Computed Tomography PDF
Document Details
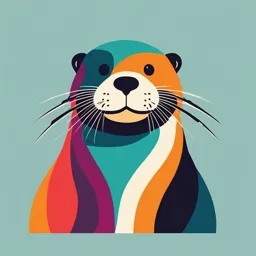
Uploaded by HilariousSchorl
Elias Estephan, Ph.D.
Tags
Summary
This document provides an overview of computed tomography (CT), covering its principles, geometry, historical development, detectors, acquisition, reconstruction methods, radiation dose considerations and image quality. It's a detailed guide to the technical aspects of this medical imaging modality.
Full Transcript
Computed Tomography 1. Basic principles Tomographic acquisition Tomographic reconstruction 2. Geometry and historical development First generation : rotate/ translate, pencil beam Second generation : rotate/translate , narrow beam Third generation : rotate /rotate , wide fan...
Computed Tomography 1. Basic principles Tomographic acquisition Tomographic reconstruction 2. Geometry and historical development First generation : rotate/ translate, pencil beam Second generation : rotate/translate , narrow beam Third generation : rotate /rotate , wide fan beam Fourth generation : rotate/stationary Fifth generation : stationary /stationary Sixth generation : helical Seventh generation : multiple detector array 3. Detectors and detector arrays Xenon detectors Solid-state detectors Multiple detector arrays 1 Computed Tomography Elias Estephan, Ph.D. Computed Tomography 7. Radiation dose 4. Details of acquisition Dose measurement Slice thichness : single detector array scanners Dose considerations in Slice thickness : multiple detector array scanners helical scanning Detector Pitch and collimator Pitch 8.Image quality 5. Tomographic reconstruction Factors affecting Rays and Views: The Sinogram spatial resolution Processing of the data Factors affecting Interpolation (Helical) contrast resolution Simple and filtered backprojrction reconstruction CT numbers or Hounsfield Units 9. Artifacts Beam hardening 6. Digital image display Motion artifacts Partial volume Averaging 2 Computed Tomography Elias Estephan, Ph.D. Computed Tomography CT was the first imaging modality that made it possible to probe the inner depths of the body, slice by slice 1. Basic principles 3D anatomy of the patient is reduced to a 2D projection image. The density at a given point x-ray attenuation properties along a line between the x-ray focal spot and the point on the detector 3 Computed Tomography Elias Estephan, Ph.D. conventional radiograph of the patient's anatomy, information with respect to the dimension parallel to the x-ray beam is lost. This limitation can be overcome 1. PA chest image height and width 2. lateral projection provides information about the height and depth 4 Computed Tomography Elias Estephan, Ph.D. Imagine that instead of just two projections, a series of 360 radiographs were acquired at 1 degree angular intervals around the patient's However, the 360 radiographic images display the anatomic information in a way that would be impossible for a human to visualize: cross-sectional images. If these 360 images were stored into a computer, the computer could in principle reformat the data and generate a complete thoracic CT examination. 5 Computed Tomography Elias Estephan, Ph.D. The tomographic image is a picture of a slab of the patient's anatomy. The 2D CT image corresponds to a 3D section of the patient CT slice-thickness is very thin (1 to 10 mm) and is approximately uniform. The 2D array of pixels in the CT image corresponds to an equal number of 3D voxels (volume elements) in the patient. Voxels have the same in-plane dimensions as pixels, but they also include the slice thickness dimension. Each pixel on the CT image displays the average x-ray attenuation properties of the tissue in the corresponding voxel 6 Computed Tomography Elias Estephan, Ph.D. Tomographic acquisition A single transmission measurement through the patient made by a single detector at a given moment in time is called a ray. A series of rays that pass through the patient at the same orientation is called a projection or view. There are two projection geometries that have been used in CT imaging parallel beam geometry rays in a projection are parallel fan beam geometry angle diverge and have the appearance of a fan. modern CT scanners use fan beam geometry The acquisition of a single axial CT image may involve approximately 800 rays taken at 1,000 different projection angles, 800,000 transmission measurements. for the next slice, the table (patient) is moved slightly in the "z-axis" of the scanner different slice of tissue in the path of the x-ray beam for the acquisition of the next image. 7 Computed Tomography Elias Estephan, Ph.D. Tomographic reconstruction Each ray transmission measurement through the patient along a line the detector measures an x-ray intensity, It. The unattenuated intensity of the x-ray beam is also measured during the scan by a reference detector, and this detects an x-ray intensity I0, thickness of the patient along the ray µ is the average linear attenuation along the ray Notice that It and I0 are machine-dependent values µt is an important parameter relating to the anatomy of the patient the measured values It and I0 can be used to calculate the parameter of interest: 8 Computed Tomography Elias Estephan, Ph.D. This computation, reduces the dependency of the CT image on the machine-dependent parameters, resulting in an image that depends primarily on the patient's anatomic characteristics. After preprocessing of the raw data, a CT reconstruction algorithm is used to produce the CT images. There are numerous reconstruction strategies: filtered backprojection The backprojection (computer) reversing the acquisition steps During backprojection reconstruction, the µ value for each ray is smeared along this same path in the image of the patient. areas of high attenuation tend to reinforce each other and areas of low attenuation also reinforce image in the computer. 9 Computed Tomography Elias Estephan, Ph.D. 2. Geometry and historical development First generation : rotate / translate, pencil beam Only two x-ray detectors were used, and they measured the transmission of x-rays through the patient for two different slices. This system used parallel ray geometry. Starting at a particular angle, the x-ray tube and detector system translated linearly across the field of view (FOV), acquiring 160 parallel rays across a 24- cm FOV. system was rotated slightly, another translation This procedure was repeated until 180 projections were acquired at 1-degree intervals. A total of 180 x 160 = 28,800 rays were measured. 10 Computed Tomography Elias Estephan, Ph.D. One advantage of the first-generation CT scanner was that it employed pencil beam geometry-only two detectors measured the transmission of x-rays through the patient. The pencil beam allowed very efficient scatter reduction, because scatter that was deflected away from the pencil ray was not measured by a detector. With regard to scatter rejection, the pencil beam geometry used in first-generation CT scanners was the best 11 Computed Tomography Elias Estephan, Ph.D. Second generation : rotate/translate , narrow beam The next incremental improvement to the CT scanner was the incorporation of a linear array of 30 detectors. This increased the utilization of the x-ray beam by 30 times, compared with the single detector used per slice in first-generation systems. A relatively narrow fan angle of 10 degrees was used. In principle, a reduction in scan time of about 30-fold could be expected. However, this reduction time was not realized, because more data (600 rays X 540 views = 324,000 data points) were acquired to improve image quality. The shortest scan time with a second-generation scanner was 18 seconds per slice, 15 times faster than with the first-generation system. 12 Computed Tomography Elias Estephan, Ph.D. Pencil beam geometry provides excellent x-ray scatter rejection. X-rays that are scattered away from the primary pencil beam are not measured (no detectors) Fan beam geometry linear x-ray detector and a divergent fan beam of x-rays. X-rays that are scattered in the same plane as the detector can be detected, but scattered out of plane are not detected. Scattered radiation accounts for approximately 5% of the signal in typical fan beam scanners. Open beam geometry conventional projection radiography highest detection of scatter (s/p=4). 13 Computed Tomography Elias Estephan, Ph.D. Third generation : rotate /rotate , wide fan beam before : At the end of each translation, the motion of the x-ray tube/detector system had to be stopped, the whole system rotated, and the translational motion restarted. more than 800 detectors the angle of the fan beam was increased so that the detector array formed an arc wide enough to allow the x-ray beam to interrogate the entire patient more expensive CT scanners. However, spanning the dimensions of the patient with an entire row of detectors eliminated the need for translational motion. The multiple detectors in the detector array capture the same number of ray measurements in one instant as was required by a complete translation in the earlier scanner systems. 14 Computed Tomography Elias Estephan, Ph.D. The mechanically joined x-ray tube and detector array rotate together around the patient without translation. The motion of third-generation CT is "rotate/rotate," referring to the rotation of the x-ray tube and the rotation of the detector array. By elimination of the translational motion, the scan time is reduced substantially. The early third-generation scanners could deliver scan times shorter than 5 seconds. Newer systems have scan times of one half second. The evolution from first- to second- and second- to third-generation scanners involved radical improvement with each step. Developments of the fourth and fifth-generation led not only to some improvements but also to some compromises in clinical CT images, compared with third-generation scanners. Indeed, rotate/rotate scanners are still as viable today 15 Computed Tomography Elias Estephan, Ph.D. Fourth generation : rotate/stationary Third-generation scanners suffered from ring artifacts Each detector has a certain amount of drift The rotate/rotate geometry of third-generation scanners leads to a situation in which each detector is responsible for the data corresponding to a ring in the image Detectors toward the center small in diameter, and more peripheral detectors larger diameter rings The detectors toward the center of the array make the transmission measurement It, while the reference detector that measures I0 is positioned near the edge of the detector array. If g1 is the gain of the reference detector, and g2 is the gain of the other detector, then the transmission measurement is 16 Computed Tomography Elias Estephan, Ph.D. true gain terms cancel each other out, and that happens when g1 = g2. If there is electronic drift g1≠g2. µt values that are backprojected cause the ring artifacts. Fourth-generation overcome the problem of ring artifacts. the detectors are placed in a stationary 360-degree ring around the patient requiring many more detectors. Modern fourth-generation CT systems use about 4,800 individual detectors. x-ray tube rotates and the detectors are stationary, fourth-generation CT is said to use a rotate/stationary geometry. 17 Computed Tomography Elias Estephan, Ph.D. During acquisition the divergent x-ray beam forms a fan-shaped x-ray beam. However, the data are processed for fan beam reconstruction with each detector as the vertex of a fan, the rays acquired by each detector being fanned out to different positions of the x-ray source. “third-generation design uses a source fan, whereas fourth-generation uses a detector fan”. The third-generation fan data are acquired by the detector array simultaneously, in one instant of time. The fourth-generation fan beam data are acquired by a single detector over the period of time that is required for the x-ray tube to rotate through the arc angle of the fan 18 Computed Tomography Elias Estephan, Ph.D. With fourth-generation geometry, each detector acts as its own reference detector. For each detector with its own gain, g, Note that the single g term in this equation is guaranteed to cancel out. ring artifacts are eliminated with modern detectors and more sophisticated calibration software, third-generation CT scanners are essentially free of ring artifacts as well. 19 Computed Tomography Elias Estephan, Ph.D. Fifth generation : stationary /stationary cardiac tomographic imaging. "cine-CT" no conventional x-ray tube; large arc of tungsten encircles the patient and lies directly opposite to the detector ring. X-rays electron beam strikes the tungsten. There are no moving parts to this scanner gantry. also called electron beam scanners, are marketed They are capable of 50-msec scan times and can produce fast-frame-rate CT movies of the beating heart. 20 Computed Tomography Elias Estephan, Ph.D. Sixth generation : helical the gantry had be stopped after each slice was acquired, because the detectors and/or the x- ray tube had to be connected by wires to the stationary scanner electronics. In the early 1990s, the design of third- and fourth-generation scanners evolved to incorporate slip ring technology. circular contact with sliding brushes that allows the gantry to rotate continually, untethered by wires. Helical CT (also inaccurately called spiral CT) scanners acquire data while the table is moving the x-ray source moves in a helical pattern around the patient being scanned. Helical CT scanners use either third- or fourth-generation slip-ring designs. helical scanning allows the use of less contrast agent 21 Computed Tomography Elias Estephan, Ph.D. In order to produce reconstructions of planar sections of the patient, the raw data from the helical data set are interpolated to approximate the acquisition of planar reconstruction data 22 Computed Tomography Elias Estephan, Ph.D. Seventh generation : multiple detector array X-ray tubes designed for CT have impressive heat storage and cooling capabilities, make better use of the x-rays that are produced by the x-ray tube. When multiple detector arrays are used the collimator spacing is wider and therefore more of the x-rays are used. With single detector array, opening up the collimator the slice thickness↗ good for improving the utilization of the x-ray beam but spatial resolution ↘ in the slice thickness multiple detector arrays the slice thickness is determined by the detector size and not by the collimator 23 Computed Tomography Elias Estephan, Ph.D. For example, for four contiguous, 5-mm detector arrays and 20-mm collimator spacing the number of x-rays being detected is four times that of a single detector array with 5-mm collimation. Furthermore, the data set from the 4 X 5 mm multiple detector array can be used to produce true 5-mm slices, true 10-, 15-, or even 20-mm slices, all from the same acquisition. 24 Computed Tomography Elias Estephan, Ph.D. 3. Detectors and detector arrays Xenon detectors Xenon detectors use high-pressure nonradioactive xenon gas, in long thin cells between two metal plates Although a gaseous detector does not have the same detection efficiency as a solid one, the detector can be made very thick (e.g., 6 cm) to compensate in part for its relatively low density. 25 Computed Tomography Elias Estephan, Ph.D. septa can also be made quite thin improves the geometric efficiency by reducing dead space between detectors. long and thin are highly directional must be positioned in a fixed orientation with respect to the x-ray source. cannot be used for fourth-generation scanners (only for third-generation systems) Xenon detectors for CT are ionization detectors. a gaseous volume is surrounded by two metal electrodes, with a voltage applied across the two electrodes As x-rays interact with the xenon atoms and cause ionization, the electric field between the plates causes the ions to move to the electrodes, where the electronic charge is collected. The electronic signal is amplified and then digitized, and its numerical value is directly proportional to the x-ray intensity striking the detector 26 Computed Tomography Elias Estephan, Ph.D. Solid-state detectors composed of a scintillator coupled tightly to a photodetector (typically a photodiode) x-rays visible light electrical signal The detector size in CT is measured in millimeters (typically 1.0 X 15 mm or 1.0 X 1.5 mm for multiple detector array scanners), 27 Computed Tomography Elias Estephan, Ph.D. The scintillator used in solid-state CT detectors varies among manufacturers, with CdW04, yttrium and gadolinium ceramics…….. Because the density and effective atomic number of scintillators are substantially higher than those of pressurized xenon gas, have better x-ray absorption efficiency. to reduce crosstalk between adjacent detector elements, a small gap between detector elements is necessary, and this reduces the geometric efficiency somewhat. The top surface of a solid-state CT detector is essentially flat and therefore is capable of x-ray detection over a wide range of angles, unlike the xenon detector. Solid-state detectors are required for fourth-generation CT scanners, and they are also used in third-generation scanners as well. 28 Computed Tomography Elias Estephan, Ph.D. Multiple detector arrays Multiple detector arrays are a set of several linear detector arrays, tightly abutted The multiple detector array is an assembly of multiple solid-state detector array modules. With a traditional single detector array CT system, the detectors are quite wide (e.g., 15 mm) and the adjustable collimator determines slice thickness, typically between 1 and 13 mm. With multiple detector arrays, slice width is determined by the detectors, not by the collimator (although a collimator does limit the beam to the total slice thickness). 29 Computed Tomography Elias Estephan, Ph.D. with multislice systems, the slice width is determined by grouping one or more detector units together. For one manufacturer, the individual detector elements are 1.25 mm wide, and there are 16 contiguous detectors across the module. The electronics are available for four detector array channels, and one, two, three or four detectors on the detector module can be combined to achieve slices of 4 X 1.25 mm, 4 X 2.50 mm, 4 X 3.75 mm, or 4 X 5.00 mm. Other manufacturers use a gradually increasing spacing, with detector widths of 1.0, 1.5, 2.5, and 5.0 mm going away from the center. third-generation multiple detector array 16 detectors in the slice thickness dimension and 750 detectors along each array 12,000 individual detector elements are used. The fan angle is about 60 degrees fourth-generation six times as many detectors as third-generation systems all currently planned multiple detector array scanners make use of third-generation geometry. 30 Computed Tomography Elias Estephan, Ph.D. 4. Details of acquisition Slice thichness : single detector array scanners Opening the collimation beyond the width of the detectors would do nothing to increase slice thickness, but would increase both the dose to the patient and the amount of scattered radiation. There are important tradeoffs with respect to slice thickness. For scans performed at the same kV and mAs, the number of detected x-ray photons increases linearly with slice thickness. Also signal to noise ratio ↗ Larger slice thicknesses yield better contrast resolution (higher SNR) with the same x-ray techniques, but the spatial resolution is reduced. For thin slices, the mAs of the study protocol usually is increased to partially compensate for the loss of x-ray photons resulting from the collimation. 31 Computed Tomography Elias Estephan, Ph.D. slice sensitivity profile. consequence of the finite width of the x-ray focal spot, the penumbra of the collimator, the fact that the image is computed from a number of projections………… Furthermore, helical scans have a slightly broader slice sensitivity profile the same object at different locations in the slice volume (e.g., positions A, B, C, and D) will produce different CT numbers in the image. small object is placed in the center of the CT slice, it produces greater contrast 32 Computed Tomography Elias Estephan, Ph.D. Slice thickness : multiple detector array scanners Multiple detector arrays can be used both in conventional axial scanning and in helical scanning protocols. Multiple detector array T scanners acquire several slices simultaneously (four slices shown here), and the x-ray beam collimation determines the outer two edges of the acquired slices. Slice thickness is determined in the multiple detector array scanner by binning different numbers of detector subunits together and by physically moving the collimator to the outer edges of all four slices. (no blurring) This causes the radiation dose to be a bit higher (penumbra)in multislice scanners, but it reduces artifacts by equalizing the slice sensitivity profiles between the detector arrays. 33 Computed Tomography Elias Estephan, Ph.D. Detector Pitch and collimator Pitch For helical scan protocols For one detector array, the pitch is determined by the collimator (collimator pitch) and is defined as: it fundamentally influences radiation dose to the patient, image quality, and scan time. A pitch of less than 1.0 involves overscanning, which may result in some slight improvement in image quality and a higher radiation dose to the patient. pitches up to 1.5 are commonly used. Pitches with values greater than 1.0 The benefit is faster scan time, less patient motion, and, in some circumstances, use of a smaller volume of contrast agent. 34 Computed Tomography Elias Estephan, Ph.D. For multiple detector arrays The collimator pitch defined previously is still valid, and collimator pitches range between 0.75 and 1.5 The detector pitch is also a useful concept for multiple detector array scanners, and it is defined as: For a multiple detector array scanner with N detector arrays, the collimator pitch is as follows: For scanners with four detector arrays, detector pitches running from 3 to 6 are used. A detector pitch of 3 for a four-detector array scanner is equivalent to a collimator pitch of 0.75 = (3/4), and a detector pitch of 6 corresponds to a collimator pitch of 1.5 (6/4). 35 Computed Tomography Elias Estephan, Ph.D. For example, with a 4-slice MDCT at 4 × 1.25- mm slice thickness and a tablefeed of 6 mm per rotation, the pitch is 1.2. 36 Computed Tomography Elias Estephan, Ph.D. Changing the pitch will affect the MDCT scan acquisition in the same ways it affects SDCT. To review, increasing the pitch will shorten the total acquisition time for a given distance covered, although there will be some penalty in image noise related to fewer data being acquired (i.e., undersampling). Again, this effect is usually small at pitch levels less than 1.5. Increasing pitch will also decrease radiation dose to the patient provided the technique remains constant. Extended pitch values may result in an increase in streak artifact. 37 Computed Tomography Elias Estephan, Ph.D. 5. TOMOGRAPHIC RECONSTRUCTION Rays and Views: The Sinogram The data acquired for one CT slice can be displayed before reconstruction sinogram The horizontal axis of the sinogram corresponds to the different rays in each projection. The vertical axis in the sinogram represents each projection angle. A state-of-the-art CT scanner may acquire approximately 1,000 views with 800 rays per view, resulting in a sinogram that is 1,000 pixels tall and 800 pixels wide, corresponding to 800,000 data points. 38 Computed Tomography Elias Estephan, Ph.D. The number of rays used to reconstruct a CT image has a profound influence on the radial component of spatial resolution, and the number of views affects the circumferential component of the resolution. CT images of a simulated object reconstructed with differing numbers of rays show that reducing the ray sampling results in low-resolution, blurred images. 39 Computed Tomography Elias Estephan, Ph.D. CT images of the simulated object reconstructed with differing numbers of views show the effect of using too few angular views (view aliasing). The view aliasing is mild with 240 views and becomes pronounced with 60 views. Aliasing 40 Computed Tomography Elias Estephan, Ph.D. Processing of the data The raw data acquired by a CT scanner is preprocessed before reconstruction. correction data that are used to adjust the electronic gain of each detector in the array. Variation in geometric efficiencies caused by imperfect detector alignments is also corrected. in fourth-generation scanners the x-ray source rotates in an arc around each of the detectors -the angle and the distance between the detector and the x-ray tube change over this arc - These geometric efficiencies are measured during calibration scans and stored in the computer after the digital data are calibrated, the logarithm of the signal is computed by the following equation µt is the total attenuation coefficient for each ray 41 Computed Tomography Elias Estephan, Ph.D. µt can be broken down into its components in each small path length ∆t: The constant ∆t factors out, resulting in the following the reconstructed value in each pixel is the linear attenuation coefficient for the corresponding voxel. 42 Computed Tomography Elias Estephan, Ph.D. Interpolation (Helical) before the actual CT reconstruction the helical data set is interpolated into a series of planar image data sets interpolationcomputation Helical scanning allows the production of additional overlapping images with no additional dose to the patient. 43 Computed Tomography Elias Estephan, Ph.D. The sensitivity of the CT image to objects not centered in the voxel is reduced subtle lesions, which lay between two contiguous images, may be missed. With helical CT scanning, interleaved reconstruction allows the placement of additional images along the patient, so that the clinical examination is almost uniformly sensitive to subtle abnormalities. Interleaved reconstruction adds no additional radiation dose to the patient, but additional time is required to reconstruct the images. 44 Computed Tomography Elias Estephan, Ph.D. It is important not to confuse the ability to reconstruct CT images at short intervals along the helical data set with the axial resolution itself. The slice thickness dictates the actual spatial resolution along the long axis of the patient. For example, images with 5-mm slice thickness can be reconstructed every 1 mm, but this does not mean that 1-mm spatial resolution is achieved. It simply means that the images are sampled at 1-mm intervals. 45 Computed Tomography Elias Estephan, Ph.D. Simple Backprojection Reconstruction Once the image raw data have been preprocessed, the final step is to use the planar projection data sets (i.e., the preprocessed sinogram) to reconstruct the individual tomographic images. Iterative reconstruction techniques filtered backprojection 46 Computed Tomography Elias Estephan, Ph.D. 47 Computed Tomography Elias Estephan, Ph.D. 48 Computed Tomography Elias Estephan, Ph.D. Simple backprojection is a mathematical process, based on trigonometry, which is designed to emulate the acquisition process in reverse. 49 Computed Tomography Elias Estephan, Ph.D. 50 Computed Tomography Elias Estephan, Ph.D. CT numbers or Hounsfield Units after CT reconstruction, each pixel in the image is represented by a high-precision floating point number that is useful for computation but less useful for display. CT images are normalized and truncated to integer values. The number CT(x,y) in each pixel, (x,y), of the image is converted using the following expression: where µ(x,y) is the floating point number of the (x,y) pixel before conversion, µwater is the attenuation coefficient of water, and CT(x,y) is the CT number that ends up in the final clinical CT image. The value of µ water is about 0.195 This normalization results in CT numbers ranging from about -1,000 to +3,000, where -1,000 corresponds to air, soft tissues range from -300 to -100, water is 0, and dense bone and areas filled with contrast agent range up to +3,000. 51 Computed Tomography Elias Estephan, Ph.D. 6. Digital image display Windowing and Leveling The level is the CT number corresponding to the center of the window. A narrow window produces a very high contrast image, corresponding to a large slope on the figure. CT numbers < P1 black ; CT numbers > P2 white. P1 < CT < P2 will be displayed in a meaningful manner. 52 Computed Tomography Elias Estephan, Ph.D. Multiplanar Reconstruction Coronal or sagittal multi planar reconstructions (MPRs) can be produced from a series of axial CT scans. For instance, in a sagittal MPR image the vertical pixel spacing (∆y) is equivalent to the spacing of the pixels on the axial image. The horizontal spacing (∆x) corresponds to the distance between the reconstructed images. Because ∆Y is usually much smaller than ∆x, it is common to interpolate image data in the horizontal dimension to produce square pixels. 53 Computed Tomography Elias Estephan, Ph.D. Helical Scan Coverage The formula for determining how many images are created and how much anatomy (lengthwise) is covered in a helical scan acquisition builds on the formula used to calculate distance covered in an axial scan sequence. 54 Computed Tomography Elias Estephan, Ph.D. 55 Computed Tomography Elias Estephan, Ph.D. 7. radiation dose The distribution of radiation dose is different than in radiography First, because a single CT image is acquired in a highly collimated manner, the volume of tissue that is irradiated by the primary x-ray beam is substantially smaller Second, the volume of tissue that is irradiated in CT is exposed to the x-ray beam from almost all angles. In radiography entrance beam experiences exponentially more dose than the tissue near the exit surface of the patient. Finally, CT acquisition requires a high SNR to achieve high contrast resolution, and therefore the radiation dose to the slice volume is higher because the techniques used (kV and mAs) are higher. 56 Computed Tomography Elias Estephan, Ph.D. Dose Measurement the radiation dose attributable to scattered radiation is considerable, and it can be higher than the radiation dose from the primary beam. CT slice delivers a considerable dose from scatter to adjacent tissues, outside the primary beam. The tissue in slice 5 will receive both primary and scattered radiation from its acquisition, but it will also receive the scattered radiation dose from slices 4 and 6, and to a lesser extent from slices 3 and 7, and so on 57 Computed Tomography Elias Estephan, Ph.D. The multiple scan average dose (MSAD) is the standard for determining radiation dose in CT. includes the dose attributable to scattered radiation emanating from all adjacent slices. defined as the average dose, at a particular depth, resulting from a large series of CT slices. measured directly by placing a small exposure meter at a point, in the middle of the slices, in a dose phantom, large series of CT scans over the slices , and adding the doses from all slices. An estimate of the MSAD (contiguous slices) single scan by measuring the CT dose index (CTDI). The CTDI is defined by the U.S. Food and Drug Agency the radiation dose to any point in the patient including the scattered radiation contribution from 7 CT slices in both directions, for a total of 14 slicesCTDlFDA 58 Computed Tomography Elias Estephan, Ph.D. CTDIFDA is measured with many small thermoluminescent dosimeters (TLDs) placed in holes along 14-slice-thickness increments in a Lucite dose phantom. A single CT image is acquired at the center of the rod containing the TLDs, and the CTDIFDA is determined from this single CT scan by summing the TLD dose measurements. 59 Computed Tomography Elias Estephan, Ph.D. Medical physicists usually measure the CTDI with the use of a long (l00 mm), thin pencil ionization chamber. 100mm ≈ 14 contiguous 7-mm CT scans good estimation of the CTDIFDA for 7- and 8-mm slices. A single CT image is acquired at the center of the pencil chamber To calculate the CTDI, all of the energy deposition along the length of the ion chamber is assigned to the thickness of the CT slice: T is the slice thickness the length of the air-kerma-to-dose (mm). pencil ion chamber (mGy/mGy) or exposure-to-dose (mGy/R or rad/R) X is the measured air kerma (mGy) or exposure (R) to the conversion factor, pencil ion chamber demonstrated : equivalent to using a small exposure meter and acquiring all 14 CT scans. The 100-mm pencil ion chamber cannot be used to measure the CTDlFDA for slice thicknesses other than 7 mm. it is commonly used for all slice thicknesses CTDI100 60 Computed Tomography Elias Estephan, Ph.D. The CTDIFDAunderestimates the MSAD for small slice thicknesses (e.g., 2 mm) significant amount of radiation is scattered beyond seven slice thicknesses. The CTDI100 provides much better estimate of the MSAD for thin slices. standard phantoms :Holes are drilled allow the placement of the pencil chamber Lucite rods are used to plug the holes not occupied by the pencil chamber. The pencil ionization chamber measures air kerma the conversion factor is approximately 0.893 mGy/mGy (air kerma), or 0.78 rad/roentgen. The dose to soft tissue is about 20% larger than the dose to Lucite. radiation dose in CT is proportional to the mAs used per slice. 61 Computed Tomography Elias Estephan, Ph.D. Dose Considerations in Helical Scanning For CT scanners with multiple detector arrays, the collimator pitch (not the detector pitch) should be used for dose calculations. The dose in helical CT is calculated in exactly the same manner as it is with axial CT, using the CTDI; however a correction factor is needed when the pitch is not 1.0: is 67% that of the helical dose =133% of dose from the axial dose conventional scanning similar to performing a conventional (nonhelical) axial scan with contiguous slices This is correct if we assume that the same mAs was used. helical scans often use less mAs per 360-degree gantry rotation than axial scans do 62 Computed Tomography Elias Estephan, Ph.D. Current Modulation in Computed Tomography scanners capable of modulating the mA during the scan. it takes fewer x-ray photons (lower mA) to penetrate thinner tissue, and more x-ray photons (higher mA) are needed to penetrate thicker projections through the body. The SNR in the final image is related to the number of x-rays that pass through the patient and are detected. the high SNR detected in the thinner angular projections is essentially wasted, because the noise levels from the thicker angular projections dominate in the final image. reduction in patient dose can be achieved with little loss in image quality by reducing the mA during acquisition through the thinner tissue projections. Other benefit: x-ray tube loading is reduced and helical scans can be performed for longer periods and with greater physical coverage 63 Computed Tomography Elias Estephan, Ph.D. Dual-Source CT A new CT technology was introduced to U.S. hospitals in 2006. Known as dual-source CT, the new design uses two sets of x-ray tubes and two corresponding detector arrays in a single CT gantry. Figure 5-18 illustrates the theoretical design. In practical application, the second detector array is restricted by the space available in the gantry. Therefore, one detector array covers the entire scan field of view (approximately 50 cm), whereas the second detector is limited to a smaller, central field of view. 64 Computed Tomography Elias Estephan, Ph.D. Figure 5-19 illustrates the actual design. With the two tubes and detectors mounted at right angles, both helical acquisitions run simultaneously. 65 Computed Tomography Elias Estephan, Ph.D. The idea of the dual-source CT is not new. The concept was attempted years ago on single-slice scanners, but the early helical scan technology limited its application. Siemens Medical Solutions (Forchheim, Germany) resurrected the idea to produce the Somatom Definition CT scanner. The primary goal of the dual-source design is increased scan speed. 66 Computed Tomography Elias Estephan, Ph.D. A second potential advantage of the dual-source CT system arises from the fact that the two x- ray tubes, although working simultaneously, have the ability to produce x-ray photons possessing different energies. That is, for a given scan each x-ray tube can use a different kVp setting. Recall that beam attenuation is caused by absorption and scattering of radiation by the object scanned. The attenuation is dependent not just on the density of the object scanned but also on the energy of the x-ray photons. Thus, additional information can be learned about the object scanned when two x-ray energies are used and the difference in attenuation is analyzed. This works particularly well in materials with high atomic numbers, such as iodine. Theoretically, this strategy can be used to differentiate iodine from other dense materials. Another idea is to use the dual-energy concept to differentiate body tissues without the application of contrast media. However, because soft tissues do not possess high atomic numbers this is likely to be more difficult to achieve. 67 Computed Tomography Elias Estephan, Ph.D. 8. Image quality the limiting spatial frequency for screen-film radiography is about 7 line pairs (lp) per millimeter and for digital radiography it is 5 Ip/mm, for CT is approximately 1 Ip/mm. It is the contrast resolution of CT that distinguishes the modality: CT has, by far, the best contrast resolution of any clinical x-ray modality. contrast resolution tied to the SNR related to the number of x-ray quanta used per pixel If pixel size ↘ (same FOV, same dose) spatial resolution↗ the number of x-rays per pixel ↘ contrast↘. Compromise between spatial resolution and contrast resolution. In CT there is a well-established relationship among SNR, pixel dimensions (∆), slice thickness (T), and radiation dose (D): 68 Computed Tomography Elias Estephan, Ph.D. Factors Affecting Spatial Resolution Number of views: Use of too few views results in view aliasing Number of rays: For a fixed FOV, the number of rays increases as the detector pitch decreases Detector pitch Detector aperture: The detector aperture is the width of the active element of one detector Object magnification: Increased magnification amplifies the blurring of the focal spot Magnification factors of 2.0 are common (2.7 for the entrant surfaces of large patients) Focal spot size: As in any x-ray imaging procedure Slice thickness: Slice sensitivity profile: The slice sensitivity profile is quite literally the line spread function in the cranial-caudal axis of the patient slice thickness. Helical pitch: with greater pitches resolution↘. 69 Computed Tomography Elias Estephan, Ph.D. Reconstruction kernel: The shape of the reconstruction kernel has a direct bearing on spatial resolution Pixel matrix Patient motion Field of view 70 Computed Tomography Elias Estephan, Ph.D. Factors Affecting Contrast Resolution mAs: number of x-ray SNR and the contrast resolution. mAs ↗SNR ↗contrast↗ Dose: The dose increases linearly with mAs per scan Pixel size (FOV): pixel dimensions ↗ number of x-rays passing through each pixel ↗. Slice thickness: Thicker slices use more photons and have better SNR. Reconstruction filter Patient size: larger patients attenuate more xrays detection of fewer x-rays SNR ↘ contrast ↘ Gantry rotation speed: faster gantry rotations mAs ↘ contrast ↘ 71 Computed Tomography Elias Estephan, Ph.D. 9. ARTIFACTS Beam Hardening CT uses a polyenergetic x-ray spectrum, with energies ranging from about 25 to 120 keV x-ray attenuation coefficients are energy dependent: After passing through a given thickness of patient, lower-energy x-rays are attenuated to a greater extent than higher-energy x-rays are. propagation the shape of the spectrum becomes skewed toward the higher energies Because the attenuation of bone is greater than that of soft tissue, bone causes more beam hardening than an equivalent thickness of soft tissue 72 Computed Tomography Elias Estephan, Ph.D. The beam-hardening phenomenon induces artifacts in CT because rays from some projection angles are hardened to a differing extent than rays from other angles sophisticated two-pass beam-hardening correction algorithms have been implemented by some manufacturers In a two pass algorithm, the image is reconstructed normally in the first pass, the path length that each ray transits through bone and soft tissue is determined from the first-pass Image then each ray is compensated for beam hardening based on the known bone and soft tissue thicknesses it has traversed. A second-pass image reconstruction is performed using the corrected ray values 73 Computed Tomography Elias Estephan, Ph.D. Motion artifacts Motion artifacts occur when the patient moves during the acquisition. Small motions cause image blurring, and larger physical displacements during CT image acquisition produce artifacts that appear as double images In some cases, the patient needs to be rescanned. 74 Computed Tomography Elias Estephan, Ph.D. Partial Volume Averaging The CT number in each pixel is proportional to the average µ in the corresponding voxel. For voxels containing all one tissue type (e.g., all bone, all liver), µ is representative of that tissue. Some voxels in the image, however, contain a mixture of different tissue types µ is a weighted average of the two different µ values. Partial volume averaging is most pronounced for softly rounded structures that are almost parallel to the CT slice. Partial volume artifacts can lead to misdiagnosis when the presence of adjacent anatomic structures is not suspected 75 Computed Tomography Elias Estephan, Ph.D. There are several approaches to reducing partial volume artifacts, and the obvious a priori approach is to use thinner CT slices. When a suspected partial volume artifact occurs with a helical study it is sometimes necessary to use the raw data to reconstruct additional CT images at different positions. Many institutions make use of interleaved reconstructions (e.g., 5-mm slices every 2.5 mm) as a matter of routine protocol. 76 Computed Tomography Elias Estephan, Ph.D. Changing Slice Incrementation Retrospectively Because helical scans result in a block of data, a choice can be made as to where in that block an image is created. This flexibility allows the creation of overlapping slices retrospectively. It does this without increasing the radiation dose to the patient, as is the case when axial images are scanned in an overlapping fashion. 77 Computed Tomography Elias Estephan, Ph.D. In addition to creating overlapping images, changing slice incrementation offers other advantages. In some cases it may help reduce the partial volume effect. Figure 5-16A represents a volume of helical data taken on an SDCT system with a 10-mm slice thickness. Initially, one image is produced for each 10 mm of data. On images 2 and 3, a lesion is seen. It is suspected that the partial volume effect may be producing an inaccurate representation of this lesion. As Figure 5-16A illustrates, at least 5 mm of normal tissue is being averaged with the Lesion Because this data are acquired in a helical fashion, we can retrospectively change the portion of data used, which produces a new image. 78 Computed Tomography Elias Estephan, Ph.D. In this example, an image is created from data at table positions 15 through 25. Figure 5-16B demonstrates that image 6 will contain the entire lesion in a single slice, rather than straddling two separate slices. In this way, the partial volume effect can be reduced or eliminated, resulting in a more accurate image. Images can be reconstructed with a very small data incrementation (