Chapter 12 - Membrane Transport PDF
Document Details
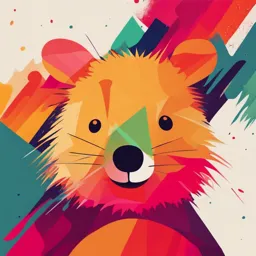
Uploaded by IncredibleNephrite
Queens College, CUNY
Tags
Summary
This document covers Chapter 12 on membrane transport. It explains different processes of transport across cell membranes and includes figures and diagrams illustrating the concepts. The document explains different mechanisms to move substances across membranes.
Full Transcript
Chapter 12 Membrane Transport Lipid bilayer is ideally suited to prevent loss of charged & polar solutes (ions, sugars, AA) Must allow movement of nutrients, respiratory gases, hormones, wastes, etc. Fig. 12-1 Size and solubility determines rate of simple diffusion across protein-free bilayer •...
Chapter 12 Membrane Transport Lipid bilayer is ideally suited to prevent loss of charged & polar solutes (ions, sugars, AA) Must allow movement of nutrients, respiratory gases, hormones, wastes, etc. Fig. 12-1 Size and solubility determines rate of simple diffusion across protein-free bilayer •membranes are semipermeable •Membranes are a billion times more permeable to water than to small ions Fig. 12-2 Inside Outside •Approximately equal quantities of +/- charges inside and outside of cell •Tiny excesses of + or - charges do occur near PM and have imp. electrical effects Table 12-1 Remember a salty banana Na+ClK+ Each cell membrane has its own characteristic set of carrier proteins Figure 12-8 Transporters and channels move inorganic ions and small, polar molecules cross membrane — facilitated diffusion A — channels either open or close; faster transport rates than transporters B — transporters undergo a series of conformational changes (alternate access) Fig. 12-3 Membranes are a selectively permeable barrier - two means for movement both of which lead to net flux of ions/compounds (influx - into cell; efflux out of cell) 1. Passively by diffusion 2. Actively by energy-coupled transport process Diffusion •substance moves from region of high conc. to region of low conc.; eventually eliminating conc. difference •driven by random thermal motion leading to increase in entropy •electrochemical gradient --chemical - compartment concentration difference -- electrical - compartment charge difference An electrochemical gradient has two components Width of green arrow -- magnitude of gradient Fig. 12-5 Different processes by which substances move across membranes 1. Simple diffusion through lipid bilayer 2. Facilitate transport through aqueous, protein-lined channel; “channels” 3. Facilitated transporters; “transporters” 4. Active transport; “pumps” 1. Diffusion through membranes •must be concentration difference •must be membrane permeable •directly through lipid -- partition coefficient - measure of polarity; ratio of solubility in nonpolar solvent/water -- molecule size (smaller is faster) Size of letter denotes concentration 1. Diffusion through membranes •must be concentration difference •must be membrane permeable •directly through lipid -- partition coefficient - measure of polarity; ratio of solubility in nonpolar solvent/water -- molecule size (smaller is faster) Size of letter denotes concentration Different processes by which substances move across membranes 1. Simple diffusion through lipid bilayer 2. Facilitated transport through aqueous, protein-lined channel; “channels” 3. Facilitated transporters; “transporters” 4. Active transport; “pumps” Diffusion of ions through membrane channels •Ions (e.g., Na+, K+, Ca2+, & Cl-) cannot pass freely through the lipid bilayer but must still enter and exit cells. • ion channels Diffusion of ions through membrane channels •transport is passive and proceeds down the concentration gradient of the ion being transported •Usually 107 - 108 ions/s •gated -- open/close with the proper stimulus • bidirectional -- depending on the gradient, channels may transport ions in either/both of the two possible directions. Figure 12-20 Ions in solution are “hydrated” by water molecules Potassium channel has a selectivity filter for ion specificity Figure 12-19 Potassium channel Unsolvated = water removed Major categories of gated ion channels 1. Voltage-gated channels -- opens due to change in voltage difference 2. Ligand-gated channels -- opens due to ligand binding; usually not the transported species 3. Stress (mechanically) gated -- opens due to mechanical stimulation Figure 12-27 1. Voltage gated 2. Ligand gated Neurotransmitter receptor -- a ligand gated ion channel Fig. 1 2 -4 2 Neurotransmitter receptor -- a ligand gated ion channel Fig. 1 2 -4 3 3. Mechanically (Stress) gated Stretch-activated Piezo channels respond to light touch Ion-conducting pore • • Channels in skin allow us to respond to light touch Channels in bladder cells detect when the bladder is full Mechanically-activated ion channels allow us to hear Fig. 1 2 -2 8 Stress-gated ion channels allow us to hear Different processes by which substances move across membranes 1. Simple diffusion through lipid bilayer 2. Facilitate transport through aqueous, protein-lined channel; “channels” 3. Facilitated transporters; “transporters” 4. Active transport; “pumps” 3. Facilitated transporters •binds to membrane-spanning protein that changes its shape; facilitative transporter •passive -- doesn’t require energy •bidirectional -- depends on conc. •102 - 104 molecules/s •molecule-specific; (AA, sugars) • activity regulatable Facilitated diffusion •Solute binding on one side of membrane may or may not be required to change protein shape, exposing solute to other surface (Example) Glucose transporter -- facilitated diffusion of glucose Outward open Inward open •Changes shape randomly even without glucose; glucose binding induces shape change •Side of membrane with highest glc conc. will tend to bind most glc •If glc higher outside, will move glc inward and vice versa Fig. 1 2 -9 (Example) Glucose transporter -- facilitated diffusion of glucose into muscle cell •down concentration gradient (High --> Low) •14 related transporters (isoforms; GLUT 1-14) in humans •Glucose in cell then phosphorylated to glc6-phosphate, which cannot bind the transporter and stays in the cell See Fig. 1 2 -9 Example of regulation of a transporter • á glucose levels triggers insulin secretion by pancreas • á glucose uptake into various cells (e.g., skeletal muscle and fat cells) •Absence of insulin ~5% transporters on PM •Presence of insulin ~50% transporters on PM Different processes by which substances move across membranes 1. Simple diffusion through lipid bilayer 2. Facilitate transport through aqueous, protein-lined channel; “channels” 3. Facilitated transporters; “transporters” 4. Active transport: “pumps” Active transport •uses energy to move molecules “up” conc. gradient (Low --> High) •energy source may be ATP hydrolysis, light absorbance or electron transfer, etc. •uses molecule-specific transmembrane proteins; usually called “pumps” Maintenance requires active transport O u tside I nside D irectio n chem. gra dient [ K +] ~5 mM ~140 mM K + io ns o ut [ N a +] ~145 mM ~5 mM N a + io ns in [~145 mM] [~5 mM] Na+/K+-ATPase (aka Na + pump) [~5 mM] ~[140 mM] Fig. 12-11 Na+/K+-ATPase changes conformation as it exchanges Na+ for K+ Fig. 12-12 Na+/K+-ATPase •Pump is unidirectional; pumps Na+ ions out & K+ ions in •phosphorylation-induced shape change alters ion affinity •If 3 Na+ ions out for every 2 K+ ions in, what does this do to net charge on each side of the membrane? •As the pump operates, it makes the cell interior more negative •electrogenic -- contributes to charge differences across PM An example of active transport --Na+/K+-ATPase •pump changes shape when autophosphorylated •establish steep gradients needed for nerve-muscle impulses •uses 1/3 of most animal cells' energy (2/3 in nerve cells) •Think of the pump keeping internal Na+ low, (not keeping external high) ~100 cycles/sec Purpose of ship’s pump is not to keep ocean full of water, but ship inside dry •functions like bilge pump on a leaky ship; constantly pumping out Na+ that came in thru channels or carrier proteins Na+ outside of cell is like water behind a dam •gradient can be used to transport other molecules •Humans have hundreds of different transporters that use this Na+ gradient to move specific molecules against their concentration gradients Fig. 12-13 How do cell use this sodium gradient? First, we need to discuss coupled transport Transporters can function as uniports, symports, or antiports Glucose transporter Fig. 12-15 3 types of carrier-mediated transport Electrochemical Na+ gradient drives coupled transporters Na+/glucose cotransporter Na+/H+exchanger Glucose transporter Figure 12-15 Glucose-Na+ symport protein uses the electrochemical Na+ gradient to drive the import of glucose • Unlikely to close unless entirely empty or entirely full • Glucose is “dragged” into cell along with Na+ Figure 12-16 Cotransport: Coupling active transport to existing ion gradients • Na+ moves glucose up its gradient as Na+ flows down its gradient across apical membrane (2° active transport system) •Transport protein (Na+/glucose cotransporter) binds 2 Na+ ions & 1 glucose molecule on outer apical surface •Movement of 2 moles of Na+ ions into a cell can generate a glucose conc. that is 30,000 times higher inside than out •Actually, 2 Na+ per 1 glucose Two types of glucose transporters allow gut epithelial cells to transport glucose from intestine to blood Figure 12-17 Academic.brooklyn.cuny.edu/biology/bio4fv Membrane potentials and nerve impulses •Nerve cells (neurons) specialized for the transmission of information, which is coded in the form of fast-moving electrical impulses •electricity in metals is carried by electrons, electricity in aqueous solutions is carried by ions (positive cations and negative anions) Membrane Potential •Thin (<1nm) layer of ions (~ 10 ions-thick) •When ions of one type cross membrane, charge difference creates membrane potential Fig. 1 2 -2 2 Membrane potential established largely by potassium leak channels •Membrane potential is determined by K+ gradient •Electrochemical gradient for K+ is zero •In animals resting potential is -20 to -200mV (inside negative) •If a Na+ channel were to open, a new potential would be reached •Membrane potential is determined by state of ion channels and ion concentrations Figure 12-23 Resting potential K+ leak channels (–) inside cell because: 1. Na+/K+-ATPase pumps more Na+ out than K+ in (10% contribution) 2. K+ ions leak out; excess (+) outside favors K+ remaining inside; balances; (90% contribution) 3. (–) proteins trapped inside Nerve cell structure -- some terminology Axon hillock Figure 12-31 Resting potential in a neuron •inside of PM (–) 60-70 mV relative to outside •magnitude and direction determined by differences in [ion] and permeability's Squid giant axon 1 mm wide (~width of pencil line) The axon is big, not the squid— so it is not the giant squid axon, and you needn’t be afraid. Fig. 12-34 –70 •Learned that of all the ions in seawater that Na+ and K+ levels needed for an action potential •resting potential determined by [K+] and height of action potential voltage change determined by extracellular [Na+] Fig. 12-33 •Resting potential of axon -60-70 mV (neg. inside) •Voltage-gated sodium channel — opens at -40 mV (so closed in resting axon) •Voltage-gated potassium channel — opens at +40 mV (so closed in resting axon) Action potential 1. K+ channels open Na+ Fig. 12-36 channels open stimulus causes opening of Na+ channels causing depolarization of membrane (gets more (+)) (if small amt, then rapidly returns to resting potential) 2. if depolarization reaches -40 mV threshold, voltage-gated Na+ channels open & Na+ rushes in; depolarization to +40 mV; all-or-none law 3. At +40 mV Na+ stops entering because electrical gradient and conc. gradient balance 4. 1 msec later, Na+ channels inactivated and voltagegated K+ channels open to let K+ out and -60 mV returns Action potential propagation and voltage-gated channels •total % of cell’s ions doesn’t change much •Internal Na+ is mM, one action potential lets in pM amounts of Na+ •many consecutive action potentials without Na+ /K+ pump requirement •in a 10 um3 cell 1/100,000 of internal K+ must flow out to change voltage by 100 mV Figure 12-36 Movement of action potential along an axon (nerve conduction) •Change in voltage in one part of axon triggers opening of channels in next part of axon •Does not weaken (decrease in size) as it travels •Note that it moves one direction. Why? Voltage-gated Na+ channel can change conformation depending on membrane potential •At +40 mV even though voltage would keep channel open, it becomes inactivated •Na+ stops entering cell and channel cannot be reopened initially Figure 12-37 Voltage-gated Na+ channel change conformation during an action potential Propagation of action potential – the nerve impulse Na+ channels inactivated --propagates up to 1 m/s Propagation of an action potential •From onset of action potential to end of refractory period ~2 msec; thus, a neuron can produce 100’s per sec. Figure 12-38 Action potential Speed of action potential propagation is of the essence •the greater the axon diameter, the faster the impulse •wrapping axon with myelin sheath allows small axons to transmit faster (~20X or more) •action potential at one node triggers next node •Ion channels are concentrated at nodes •saltatory conduction Unmyelinated axon Sodium influx Sodium efflux Myelinated axon Neurotransmission -- jumping the synaptic cleft •neurons are connected to their target cells at junctions called synapses •presynaptic cells (neuron or sensory cell) and postsynaptic cells (neuron, muscle or gland cell) are separated by a synaptic cleft (example of ligand-gated ion channel at synapse and voltage-gated along axon) An example -- Neuromuscular junction Excites or Inhibits •Voltage-gated Ca++ channels at terminal open to increase cytosolic Ca++ •Increased Ca++ causes synaptic vesicles to fuse with PM •synaptic vesicles contain neurotransmitter Synapse: electrical -> chemical -> electrical transmission