing deep valleys. Geotechnical Conditions: The type of soil and rock at the site affects the foundation design, which in turn influences the choice of bridge. For instance, a site with poor soil conditions may require deep foundations, making some types of bridges, such as cantilever or suspension bridges, more viable than others. Hydrological Factors: For bridges over rivers or flood-prone areas, considerations such as water flow, tidal movements, and potential for scouring are crucial. These factors can dictate the need for specific types of foundations and piers, which might favor certain bridge types like beam or arch bridges. Environmental Impact: Environmental regulations and the potential impact on the surrounding ecosystem can influence the bridge selection process. Minimizing the ecological footprint may lead to the choice of a bridge type that requires fewer piers in the water or that can be constructed with minimal disruption to the environment, such as cable-stayed bridges. 2. Functionality and Load Requirements The intended use of the bridge plays a pivotal role in its selection. The bridge must be designed to accommodate the expected loads and traffic patterns, which can vary significantly based on its function. 17 Traffic Type and Volume: Bridges designed for vehicular traffic, railways, pedestrians, or a combination of these will have different requirements. For example, a heavy rail bridge might necessitate a truss design due to its high load-bearing capacity, whereas a pedestrian bridge could be a simple beam or arch bridge. Load Capacity: The type of load the bridge is expected to carry, including live loads (vehicles, trains, pedestrians) and dead loads (the weight of the bridge structure itself), must be considered. High load requirements may necessitate the use of stronger materials or more complex bridge designs, such as suspension or truss bridges. Span Length: The distance the bridge needs to span without intermediate supports is a critical factor. Long spans generally require more complex designs such as suspension or cable-stayed bridges, while shorter spans can be effectively served by simpler beam or arch bridges. 3. Material Availability and Selection The choice of materials for constructing a bridge is another critical factor that influences the selection of the bridge type. The availability, cost, and properties of materials dictate the feasibility and sustainability of different bridge designs. Material Strength and Durability: Materials must be selected based on their ability to withstand the stresses and environmental conditions the bridge will encounter. For example, steel is often chosen for its high tensile strength, making it ideal for suspension and truss bridges, while concrete, with its compressive strength, is well-suited for arch and beam bridges. Material Availability: Local availability of materials can significantly influence the cost and feasibility of construction. For instance, if high-quality steel is readily available, a steel truss or suspension bridge might be the most practical choice. Environmental Considerations: The environmental impact of material extraction, transportation, and construction also plays a role in material selection. Sustainable materials or those that reduce the carbon footprint may be prioritized, influencing the bridge type. 4. Economic Constraints The budget available for the bridge project is a critical factor in determining the bridge type. The costs associated with construction, maintenance, and long-term durability must be balanced against the available funding. Initial Construction Costs: Different bridge types vary significantly in their construction costs. Beam bridges, for example, are typically less expensive to build than suspension or cable-stayed bridges, which require more complex designs and construction techniques. Maintenance and Life-Cycle Costs: Beyond initial construction, the long-term maintenance and operational costs must be considered. Some bridge types, such as concrete beam bridges, might have lower maintenance costs, while others, like suspension bridges, may require regular inspections and maintenance to ensure their cables and other components remain in good condition. Cost-Benefit Analysis: A thorough cost-benefit analysis that includes both initial and life-cycle costs is essential to determining the most economically viable bridge type for a given project. 5. Aesthetic and Cultural Considerations Bridges are often iconic structures that contribute to the visual and cultural landscape of an area. As such, aesthetic and cultural factors can play a significant role in bridge selection. Aesthetic Appeal: The visual impact of a bridge can be a decisive factor, especially in urban or scenic environments. Cable-stayed and arch bridges are often favored for their aesthetic qualities, while beam bridges might be chosen for their unobtrusiveness. 18 Cultural Significance: In some cases, the bridge may need to reflect cultural or historical values. For instance, in a historic district, an arch bridge made from stone might be selected to complement the surrounding architecture. 6. Regulatory and Safety Requirements Compliance with local, national, and international regulations, as well as safety standards, is non-negotiable in bridge design and selection. Building Codes and Standards: The bridge must meet all relevant engineering standards and codes, which dictate specific design requirements for safety, durability, and resilience. Seismic and Wind Considerations: In areas prone to earthquakes or strong winds, certain bridge types may be more appropriate. For example, suspension bridges are designed to flex and move with wind forces, while certain types of truss or arch bridges may be more resilient against seismic activity. Redundancy and Resilience: The design must incorporate safety features that ensure the bridge can withstand unexpected loads or environmental events without catastrophic failure. This might involve selecting a bridge type known for its resilience or incorporating additional safety measures into the design. Essential Design Data and Their Acquisition - In the field of civil engineering and structural design, the acquisition of essential design data is a foundational step that underpins the successful execution of any project. - This data informs every aspect of the design process, from conceptualization to detailed engineering, and ultimately to construction and operation. - The accuracy, relevance, and comprehensiveness of the design data directly influence the safety, functionality, and longevity of the structure. - This discussion explores the various types of essential design data, the methods of acquiring this data, and the importance of integrating these elements into the design process. 1. Types of Essential Design Data The essential design data required for any engineering project can be categorized into several key areas: Geotechnical Data Geotechnical data pertains to the physical properties of the soil and rock at the construction site. This data is crucial for designing foundations, assessing site stability, and predicting potential challenges related to ground conditions. o Soil Composition: Information about the type, density, moisture content, and stratification of soil layers. o Bearing Capacity: The ability of the soil to support the load of the structure without excessive settlement. o Shear Strength: The soil's resistance to shear stress, which affects slope stability and foundation design. o Groundwater Level: The position of the water table and the potential for groundwater fluctuations, which can impact foundation design and construction methods. Topographical Data Topographical data involves the mapping of the physical features of the land, including elevations, contours, and natural and man-made features. Accurate topographical data is essential for site planning, determining cut and fill requirements, and ensuring proper drainage. o Elevation and Contour Maps: Detailed maps showing the height of the land and its slope. o Surface Features: Identification of natural features (e.g., rivers, trees) and man-made features (e.g., roads, buildings) that may influence design decisions. o Existing Infrastructure: Information about existing utilities, transportation networks, and other infrastructure that may interact with the new structure. Environmental Data Environmental data includes information about the climate, weather patterns, and ecological conditions at the site. This data is critical for 19 designing structures that are resilient to environmental forces and for ensuring compliance with environmental regulations. o Climate Data: Historical data on temperature, humidity, precipitation, and wind patterns, which influence material selection and structural design. o Seismic Data: Information about the seismic activity in the region, which is essential for designing earthquake-resistant structures. o Hydrological Data: Data on rainfall, river flow rates, and flood levels to assess flood risks and design appropriate drainage and flood protection systems. Material Properties Data Understanding the properties of the materials to be used in construction is fundamental to ensuring that the structure will perform as intended under various loads and environmental conditions. o Strength and Durability: Data on the compressive, tensile, and shear strength of materials like concrete, steel, and wood. o Thermal Properties: Information on how materials expand, contract, or degrade under temperature variations. o Corrosion and Weathering: Data on how materials respond to exposure to the elements, such as moisture, salt, and pollutants. Load Data Load data encompasses the forces and stresses that the structure must be designed to withstand. This includes both static and dynamic loads. o Dead Loads: The weight of the structure itself, including all permanent fixtures and finishes. o Live Loads: The variable forces from occupants, vehicles, and environmental conditions such as rain, wind, and earthquakes. o Impact and Dynamic Loads: Forces that occur suddenly, such as those from vehicles or seismic activity, which can significantly affect the structural integrity. Regulatory and Code Requirements Compliance with local, national, and international building codes and regulations is mandatory. These codes specify minimum standards for safety, durability, and performance. o Structural Codes: Regulations that dictate the minimum design standards for structural integrity, fire resistance, and energy efficiency. o Zoning Laws: Local regulations that govern land use, building heights, and setbacks from property lines. o Environmental Regulations: Laws that require environmental impact assessments and adherence to sustainability practices. 2. Methods of Acquiring Essential Design Data - The acquisition of essential design data involves a combination of fieldwork, laboratory testing, historical research, and the use of advanced technologies. - The methods used to gather this data must be precise and appropriate for the specific needs of the project. Site Surveys Site surveys are conducted to gather topographical and geotechnical data directly from the project location. These surveys are typically the first step in the design process. Topographic Surveys: Using instruments such as total stations, GPS, and drones to create detailed maps of the site. Geotechnical Investigations: Involving methods such as borehole drilling, soil sampling, and in-situ testing to determine soil properties and groundwater conditions. Laboratory Testing Laboratory testing is used to analyze samples of soil, rock, water, and construction materials. This data helps in determining the suitability and strength of materials under controlled conditions. Soil Testing: Laboratory tests such as grain size analysis, Atterberg limits, and compaction tests to understand soil behavior. Material Testing: Tests on concrete, steel, and other construction materials to assess their mechanical properties, such as compressive strength, elasticity, and corrosion resistance. 20 Environmental Monitoring Environmental monitoring involves the collection of data related to weather, climate, and ecological conditions. This data is often gathered over time to capture seasonal variations and long-term trends. Weather Stations: On-site weather stations to collect real-time data on temperature, humidity, wind speed, and rainfall. Hydrological Monitoring: Installing sensors and gauges to monitor water levels, flow rates, and water quality in nearby rivers or lakes. Historical Data Analysis Analyzing historical data is essential for understanding past events that could influence the design, such as previous floods, earthquakes, or significant weather patterns. Seismic Records: Studying past earthquake records to estimate the seismic risk at the site. Flood History: Reviewing historical flood data to determine the highest water levels and the frequency of flood events. Remote Sensing and GIS Remote sensing and Geographic Information Systems (GIS) are powerful tools for acquiring and analyzing spatial data over large areas. Satellite Imagery: Using satellite images to monitor changes in land use, vegetation cover, and surface conditions over time. GIS Mapping: Creating layered maps that integrate various data sources, such as topography, geology, and infrastructure, to visualize and analyze spatial relationships. Regulatory and Code Research Researching applicable building codes, zoning laws, and environmental regulations is essential to ensure that the design complies with all legal requirements. Code Reviews: Systematic review of relevant codes and standards to identify specific design criteria. Consultations with Authorities: Engaging with local authorities to clarify requirements and obtain necessary permits and approvals. 3. Importance of Integrating Essential Design Data into the Design Process - The integration of essential design data into the design process is critical to the success of any engineering project. - The following points highlight the importance of this integration: Ensuring Structural Safety Accurate and comprehensive design data is essential to ensure that the structure can withstand all anticipated loads and environmental conditions. Geotechnical data, for instance, is crucial for designing foundations that prevent excessive settlement or failure. Optimizing Design Efficiency By having detailed knowledge of the site conditions, materials, and environmental factors, engineers can optimize the design for efficiency. This may involve selecting the most suitable materials, minimizing waste, and reducing costs while maintaining safety and performance. Mitigating Risks Early identification of potential risks, such as soil instability or flood hazards, allows for the incorporation of appropriate mitigation measures into the design. This proactive approach can prevent costly design revisions and construction delays. Compliance with Regulations Ensuring that the design meets all regulatory requirements is vital to obtaining approvals and avoiding legal challenges. Accurate data helps in demonstrating compliance with building codes, zoning laws, and environmental regulations. Sustainability Considerations Integrating environmental data into the design process enables the development of sustainable structures that minimize environmental impact and reduce energy consumption. This is increasingly important in the context of climate change and environmental stewardship. Facilitating Stakeholder Communication Clear and precise design data is essential for effective communication among project stakeholders, including engineers, architects, contractors, and regulators. This data provides a common 21 basis for decision-making and ensures that all parties are aligned with the project’s goals. Fundamentals of Bridge Design - Bridge design is a multidisciplinary field that combines engineering principles, material science, and architecture to create structures that safely and efficiently span physical obstacles such as rivers, valleys, roads, and railways. - The process of bridge design is complex and requires careful consideration of various factors, including load-bearing capacity, environmental impact, aesthetics, and durability. 1. Understanding the Purpose and Requirements Bridge design is a multidisciplinary field that requires a comprehensive understanding of engineering principles, material science, environmental factors, and societal needs. At its core, the design of a bridge involves balancing multiple requirements and constraints to ensure that the structure serves its intended purpose safely, efficiently, and economically. Below is a detailed exploration of the fundamental concepts related to the purpose and requirements in bridge design. a. Purpose of Bridge Design The primary purpose of bridge design is to create a structure that provides safe passage over obstacles such as rivers, valleys, roads, or other transportation routes. The key elements of purpose-driven bridge design include: i. Transportation Functionality o Bridges serve as connectors in transportation networks, facilitating the movement of people, goods, and vehicles. The type and volume of traffic the bridge will accommodate are central to its design. Common considerations include: Vehicle Type: Bridges may be designed for vehicles (highways), trains (railroads), pedestrians, or cyclists. This significantly influences the design loads and geometric configurations. Traffic Volume: Traffic analysis is critical to determine the expected load on the bridge during its design life. Design codes often prescribe load combinations, including pedestrian, vehicular, and environmental loads. Bridge Span and Clearance: The distance between the abutments (the ends of the bridge) and the vertical clearance beneath the bridge will depend on the geographical and infrastructural characteristics of the site. ii. Site-Specific Functionality o The geographical features and environmental conditions of the bridge location profoundly affect the design. Key site-specific factors include: Topography: The elevation differences and surface features such as rivers, ravines, or roadways dictate the required span length and the foundation depth. Environmental Conditions: The design must consider potential exposure to elements like wind, water, snow, and seismic activity, which can impose dynamic and extreme loads on the structure. Navigation Requirements: For bridges spanning waterways, the height and width of the bridge should allow for ship and boat traffic. This influences the clearance dimensions for the design. iii. Aesthetic Considerations o Aesthetics can be an important purpose in bridge design, particularly for structures in urban or high-profile locations. The appearance of a bridge often reflects the local culture, history, and environment. While aesthetics must be balanced with functional and structural requirements, some bridges are designed as architectural landmarks. 22 iv. Economic and Social Impact o The design must consider the economic feasibility of construction, maintenance, and repair. A well-designed bridge should minimize long- term costs and provide maximum benefit to the community it serves. Additionally, the bridge may serve broader economic functions, such as connecting business hubs or facilitating trade. b. Requirements of Bridge Design Understanding the requirements in bridge design is crucial to ensure the structure is safe, durable, and meets the needs of the users and the environment. These requirements are governed by engineering standards, codes, and best practices, which cover various aspects of structural performance. i. Structural Integrity and Safety o The safety of a bridge is paramount. The design must ensure that the bridge can support the maximum expected loads without collapsing or experiencing excessive deformation. Key considerations for structural integrity include: Load-Bearing Capacity: The bridge must be designed to handle both dead loads (the weight of the structure itself) and live loads (vehicles, pedestrians, trains) along with environmental loads (wind, earthquakes, snow). Redundancy and Ductility: To ensure resilience, especially in critical infrastructure, redundancy is designed into the system so that the failure of one component does not lead to a catastrophic collapse. Ductile materials allow for deformation under stress, providing warning before failure. Stability: The bridge must be designed to resist overturning, sliding, or excessive deflection under various load combinations. This requires a comprehensive analysis of lateral forces, including seismic loads, wind forces, and impact from vehicles. ii. Compliance with Design Codes and Standards o Various international, national, and local building codes and standards govern bridge design. These codes ensure the bridge's safety, reliability, and serviceability. Some important codes and guidelines include: AASHTO LRFD (Load and Resistance Factor Design): In the U.S., the American Association of State Highway and Transportation Officials (AASHTO) sets standards for highway bridge design using LRFD methodologies. Eurocodes: European bridge designs are governed by Eurocodes, which provide comprehensive guidelines for loads, materials, and safety factors. Seismic Codes: In seismic regions, specialized codes such as the AASHTO Guide Specifications for Seismic Bridge Design ensure the bridge can withstand earthquakes without catastrophic failure. iii. Serviceability and Durability o While strength and safety are essential, serviceability addresses the bridge’s performance under normal conditions. The design must ensure: Deflection Control: The bridge should not deflect excessively under load, as this can cause discomfort for users and potential damage to structural components. Fatigue Resistance: Bridges experience repetitive loading from vehicles, wind, and temperature fluctuations. The materials and joints must be designed to resist fatigue over the bridge’s service life. Durability Against Environmental Degradation: The design must consider the bridge’s exposure to corrosive environments (e.g., near saltwater bodies), extreme temperatures, and weathering. Material selection and protective coatings are critical to preventing long-term damage. 23 iv. Economic Feasibility o Cost considerations include initial construction costs, as well as long- term maintenance and operation expenses. Bridge design should minimize both by: Material Efficiency: The selection of materials (e.g., steel, concrete, composites) is optimized to balance cost, strength, and durability. Modern bridge design often incorporates high-performance materials that provide longer service life with reduced maintenance. Construction Methods: Design decisions must align with construction techniques that reduce time and cost. For example, prefabricated elements can speed up construction and reduce site-related expenses. Maintenance and Inspection: The design must facilitate regular inspection and maintenance. This includes ensuring access for workers and incorporating features that allow for easier identification of potential issues, such as corrosion or fatigue cracking. v. Environmental and Sustainability Considerations o Modern bridge design increasingly emphasizes sustainability, minimizing the environmental impact during construction and throughout the bridge’s life cycle. Key sustainability goals include: Minimal Environmental Disruption: The design should minimize harm to the natural environment, particularly in ecologically sensitive areas such as wetlands, rivers, and urban green spaces. Use of Sustainable Materials: The incorporation of recycled materials or low-carbon construction methods can significantly reduce the environmental footprint. Long-Term Environmental Impact: The design should aim to reduce the need for repairs and replacements, thereby minimizing the depletion of natural resources and energy consumption. vi. Special Requirements for Complex Bridge Types o Certain bridges, such as long-span, cable-stayed, or suspension bridges, have unique design requirements due to the complexities of their structural systems. These designs involve advanced analysis techniques like finite element modeling to ensure the stability and performance of the bridge. For instance: Aerodynamic Stability: Long-span bridges are vulnerable to wind- induced oscillations. Detailed aerodynamic studies are required to mitigate phenomena like vortex shedding or flutter. Seismic Isolation: In seismic regions, special devices (e.g., base isolators, dampers) are incorporated to absorb earthquake energy and prevent damage to the bridge structure. 2. Structural Analysis and Design Principles - Bridge design is a multifaceted process that requires the integration of structural analysis and design principles to ensure safety, functionality, durability, and cost- effectiveness. - Structural analysis allows engineers to understand how forces interact with the bridge and affect its components, while design principles guide the process of selecting the most efficient and safe structural systems and materials. a. Overview of Structural Analysis in Bridge Design Structural analysis involves calculating the effects of loads and forces acting on a bridge, including how these forces are distributed throughout the structure. It provides insights into the bridge’s behavior under normal and extreme conditions. The goal of structural analysis is to ensure that the bridge can support the required loads safely and efficiently over its design life. i. Types of Loads on Bridges o Understanding the different types of loads acting on a bridge is a critical component of structural analysis. These loads can be broadly 24 categorized into dead loads, live loads, environmental loads, and dynamic loads. Dead Loads: These are the permanent forces due to the weight of the bridge itself, including the deck, girders, cables, pylons, and any other structural components. The magnitude of dead loads depends on the materials used in the bridge, and they must be calculated accurately to avoid overloading the structure. Live Loads: These are transient loads, such as vehicles, trains, pedestrians, and cyclists. Live loads vary in magnitude and distribution over time. Bridge design codes provide guidelines for calculating live loads, including dynamic amplification factors to account for vehicle movement and vibrations. Environmental Loads: Environmental forces include wind, water (river currents, tidal forces), snow, and temperature effects (expansion/contraction). Wind loads are critical for long-span bridges like suspension and cable-stayed bridges. In areas prone to floods, hydrodynamic forces from flowing water can significantly affect bridge piers and abutments. Seismic Loads: In earthquake-prone areas, seismic analysis is required to assess the bridge’s response to ground motion. Seismic loads are dynamic and often induce significant stresses and strains in the bridge, requiring special considerations in design. ii. Methods of Structural Analysis o There are various methods for analyzing how these loads interact with the structure of the bridge. The choice of method depends on the complexity of the bridge design, the load types, and the accuracy required. Linear Static Analysis: This is the simplest form of analysis and assumes that the relationship between applied forces and displacements is linear. It is useful for small and simple bridge designs with well-defined load paths. Non-Linear Analysis: Non-linear analysis accounts for the non-linear behavior of materials (e.g., yielding of steel) and geometric non- linearity (e.g., large deflections). This is essential for large-span or complex bridges, where deformations can lead to significant changes in the load paths. Dynamic Analysis: Dynamic analysis is required for bridges subjected to time-dependent loads such as earthquakes, wind, or moving vehicles. Methods like modal analysis and time-history analysis help engineers understand how a bridge will respond to these dynamic loads, including resonance and vibration effects. Finite Element Method (FEM): FEM is a computational technique widely used in bridge analysis. The bridge is broken down into a mesh of small elements, and the forces and displacements within each element are calculated. FEM allows for highly accurate modeling of complex geometries and material behaviors, making it indispensable in modern bridge engineering. b. Fundamental Design Principles in Bridge Design Once the structural analysis is complete, design principles are applied to develop a bridge that can withstand the calculated forces and meet all relevant criteria for safety, serviceability, and durability. The design process is governed by principles that ensure the bridge performs its intended function efficiently over its lifespan. i. Design for Strength and Stability o The most fundamental design principle is to ensure that the bridge is strong enough to support all the anticipated loads and remain stable under various conditions. 25 Material Selection: The choice of materials (e.g., steel, reinforced concrete, prestressed concrete) is based on their mechanical properties such as strength, ductility, and durability. The material should be able to carry the maximum load without failure while providing enough flexibility to absorb energy (ductility) under extreme conditions like earthquakes. Load Path and Force Distribution: A well-designed bridge efficiently transfers forces from the deck (where loads are applied) to the supports (piers and abutments). The load path should minimize the stress concentrations at any single point and ensure even force distribution. Bridge types like trusses, arches, suspension, and cable- stayed bridges each distribute loads differently, and the choice of structure is driven by the specific site and load conditions. Factor of Safety: Engineers incorporate safety factors to account for uncertainties in material properties, load predictions, and future conditions. This ensures that the bridge can handle unexpected overloads or material degradation over time. ii. Design for Serviceability o Serviceability refers to the bridge's performance under normal operating conditions without excessive deformation, vibration, or discomfort to users. Deflection Limits: Bridges are designed to limit deflection under live loads to avoid discomfort for users and prevent damage to structural and non-structural components. Excessive deflection can cause cracking in the deck and lead to long-term serviceability issues. Vibration Control: Bridges must also be designed to avoid excessive vibrations, particularly those caused by moving vehicles or pedestrians. Long-span bridges like cable-stayed and suspension bridges are especially sensitive to vibrations and require special design considerations such as tuned mass dampers or aerodynamic shaping to minimize oscillations. iii. Design for Durability and Maintenance o Durability is crucial to ensure that the bridge remains safe and functional throughout its design life with minimal maintenance. Corrosion Protection: Bridges exposed to harsh environments (e.g., coastal areas) must incorporate corrosion-resistant materials or coatings to protect against the damaging effects of moisture and salt. Steel components are often galvanized or painted with special coatings, while concrete can be mixed with additives to improve its resistance to water infiltration. Fatigue Resistance: Repetitive loading from vehicles, wind, and temperature changes can cause fatigue in materials over time, leading to cracks and eventual failure. Bridges are designed to ensure that critical components have enough fatigue resistance to withstand the expected number of load cycles over their lifespan. Access for Inspection and Maintenance: The design must facilitate regular inspection and maintenance to detect early signs of distress or deterioration. This includes providing access points for inspectors, such as catwalks, ladders, or maintenance platforms. iv. Redundancy and Robustness o A robust bridge design includes redundancy, meaning that the failure of one element does not lead to the collapse of the entire structure. This is especially important in bridges classified as critical infrastructure, where failure would have catastrophic consequences. Redundant Load Paths: For example, in truss bridges, the loads are distributed among multiple members. If one member fails, the remaining members can carry the load, preventing a complete collapse. 26 Seismic Detailing: In earthquake-prone areas, seismic detailing (e.g., reinforcing concrete members, using flexible joints) ensures that the bridge can deform during an earthquake without suffering a brittle failure. v. Cost-Effectiveness and Constructability o Designing a bridge is not just about strength and durability; it also involves making decisions that optimize costs without compromising safety or functionality. Material Efficiency: Engineers seek to minimize the amount of material used without sacrificing performance. This includes selecting efficient cross-sections for girders, optimizing the spacing of piers, and using high-performance materials that offer better strength-to- weight ratios. Ease of Construction: The bridge design must consider the practical aspects of construction, such as the availability of materials, local construction methods, and the time required to complete the structure. Prefabrication, modular construction, and accelerated bridge construction (ABC) techniques are increasingly used to speed up the process and reduce labor costs. c. Integration of Structural Analysis and Design The integration of structural analysis and design is a continuous and iterative process. Engineers begin by performing preliminary analyses based on assumed loads and structural systems. These results are used to make initial design decisions, which are then refined through further analysis. This cycle continues until a satisfactory design is achieved. o Iterative Refinement: If an initial analysis shows that a certain member or system is over-stressed, the design is modified—either by increasing the size of the member, changing the material, or altering the load paths. This process continues iteratively until an optimal balance between strength, serviceability, and cost is reached. o Use of Design Codes: Design codes such as the AASHTO LRFD (Load and Resistance Factor Design) Bridge Design Specifications or Eurocodes provide guidelines for the analysis and design process. These codes specify the minimum requirements for load combinations, material properties, and safety factors, ensuring consistency and reliability in bridge design across different projects. 3. Bridge Types and Structural Systems - Bridge design involves selecting the most suitable bridge type and structural system to meet the project’s specific requirements, such as spanning obstacles, supporting loads, and withstanding environmental forces. - The choice of bridge type is guided by factors like span length, site conditions, material availability, and load requirements. - Different structural systems are used to distribute and transfer loads efficiently, while ensuring safety, durability, and constructability. Here, we’ll delve deeply into the types of bridges and their structural systems, which form the backbone of bridge design. a. Categories of Bridges by Structural Action Bridges are typically classified based on how they support loads and how those loads are transferred to the foundations. This classification determines the behavior of the structure under various loads, guiding decisions on materials, construction methods, and structural detailing. i. Beam Bridges (Girder Bridges) Beam bridges are the simplest type of bridge, consisting of horizontal beams supported by piers or abutments at each end. The load is carried by the beam, which transfers it to the supports through vertical reactions. Key Features: 27 Load Distribution: The loads (dead, live, and environmental) on the bridge are directly transferred to the supports at the ends of the beam. Material Use: Steel, reinforced concrete, or prestressed concrete are commonly used for the girders. Span Length: Beam bridges are most effective for short-to-medium spans, typically up to 100 meters. Structural Systems: Simple Beam Bridge: A single-span beam bridge where the beam rests on supports at either end. The span length is typically short, as bending moments and deflections increase with longer spans. Continuous Beam Bridge: Consists of multiple spans connected continuously over intermediate supports. This type provides better load distribution and reduces bending moments, making it more economical for medium spans. Advantages: Simplicity in design and construction. Easily adaptable for short and medium spans. Cost-effective for straightforward applications. Disadvantages: Limited span length. Larger deflections and bending moments compared to more advanced bridge types. ii. Arch Bridges An arch bridge uses a curved structure to transfer loads primarily through compression. The arch distributes loads horizontally toward the supports (abutments), reducing the need for large bending moments in the bridge’s superstructure. Key Features: Load Distribution: Loads are transferred in a radial direction along the arch to the abutments. This allows for efficient load transfer, minimizing bending moments in the structure. Material Use: Typically constructed using materials strong in compression, such as stone, reinforced concrete, or steel. Span Length: Arch bridges are effective for medium-to-long spans, ranging from 30 meters to several hundred meters. Structural Systems: Fixed Arch: A traditional arch bridge with fixed supports, where the shape of the arch itself resists external forces. Fixed arches are rigid and are designed to carry static loads efficiently. Hinged Arch: These arches have hinges at the crown and abutments, which allow slight rotations to relieve stress due to temperature changes or settlement. This provides more flexibility under dynamic loads. Advantages: Efficient use of materials due to compression-dominated load paths. Aesthetic appeal, often seen as a landmark structure. Ability to span long distances without piers in the water, making it suitable for areas where mid-span supports are not feasible. Disadvantages: Complex design and construction processes, especially for longer