Chapter 9: T-cell-Mediated Immunity PDF
Document Details
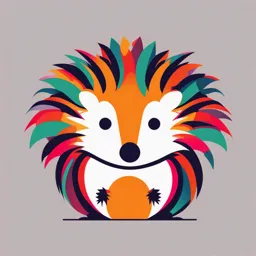
Uploaded by VigilantJasper
Tags
Summary
This chapter details the processes involved in T-cell-mediated immunity, including the activation, differentiation, and effector functions of T cells. It covers topics such as the development and function of secondary lymphoid organs, the role of dendritic cells in antigen presentation, and the various subsets of CD4 effector T cells. The chapter provides a comprehensive overview of T-cell responses against pathogens.
Full Transcript
PART IV the adaptive immune response 9 10 11 12 T-cell-Mediated Immunity The Humoral Immune Response Integrated Dynamics of Innate and Adaptive Immunity The Mucosal Immune System T-cell-Mediated Immunity An adaptive immune response is initiated when a pathogen overwhelms innate defense mechanisms...
PART IV the adaptive immune response 9 10 11 12 T-cell-Mediated Immunity The Humoral Immune Response Integrated Dynamics of Innate and Adaptive Immunity The Mucosal Immune System T-cell-Mediated Immunity An adaptive immune response is initiated when a pathogen overwhelms innate defense mechanisms. As the pathogen replicates and antigen accumulates, sensor cells of the innate immune system become activated to trigger the adaptive immune response. While some infections may be dealt with solely by innate immunity, as discussed in Chapters 2 and 3, host defense against most pathogens, almost by definition, requires recruitment of adaptive immunity. This is shown by the immunodeficiency syndromes that are associated with failure of particular parts of the adaptive immune response; these will be discussed in Chapter 13. In the next three chapters, we will learn how the adaptive immune response involving antigen-specific T cells and B cells is initiated and deployed. T-cell responses that lead to cellular immunity will be considered first, in this chapter; and B-cell responses that lead to antibody-mediated, or humoral, immunity will be considered in Chapter 10. In Chapter 11 we will consider the dynamics of T-cell and B-cell responses in the context of their integration with innate immunity and how this culminates in one of the most important features of adaptive immunity—immunological memory. Once T cells have completed their primary development in the thymus, they enter the bloodstream. On reaching a secondary lymphoid organ, they leave the blood to migrate through the lymphoid tissue, returning via the lymphatics to the bloodstream to recirculate between blood and secondary lymphoid tissues. Mature recirculating T cells that have not yet encountered their specific antigens are known as naive T cells. To participate in an adaptive immune response, a naive T cell must meet its specific antigen, presented to it as a peptide:MHC complex on the surface of an antigen-presenting cell, and be induced to proliferate and differentiate into progeny with new activities that contribute to removal of antigen. These progeny cells are called effector T cells and, unlike naive T cells, perform their functions as soon as they encounter ERRNVPHGLFRVRUJ 9 IN THIS CHAPTER Development and function of secondary lymphoid organs—sites for the initiation of adaptive immune responses. Priming of naive T cells by pathogen-activated dendritic cells. General properties of effector T cells and their cytokines. T-cell-mediated cytotoxicity. 346 Chapter 9: T-cell-Mediated Immunity their specific antigen on other cells—generally without requirement for further differentiation. Because of their requirement to recognize peptide antigens presented by MHC molecules, all effector T cells act on other host cells, not on the pathogen itself. The cells on which effector T cells act will be referred to as their target cells. On recognizing antigen, naive T cells differentiate into several functional classes of effector T cells that are specialized for different activities. CD8 T cells recognize pathogen peptides presented by MHC class I molecules, and naive CD8 T cells differentiate into cytotoxic effector T cells that recognize and kill infected cells. CD4 T cells have a more flexible repertoire of effector activities. After recognizing pathogen peptides presented by MHC class II molecules, naive CD4 T cells can differentiate down distinct pathways that generate effector subsets with different immunological functions. The main CD4 effector subsets are TH1, TH2, TH17, and TFH, which activate their target cells; and regulatory T cells, or Treg cells, which inhibit the extent of immune activation. Effector T cells differ from their naive precursors in ways that equip them to respond quickly and efficiently when they encounter specific antigen on target cells. Among the changes that occur are alterations in the expression of surface molecules that alter the patterns of migration of effector T cells, directing them to exit the secondary lymphoid tissues and move to sites of inflammation where pathogens have entered, or to B-cell zones within secondary lymphoid tissues, where they help generate pathogen-specific antibodies. The interactions with target cells in these sites are mediated both by direct T-cell–target cell contact and the release of cytokines, which can act locally on target cells; and at a distance to orchestrate the clearance of antigen. Some of the effector functions of T cells will be considered in this chapter; others will be discussed in Chapters 10 and 11 in the context of T-cell help for B cells and heightened activation of effector cells of the innate immune system. The activation and clonal expansion of a naive T cell on its initial encounter with antigen is often called priming, to distinguish this process from the responses of effector T cells to antigen on their target cells and the responses of primed memory T cells. The initiation of adaptive immunity is one of the most compelling narratives in immunology. As we will learn, the activation of naive T cells is controlled by a variety of signals. The primary signal that a naive T cell must recognize is antigen in the form of a peptide:MHC complex on the surface of a specialized antigen-presenting cell, as discussed in Chapter 6. Activation of the naive T cell also requires that it recognize co-stimulatory molecules that are displayed by antigen-presenting cells. Finally, cytokines that control differentiation into different types of effector cells are delivered to the activated naive T cell. All these events are set in motion by earlier signals that arise from the initial detection of the pathogens by the innate immune system. Microbe-derived signals are delivered to cells of the innate immune system by receptors such as the Toll-like receptors (TLRs), which recognize microbe-associated molecular patterns, or MAMPs, that signify the presence of nonself (see Chapters 2 and 3). As we will see in this chapter, these signals are essential to activate antigen-presenting cells so that they are able, in turn, to activate naive T cells. By far the most important antigen-presenting cells in the activation of naive T cells are dendritic cells, whose major function is to ingest and present antigen. Tissue dendritic cells take up antigen at sites of infection and are activated as part of the innate immune response. This induces their migration to local lymphoid tissue and their maturation into cells that are highly effective at presenting antigen to recirculating naive T cells. In the first part of this chapter we will consider the development and organization of secondary lymphoid tissues and discuss how naive T cells and dendritic cells meet in these sites to initiate adaptive immunity. ERRNVPHGLFRVRUJ Development and function of secondary lymphoid organs—sites for the initiation of adaptive immune responses. Development and function of secondary lymphoid organs—sites for the initiation of adaptive immune responses. As discussed in Chapter 8, the primary lymphoid organs—the thymus and bone marrow—are the tissue sites where antigenic receptor repertoires of T and B cells, respectively, are selected. Adaptive immune responses are initiated in the secondary lymphoid organs—lymph nodes, spleen, and the mucosa-associated lymphoid tissues (MALTs) such as the Peyer’s patches in the gut. The architecture of these tissues is similar throughout the body and is structured to provide a crossroads for the interaction of rare clonal precursors of recirculating T and B cells with their cognate antigens—whether delivered by dendritic cells in the case of T cells, or as free antigens in the case of B cells. In view of the rarity of naive T cells that recognize a specific peptide:MHC complex—roughly 50–500 cells in the entire immune repertoire of approximately 100 million T cells in the mouse—and the large area over which an infectious agent can invade, the antigens derived from the pathogen, or in some instances the pathogen itself, must be brought from sites of entry to secondary lymphoid organs to facilitate their recognition by lymphocytes. In this part of the chapter we shall first consider the development and structure of secondary lymphoid organs that enable these interactions. We shall then discuss how naive T cells are directed to exit the blood and enter the lymphoid organs. This will be followed by considering how dendritic cells pick up antigen and travel to local lymphoid organs, where they can both present antigen to T cells and activate them. 9-1 T and B lymphocytes are found in distinct locations in secondary lymphoid tissues. The various secondary lymphoid organs are organized roughly along the same lines (see Chapter 1), with distinct areas in which B cells and T cells are concentrated—the B-cell and T-cell zones. They also contain macro phages, dendritic cells, and nonleukocyte stromal cells. In the case of the spleen, which is specialized for the capture of antigens that enter the bloodstream, the lymphoid tissue component is called the white pulp (Fig. 9.1). Fig. 9.1 Secondary lymphoid tissues serve as anatomical crossroads for interactions of antigens and lymphocytes. Secondary lymphoid tissues are specialized to serve as sites that facilitate interactions between lymphocytes and antigens. In lymph nodes (upper panel), antigen (denoted by red dots) is delivered in lymph either free or as cargo of dendritic cells that have taken up the antigen in the tissues drained by the lymph node. The antigen is conducted via afferent lymphatics to the subcapsular sinus, from which it is delivered to T-cell zones, where T cells can recognize it on the surface of dendritic cells; or, in the case of B cells, it is detected as a free antigen at the border of the T-cell zone and B-cell follicles. T and B cells enter the lymph node via high endothelial venules (HEVs) in T-cell zones, and then diverge into T-cell and B-cell zones. In the spleen (middle panel), antigen is delivered via arterioles that branch from the central arteriole to the marginal sinus, which is the boundary between the white pulp and red pulp, with which the marginal sinus communicates. In the marginal sinus, antigen can be taken up by marginal zone B cells, macrophages, or dendritic cells, which can transport antigen into either T-cell zones (periarteriolar lymphoid sheath, or PALS) or B-cell follicles. T and B cells enter the spleen via the same route as antigen, and leave the marginal sinus to travel to either the PALS or the B-cell follicles. In the intestines (lower panel), antigens are transported from the lumen via the microfold or M cells—specialized epithelium that overlays Peyer’s patches—to dendritic cells that reside in the subepithelial dome. Antigen-loaded dendritic cells are then surveyed by T cells in T-cell zones, and if the antigens they bear are not recognized by T cells locally, the dendritic cells can migrate to mesenteric lymph nodes to be further surveyed. As for lymph nodes, T and B cells enter the Peyer’s patches via HEVs in the T-cell zones. ERRNVPHGLFRVRUJ Lymph node dendritic cell loaded with antigen B-cell follicle subcapsular sinus HEV B-cell zone T-cell zone medulla blood vessel B cell T cell Spleen red pulp marginal sinus B-cell zone central arteriole B-cell follicle T-cell zone (PALS) white pulp Peyer’s patch dendritic cell bacteria follicle-associated epithelium M cell B-cell zone T-cell zone blood vessel afferent lymphatics to mesenteric lymph node B cell T cell Immunobiology | chapter 9 | 09_100 Murphy et al | Ninth edition © Garland Science design by blink studio limited 347 348 Chapter 9: T-cell-Mediated Immunity Each area of white pulp is demarcated from the red pulp by a marginal sinus, a vascular network that is formed from branches of the central arteriole. Circulating T and B cells are initially delivered to the marginal sinus, which is a highly organized region of cells that is specialized for the capture of bloodborne antigens or intact microbes, such as viruses and bacteria. It is rich in macrophages and contains a unique population of B cells, the marginal zone B cells, which do not recirculate. Pathogens reaching the bloodstream are efficiently trapped in the marginal zone by macrophages, and it could be that marginal zone B cells are adapted to provide the first responses to such pathogens. From the marginal sinus, T and B cells migrate centrally toward the central arteriole, where they bifurcate into T-cell zones that are clustered around the central arteriole—the so-called periarteriolar lymphoid sheath (PALS)— and B-cell zones, or follicles, that are located more peripherally. Some follicles may contain germinal centers, in which B cells involved in an adaptive immune response are proliferating and undergoing somatic hypermutation (see Section 1-16). The antigen-driven production of germinal centers will be described in detail when we consider B-cell responses in Chapter 10. Other types of cells are found within the B-cell and T-cell areas. The B-cell zone contains a network of follicular dendritic cells (FDCs), which are concentrated mainly in the area of the follicle most distant from the central arteriole. FDCs have long processes that are in contact with B cells. FDCs are a distinct type of cell from the dendritic cells we encountered previously (see Section 1-3), in that they are not leukocytes and are not derived from bone marrow precursors; in addition, they are not phagocytic and do not express MHC class II proteins. FDCs are specialized for the capture of antigen in the form of immune complexes—complexes of antigen, antibody, and complement. The immune complexes are not internalized but remain intact on the surface of the FDC for prolonged periods of time, where the antigen can be recognized by B cells. FDCs are also important in the development of B-cell follicles. T-cell zones contain a network of bone marrow-derived dendritic cells, sometimes known as interdigitating dendritic cells from the way in which their processes interweave among T cells. There are two major subtypes of these dendritic cells, distinguished by characteristic cell-surface proteins: one expresses the α chain of CD8, whereas the other is CD8-negative but expresses CD11b:CD18, an integrin that is also expressed by macrophages. As in the spleen, the T cells and B cells in lymph nodes are organized into discrete T-cell and B-cell areas (see Fig. 9.1). B-cell follicles have a similar structure and composition to those in the spleen and are located just under the outer capsule of the lymph node. T-cell zones surround the follicles in the paracortical areas. Unlike the spleen, lymph nodes have connections to both the blood system and the lymphatic system. Lymph conducted to lymph nodes by afferent lymphatic vessels enters into the subcapsular space, which is also known as the marginal sinus, and brings in antigen and antigen-bearing dendritic cells from the tissues. T and B cells enter the lymph node via specialized blood vessels called high endothelial venules (HEVs) that are found in T-cell zones, as will be discussed further in Section 9-3. The mucosa-associated lymphoid tissues (MALTs) are associated with the body’s epithelial surfaces, which provide physical barriers against infection. Peyer’s patches are part of the MALT and are lymph node-like structures interspersed at intervals just beneath the gut epithelium. They have B-cell follicles and T-cell zones (see Fig. 9.1), and the epithelium overlying them contains specialized M cells that are adapted to channel antigens and pathogens directly from the gut lumen to the underlying lymphoid tissue (see Section 1-16 and Chapter 12). Peyer’s patches and similar tissue present in the tonsils provide specialized sites where B cells can become committed to the synthesis of IgA. The mucosal immune system is discussed in more detail in Chapter 12. ERRNVPHGLFRVRUJ Development and function of secondary lymphoid organs—sites for the initiation of adaptive immune responses. 9-2 The development of secondary lymphoid tissues is controlled by lymphoid tissue inducer cells and proteins of the tumor necrosis factor family. Before discussing how T cells and B cells become partitioned into their respective zones in secondary lymphoid organs, we shall briefly look at how these organs develop in the first place. Lymphatic vessels are formed during embryonic development from endothelial cells that originate in blood vessels. Some endothelial cells in the early venous system begin to express the homeobox transcription factor Prox1. These cells bud from the vein, migrate away, and reassociate to form a parallel network of lymphatic vessels. Mice lacking Prox1 have normal arteries and veins, but fail to form a lymphatic system, showing this factor to be critical in establishing the identity of lymphatic endothelium. As the lymphatic vessels form, hematopoietic cells called lymphoid tissue inducer (LTi) cells arise in the fetal liver and are carried in the bloodstream to sites of prospective lymph nodes and Peyer’s patches. LTi cells initiate the formation of lymph nodes and Peyer’s patches by interacting with stromal cells and inducing the production of cytokines and chemokines, which recruit other lymphoid cells to these sites. Members of the tumor necrosis factor (TNF)/ TNF receptor (TNFR) family of cytokines turn out to be critically involved in the interactions between LTi cells and stromal cells. The role of this family of cytokines in the formation of secondary lymphoid organs has been demonstrated in a series of studies involving knockout mice in which either the TNF-family ligand or its receptor was inactivated (Fig. 9.2). These knockout mice have complicated phenotypes, which is partly due to the fact that individual TNF-family proteins can bind to multiple receptors and, conversely, many receptors can bind more than one ligand. In addition, it seems clear that there is some overlapping function or cooperation between TNFfamily proteins. Nonetheless, some general conclusions can be drawn. Lymph-node development depends on the expression of TNF-family proteins known as the lymphotoxins (LTs), and different types of lymph nodes depend on signals from different LTs. LT-α3, a soluble homotrimer of the LT-α chain, supports the development of cervical and mesenteric lymph nodes, and possibly lumbar and sacral lymph nodes. All these lymph nodes drain mucosal sites. LT-α3 probably exerts its effects by binding to TNFR-I. The membrane-bound heterotrimer consisting of two molecules of LT-α and one molecule of the distinct transmembrane protein LT-β (that is, LT-α2:β1), often known as LT-β, binds only to the LT-β receptor and supports the development of all the other lymph nodes. Peyer’s patches also do not form in the absence of LT-β. The effects of the LT knockouts are not reversible in adult animals; there are certain critical developmental periods during which the absence or inhibition of these LT-family proteins will permanently prevent the development of lymph nodes and Peyer’s patches. LTi cells express LT-β, which engages the LT-β receptors on stromal cells in the prospective lymphoid site, activating the non-canonical NFκB pathway Fig. 9.2 The role of TNF family members in the development of peripheral lymphoid organs. The role of TNF family members in the development of peripheral lymphoid organs has been deduced mainly from the study of knockout mice deficient in one or more TNF-family ligands or receptors. Some receptors bind more than one ligand, and some ligands bind more than one receptor, complicating elucidation of the effects of their deletion. (Note that receptors are named for the first ligand known to bind them.) The defects are organized here with respect to the two main receptors, TNFR-I and the LT-β receptor, and their ligands, TNF-α and the lymphotoxins (LTs). Note that in some cases, the losses of individual ligands out of several that bind the same receptor lead to different respective phenotypes, as indicated in the figure. This is due to the ability of the different ligands to bind different sets of receptors. The LT-α protein chain contributes to two distinct ligands, the trimer LT-α3 and the heterodimer LT-α2:β1, each of which acts through a distinct receptor. In general, signaling through the LT-β receptor is required for lymph-node and follicular dendritic cell (FDC) development and for normal splenic architecture, whereas signaling through TNFR-I is also required for FDCs and normal splenic architecture but not for lymph-node development. Effects seen in knockout (KO) mice Receptor TNFR-I LT-β receptor Ligands Spleen Peripheral lymph node Mesenteric lymph node Peyer's patch Follicular dendritic cells TNF-α LT-α3 Distorted architecture Present in TNF-α KO Absent in LT-α KO owing to lack of LT-β signals Present Reduced Absent TNF-α LT-α2:β1 Distorted architecture No marginal zones Absent Present in LT-β KO Absent in LT-β receptor KO Absent Absent Immunobiology | chapter 9 | 08_037 Murphy et al | Ninth edition © Garland Science design by blink studio limited ERRNVPHGLFRVRUJ 349 350 Chapter 9: T-cell-Mediated Immunity (see Section 7-23). This induces the stromal cells to express adhesion molecules and chemokines such as CXCL13 (B-lymphocyte chemokine, BLC), which in turn recruits more LTi cells, which have receptors for these molecules, eventually generating large clusters of cells that will become lymph nodes or Peyer’s patches. The chemokines also attract cells such as lymphocytes and other hematopoietic-lineage cells with appropriate receptors to populate the forming lymphoid organ. The principles, and even some of the molecules, underlying the development of secondary lymphoid organs in the fetus are very similar to those that maintain the organization of lymphoid organs in the adult, as we shall see in the next section. Fig. 9.3 The development of secondary lymphoid organs is orchestrated by chemokines. The cellular organization of lymphoid organs is initiated by stromal cells and vascular endothelial cells, which secrete the chemokine CCL21 (first panel). Dendritic cells with CCR7—a receptor for CCL21—are attracted to the site of the developing lymph node by CCL21 (second panel); it is not known whether at the earliest stages of lymph-node development immature dendritic cells enter from the bloodstream or via the lymphatics, as they do later in life. Once in the lymph node, the dendritic cells express the chemokine CCL19, which is also bound by CCR7. Together, the chemokines secreted by stromal cells and dendritic cells attract T cells to the developing lymph node (third panel). The same combination of chemokines also attracts B cells into the developing lymph node (fourth panel). The B cells are able to either induce the differentiation of the nonleukocyte FDCs (which are a lineage distinct from the bone marrow-derived dendritic cells) or direct their recruitment into the lymph node. Once present, the FDCs secrete CXCL13, a chemokine that is a chemoattractant for B cells. The production of CXCL13 drives the organization of B cells into discrete B-cell areas (follicles) around the FDCs and contributes to the further recruitment of B cells from the circulation into the lymph node (fifth panel). Stromal cells and high endothelial venules (HEVs) secrete the chemokine CCL21 Although the spleen will develop in mice deficient in any of the known TNF or TNFR family members, its architecture will be abnormal in many of these mutants (see Fig. 9.2). LT (most probably the membrane-bound LT-β) is required for the normal segregation of T-cell and B-cell zones in the spleen. TNF-α, binding to TNFR-I, also contributes to the organization of the white pulp: when TNF-α signals are disrupted, B cells surround T-cell zones in a ring rather than forming discrete follicles, and the marginal zones are not well defined. Perhaps the most important role of TNF-α and TNFR-I in lymphoid organ development is in the development of FDCs, as these cells are lacking in mice with knockouts of either TNF-α or TNFR-I (see Fig. 9.2). The knockout mice do have lymph nodes and Peyer’s patches, because they express LTs, but these structures lack FDCs. LT-β is also required for FDC development: mice that cannot form LT-β or signal through its receptor lack normal FDCs in the spleen and any residual lymph nodes. Unlike the disruption of lymph-node development, the disorganized lymphoid architecture in the spleen is reversible if the missing TNF-family member is restored. B cells are the likely source of the LT-β, because normal B cells can restore FDCs and follicles when transferred to RAG-deficient recipients (which lack lymphocytes). 9-3 T and B cells are partitioned into distinct regions of secondary lymphoid tissues by the actions of chemokines. Circulating T and B cells seed secondary lymphoid tissues from the blood by a common route, but are then directed into their respective compartments under the control of distinct chemokines that are produced by both stromal cells and bone marrow-derived cells resident in the T- and B-cell zones (Fig. 9.3). The localization of T cells into T-cell zones involves two chemokines, CCL19 (MIP-3β) and CCL21 (secondary lymphoid chemokine, SLC). Both of these bind the receptor CCR7, which is expressed by T cells; mice that lack Dendritic cells express a receptor for CCL21 and migrate into the developing lymph node Dendritic cells secrete CCL19, which attracts T cells to the developing lymph node B cells are initially attracted into the developing lymph node by the same chemokines B cells induce the differentiation of follicular dendritic cells, which in turn secrete the chemokine CXCL13 to attract more B cells CCL19 HEV CCL21 CCL21 CXCL13 stromal cell B-cell follicle dendritic cell Immunobiology | chapter 9 | 08_038 Murphy et al | Ninth edition © Garland Science design by blink studio limited ERRNVPHGLFRVRUJ Development and function of secondary lymphoid organs—sites for the initiation of adaptive immune responses. CCR7 do not form normal T-cell zones and have impaired primary immune responses. CCL21 is produced by stromal cells of T-cell zones in secondary lymphoid tissues, and is displayed on endothelial cells of high endothelial venules (HEVs). Another source of CCL21 is interdigitating dendritic cells, which also produce CCL19 and are prominent in T-cell zones. Indeed, dendritic cells themselves express CCR7 and will localize to secondary lymphoid tissues even in RAG-deficient mice, which lack lymphocytes and therefore defined T-cell zones. Thus, during normal lymph-node development, the T-cell zone might be organized first through the attraction of dendritic cells and T cells by CCL21 produced by stromal cells. This organization would then be reinforced by CCL21 and CCL19 secreted by resident dendritic cells, which in turn attract more T cells and migratory dendritic cells. Like T cells, circulating B cells express CCR7, which initially directs them into the lymph node across HEVs. Because they also constitutively express the chemokine receptor CXCR5, they are then attracted to the follicles by the ligand for this receptor, CXCL13. The most likely source of CXCL13 is the FDC, possibly along with other follicular stromal cells. This is reminiscent of the expression of CXCL13 by stromal cells during the formation of the lymph node (Section 9-2). B cells are, in turn, the source of the LT that is required for the development of FDCs, which is reminiscent of LTi cells expressing the LT required to activate stromal cells. The reciprocal dependence of B cells and FDCs, and LTis and stromal cells, illustrates the complex web of interactions that organizes secondary lymphoid tissues. A subset of CD4 T cells called T follicular helper, or TFH, cells can also express CXCR5 following their activation by antigen, allowing them to enter B-cell follicles to participate in the formation of germinal centers (see Chapter 10). 9-4 Naive T cells migrate through secondary lymphoid tissues, sampling peptide:MHC complexes on dendritic cells. Naive T cells perpetually circulate from the bloodstream into lymph nodes, spleen, and mucosa-associated lymphoid tissues and back to the blood (see Fig. 1.21). This allows them to contact thousands of dendritic cells every day and sample the peptide:MHC complexes on the surfaces of these cells. Because of their high rates of recirculation and their concentration in T-cell zones where incoming dendritic cells dwell, each T cell has a high probability of encountering antigens derived from any pathogen that has set up an infection anywhere in the body (Fig. 9.4). Within hours of their arrival, naive T cells that do not encounter their specific antigen exit from the lymphoid tissue and reenter the bloodstream, where they continue to recirculate—via the efferent lymphatics in lymph nodes or MALTs, or directly back to the blood in the spleen, which has no connection with the lymphatic system. T cells enter lymph node cortex from the blood via high endothelial venules (HEVs) lymph cortical sinus follicle HEV dendritic cell paracortex medullary sinus efferent lymphatic T cell artery vein T cells not activated by antigen presented by dendritic cells exit from the lymph node via the cortical sinuses follicle HEV cortical sinus T cells activated by antigen presented by dendritic cells start to proliferate and lose the ability to exit from the lymph node When a naive T cell recognizes its specific antigen on the surface of an activated dendritic cell, however, it ceases to migrate. It remains in the T-cell zone, Fig. 9.4 Naive T cells encounter antigen during their recirculation through peripheral lymphoid organs. Naive T cells recirculate through peripheral lymphoid organs, such as a lymph node (shown here), entering from the arterial blood via the specialized vascular endothelium of high endothelial venules (HEVs). Entry into the lymph node is regulated by chemokines (not shown) that direct the T cells’ migration through the HEV wall and into the paracortical areas, where the T cells encounter mature dendritic cells (top panel). Those T cells shown in green do not encounter their specific antigen; they receive a survival signal through their interaction with self peptide:self MHC complexes and IL-7, and leave the lymph node through the lymphatics to return to the circulation (second panel). T cells shown in blue encounter their specific antigen on the surface of mature dendritic cells; they lose their ability to exit from the node and become activated to proliferate and to differentiate into effector T cells (third panel). After several days, these antigen-specific effector T cells regain the expression of receptors needed to exit from the node, leave via the efferent lymphatics, and enter the circulation in greatly increased numbers (bottom panel). ERRNVPHGLFRVRUJ Activated T cells differentiate to effector cells and exit from the lymph node Immunobiology | chapter 9 | 09_002 Murphy et al | Ninth edition © Garland Science design by blink studio limited 351 352 Chapter 9: T-cell-Mediated Immunity where it proliferates for several days, undergoing clonal expansion and differentiation to give rise to effector T cells and memory cells of identical antigen specificity. At the end of this period, most effector T cells exit the lymphoid organ and reenter the bloodstream, through which they migrate to the sites of infection (see Chapter 11). Some effector T cells that are fated to interact with B cells migrate instead to B-cell zones, where they participate in the germinal center response (see Chapter 10). Antigen-specific T cells are detained transiently in the lymph node, where they become activated Number of antigenspecific cells in efferent lymph Trapping 0 2 Activation 4 Emigration of effector T cells 6 8 Time after viral infection (days) Immunobiology | chapterand 9 | 09_003 Fig. 9.5 Trapping activation of Murphy et al | Ninth edition antigen-specific naive T cells in © Garland Science design by blink studio limited lymphoid tissue. Naive T cells entering the lymph node from the blood encounter antigen-presenting dendritic cells in T-cell zones. T cells that recognize their specific antigen bind stably to the dendritic cells and are activated through their T-cell receptors, resulting in their retention within the lymph node as they develop into effector T cells. By 5 days after the arrival of antigen, activated effector T cells are leaving the lymph node in large numbers via the efferent lymphatics. Lymphocyte recirculation and recognition are so effective that all the naive T cells in the peripheral circulation specific for a particular antigen can be trapped by that antigen in one node within 2 days. The efficiency with which T cells screen antigen-presenting cells in lymph nodes is very high, as can be seen by the rapid trapping of antigen-specific T cells in a single lymph node containing antigen; within 48 hours, all antigenspecific T cells in the body can be trapped in the lymph node draining a site of antigen injection (Fig. 9.5). Such efficiency is crucial for the initiation of an adaptive immune response, as only one naive T cell in 105–106 is likely to be specific for a particular antigen, and adaptive immunity depends on the activation and expansion of these rare cells. 9-5 Lymphocyte entry into lymphoid tissues depends on chemokines and adhesion molecules. Migration of naive T cells into secondary lymphoid tissues depends on their binding to high endothelial venules (HEVs) through cell–cell interactions that are not antigen-specific but are governed by cell-adhesion molecules. The main classes of adhesion molecules involved in lymphocyte interactions are the selectins, the integrins, members of the immunoglobulin superfamily, and some mucin-like molecules (see Fig. 3.30). Entry of lymphocytes into lymph nodes occurs in distinct stages that include initial rolling of lymphocytes along the endothelial surface, activation of integrins, firm adhesion, and trans migration or diapedesis across the endothelial layer into the paracortical areas, the T-cell zones (Fig. 9.6). These stages are regulated by a coordinated interplay of adhesion molecules and chemokines that resembles the recruitment of leukocytes to sites of inflammation (see Chapter 3). Adhesion mole cules have fairly broad roles in immune responses, being involved not only in lymphocyte migration but also in interactions between naive T cells and antigen-presenting cells (see Section 9-14). The selectins (Fig. 9.7) are important for specifically guiding leukocytes to particular tissues, a phenomenon known as leukocyte homing. L-selectin (CD62L) is expressed on leukocytes, whereas P-selectin (CD62P) and E-selectin (CD62E) are expressed on vascular endothelium (see Section 3-18). L-selectin on naive T cells guides their exit from the blood into secondary lymphoid tissues by initiating a light attachment to the wall of the HEV that results in the T cells’ rolling along the endothelial surface (see Fig. 9.6). P-selectin and Fig. 9.6 Lymphocyte entry into a lymph node from the blood occurs in distinct stages involving the activity of adhesion molecules, chemokines, and chemokine receptors. Naive T cells are induced to roll along the surface of a high endothelial venule (HEV) by the interactions of selectins expressed by the T cells with vascular addressins on the endothelial cell membranes. Chemokines present at the HEV surface activate receptors on the T cell, and chemokine receptor signaling leads to an increase in the affinity of integrins on the T cell for the adhesion molecules expressed on the HEV. This induces strong adhesion. After adhesion, the T cells follow gradients of chemokines to pass through the HEV wall into the paracortical region of the lymph node. Rolling Activation Adhesion Diapedesis Selectins Chemokines Integrins Chemokines L-selectin CCL21 LFA-1 CCL21, CXL12 Immunobiology | chapter 9 | 09_004 Murphy et al | Ninth edition ERRNVPHGLFRVRUJ © Garland Science design by blink studio limited Development and function of secondary lymphoid organs—sites for the initiation of adaptive immune responses. Binding of selectins to vascular addressins naive T cell naive T cell X x sulfated sialyl-Lewis sialyl-Lewis L-selectin MAdCAM-1 CD34 GlyCAM-1 high endothelial venule mucosal endothelium Immunobiology | chapter 9 | 09_005 Murphy et al | Ninth edition E-selectin are expressed on the vascular endothelium at sites of infection, and serve to recruit effector cells into the infected tissue. Selectins are cellsurface molecules with a common core structure and are distinguished from each other by the presence of different lectin-like domains in their extracellular portion. The lectin domains bind to particular sugar groups, and each selectin binds to a cell-surface carbohydrate. L-selectin binds to the carbohydrate moiety—sulfated sialyl-LewisX—of mucin-like molecules called vascular addressins, which are expressed on the surface of vascular endothelial cells. Two of these addressins, CD34 and GlyCAM-1 (see Fig. 9.7), are expressed on high endothelial venules in lymph nodes. A third, MAdCAM-1, is expressed on endothelium in mucosae, and guides lymphocyte entry into mucosal lymphoid tissue such as the Peyer’s patches in the gut. © Garland Science design by blink studio limited The interaction between L-selectin and the vascular addressins is responsible for the specific homing of naive T cells to lymphoid organs. On its own, however, it does not enable the cell to cross the endothelial barrier into the lymphoid tissue. This requires the concerted action of chemokines and integrins. 9-6 Activation of integrins by chemokines is responsible for the entry of naive T cells into lymph nodes. Naive T cells rolling on the endothelium of HEVs via selectins require two additional types of cell-adhesion molecules to enter secondary lymphoid organs— integrins, and members of the immunoglobulin superfamily. Integrins bind tightly to their ligands after receiving signals that induce a change in their conformation. Signaling by chemokines activates integrins on leukocytes to bind tightly to the vascular wall in preparation for the migration of the leukocytes into sites of inflammation (see Section 3-18). Similarly, chemokines present at the luminal surface of the HEV activate integrins expressed on naive T cells during migration into lymphoid organs (see Fig. 9.6). An integrin molecule consists of a large α chain that pairs noncovalently with a smaller β chain. There are several integrin subfamilies, broadly defined by their common β chains. We will be concerned here chiefly with the leukocyte integrins, which have a common β2 chain paired with distinct α chains (Fig. 9.8). All T cells express the integrin αL:β2 (CD11a:CD18), better known as leukocyte functional antigen-1 (LFA-1). It enables migration of both naive and effector T cells out of the blood. This integrin is also present on macrophages and neutrophils, and is involved in their recruitment to sites of infection (see Section 3-18). LFA-1 is also important in the adhesion of both naive and effector T cells to their target cells. Nevertheless, T-cell responses can be normal in individuals genetically lacking the β2 integrin chain and hence all β2 integrins, ERRNVPHGLFRVRUJ Fig. 9.7 L-selectin binds to mucinlike vascular addressins. L-selectin is expressed on naive T cells and recognizes carbohydrate motifs. Its binding to sulfated sialyl-LewisX moieties on the vascular addressins CD34 and GlyCAM-1 on HEVs binds the lymphocyte weakly to the endothelium. The relative importance of CD34 and GlyCAM-1 in this interaction is unclear. CD34 has a transmembrane anchor and is expressed in appropriately glycosylated form only on HEV cells, although it is found in other forms on other endothelial cells. GlyCAM-1 is expressed on HEVs but has no transmembrane region and may be secreted into the HEVs. The addressin MAdCAM-1 is expressed on mucosal endothelium and guides lymphocytes to mucosal lymphoid tissue. The configuration shown represents mouse MAdCAM-1, which contains an IgA-like domain closest to the cell membrane; human MAdCAM-1 has an elongated mucin-like domain and lacks the IgA-like domain. 353 354 Chapter 9: T-cell-Mediated Immunity Fig. 9.8 Integrins are important in T-lymphocyte adhesion. Integrins are heterodimeric proteins containing a β chain, which defines the class of integrin, and an α chain, which defines the different integrins within a class. The α chain is larger than the β chain and contains binding sites for divalent cations that may be important in signaling. LFA-1 (integrin αL:β2) is expressed on all leukocytes. It binds ICAMs and is important in cell migration and in the interactions of T cells with antigenpresenting cells (APCs) or target cells; it is expressed at higher levels on effector T cells than on naive T cells. Lymphocyte Peyer’s patch adhesion molecule (LPAM‑1, or integrin α4:β7) is expressed by a subset of naive T cells and contributes to lymphocyte entry into mucosal lymphoid tissues by supporting adhesive interactions with vascular addressin MAdCAM-1. VLA-4 (integrin α4:β1) is expressed strongly after T-cell activation. It binds to VCAM-1 on activated endothelium and is important for recruiting effector T cells into sites of infection. Binding of integrins to adhesion molecules all T cells integrin adhesion molecule activated effector T cells subset of naive cells LFA-1 VLA-4 LPAM-1 β7 αL β2 ICAM-1 β1 α4 MAdCAM-1 HEV or APC α4 VCAM-1 mucosal endothelium activated endothelium Immunobiology | chapter 9 | 09_006 Murphy et al | Ninth edition This is including LFA-1. probably because T cells also express other adhesion molecules, including the immunoglobulin superfamily member CD2 and β1 integrins, which may compensate for the absence of LFA-1. Expression of the β1 integrins increases significantly at a late stage in T-cell activation, and they are thus often called VLAs, for very late activation antigens; they are important in directing effector T cells to inflamed target tissues. © Garland Science design by blink studio limited At least five members of the immunoglobulin superfamily are especially important in T-cell activation (Fig. 9.9). Three very similar intercellular adhesion molecules (ICAMs)—ICAM-1, ICAM-2, and ICAM-3—all bind to the T-cell integrin LFA-1. ICAM-1 and ICAM-2 are expressed on endothelium as well as on antigenpresenting cells, and binding to these molecules enables lymphocytes to migrate through blood vessel walls. ICAM-3 is expressed only on naive T cells and is thought to have an important role in the adhesion of T cells to antigen-presenting cells by binding to LFA-1 expressed on dendritic cells. The two remaining immunoglobulin superfamily adhesion molecules, CD58 (formerly known as LFA-3) on the antigen-presenting cell and CD2 on the T cell, bind to each other; this interaction synergizes with that of ICAM-1 or ICAM-2 with LFA-1. As discussed above in the context of lymphoid tissue development (see Section 9-3), naive T cells are specifically attracted into the T-cell zones of secondary lymphoid tissues by chemokines. The chemokines bind to proteoglycans in the extracellular matrix and high endothelial venule wall, forming a chemical gradient, and are recognized by receptors on the naive T cell. The extravasation of naive T cells is prompted by the chemokine CCL21, which is expressed by vascular high endothelial cells and the stromal cells of lymphoid tissues, as well as by dendritic cells that reside in T-cell zones. It binds to the chemo kine receptor CCR7 on naive T cells, stimulating activation of the intracellular receptor-associated G-protein subunit Gαi. The resulting intracellular signaling rapidly increases the affinity of integrin binding (see Section 3-18). Immunoglobulin superfamily Fig. 9.9 Immunoglobulin superfamily adhesion molecules involved in leukocyte interactions. Adhesion molecules of the immunoglobulin superfamily bind to adhesion molecules of various types, including integrins (LFA-1 and VLA-4) and other immunoglobulin superfamily members [the CD2–CD58 (LFA-3) interaction]. These interactions have a role in lymphocyte migration, homing, and cell–cell interactions; see Fig. 3.24 for the other molecules listed here. ICAM1/3, VCAM1 CD58 CD2 Name Tissue distribution Ligand CD2 (LFA-2) T cells CD58 (LFA-3) ICAM-1 (CD54) Activated vessels, lymphocytes, dendritic cells LFA-1, Mac-1 ICAM-2 (CD102) Resting vessels LFA-1 ICAM-3 (CD50) Naive T cells LFA-1 LFA-3 (CD58) Lymphocytes, antigen-presenting cells CD2 VCAM-1 (CD106) Activated endothelium VLA-4 Immunobiology | chapter 9 | 09_007 Murphy et al | Ninth edition © Garland Science design by blink studio limited ERRNVPHGLFRVRUJ Development and function of secondary lymphoid organs—sites for the initiation of adaptive immune responses. Circulating lymphocyte enters the high endothelial venule in the lymph node Binding of L-selectin to GlyCAM-1 and CD34 allows rolling interaction LFA-1 is activated by CCR7 signaling in response to CCL21 bound to endothelial surface Activated LFA-1 binds tightly to ICAM-1 Lymphocyte migrates into the lymph node by diapedesis CCR7 LFA-1 L-selectin GlyCAM-1 ICAM-1 CD34 CCL21 basement membrane lymph node Fig. 9.10 Lymphocytes in the blood enter lymphoid tissue by Immunobiology | chapter 9 | 09_008 Murphy et al | the Ninthwalls edition of high endothelial venules. The first step is crossing Garland Scienceof design by blink studio © the binding L-selectin onlimited the lymphocyte to sulfated carbohydrates (sulfated sialyl-LewisX) of GlyCAM-1 and CD34 on the HEV. Local chemokines such as CCL21 bound to a proteoglycan matrix on the HEV surface stimulate chemokine receptors on the T cell, leading to the activation of LFA-1. This causes the T cell to bind tightly to ICAM‑1 on the endothelial cell, allowing migration across the endothelium. As in the case of neutrophil migration (see Fig. 3.31), matrix metalloproteinases on the lymphocyte surface (not shown) enable the lymphocyte to penetrate the basement membrane. The entry of a naive T cell into a lymph node is shown in detail in Fig. 9.10. Initial rolling of the T cell along the surface of HEVs is mediated by L-selectin. Recognition of CCL21 on the endothelial surface of the HEV by CCR7 on the T cell causes LFA-1 to become activated, increasing its affinity for ICAM-2 and ICAM-1. ICAM-2 is expressed constitutively on all endothelial cells, whereas in the absence of inflammation, ICAM-1 is expressed only on the high endothelial cells of secondary lymphoid tissues. The organization of LFA-1 molecules in the T-cell membrane is also altered by chemokine stimulation, such that they become concentrated in areas of cell–cell contact. This produces stronger binding, which arrests the T cell on the endothelial surface and thus enables it to enter the lymphoid tissue. Once naive T cells have arrived in the T-cell zone via high endothelial venules, CCR7 directs their retention in this location, as they are attracted to dendritic cells that produce CCL21 and CCL19 in the T-cell zone. The naive T cells scan the surfaces of dendritic cells for specific peptide:MHC complexes, and if they find their antigen and bind to it, they are trapped in the lymph node. If they are not activated by antigen, naive T cells soon leave the lymph node (see Fig. 9.4). 9-7 The exit of T cells from lymph nodes is controlled by a chemotactic lipid. T cells exit from a lymph node via the cortical sinuses, which lead into the medullary sinus and then the efferent lymphatic vessel. The egress of T cells from secondary lymphoid organs involves the lipid molecule sphingosine 1-phosphate (S1P) (Fig. 9.11). This lipid has chemotactic activity and signaling properties similar to those of chemokines, in that the receptors for S1P are G-protein-coupled receptors. A concentration gradient of S1P between the lymphoid tissues and lymph or blood acts to draw unactivated naive T cells expressing an S1P receptor away from the lymphoid tissues and back into circulation. ERRNVPHGLFRVRUJ 355 356 Chapter 9: T-cell-Mediated Immunity Fig. 9.11 The egress of lymphocytes from lymphoid tissue is mediated by a sphingosine 1-phosphate (S1P) gradient. The level of sphingosine 1-phosphate (S1P) within lymphoid tissue is low compared with efferent lymph, thereby forming an S1P gradient (indicated by shading). The S1P receptor 1 (S1PR1) expressed on naive T cells is responsive to the S1P gradient. In the absence of antigen recognition, S1PR1 signaling promotes T-cell egress from the T-cell zones into the efferent lymphatic vessel. T cells activated by an antigen-expressing dendritic cell upregulate CD69, which causes a decrease in S1PR1 expression and retention in the T-cell zone. Effector T cells eventually reexpress S1PR1 as CD69 expression decreases, and thereby egress from the lymph node. FTY720 inhibits T-cell egression by downmodulating expression of S1PR1 by ligand-induced internalization and by S1PR1-mediated closure of egress ports on the endothelium by enhancement of junctional contacts (not shown). lymph node naive T cell S1PR1 effector T cells dendritic cell ↑CD69 ↓S1PR1 FTY720 proliferation T-cell activation no T-cell activation CD69 chemoattraction/ retention override endothelial cell ↓CD69 ↑S1PR1 S1P efferent lymphatic Immunobiology | chapter 9 |by 09_103 T cells activated antigen Murphy et al | Ninth edition in lymphoid organs downregulate the surface expression of the S1P receptor, S1PR1, for several days. This loss of S1PR1 surface expression is caused by CD69, a surface protein whose expression is induced by T-cell receptor signaling and which acts to internalize S1PR1. During this period, T cells cannot respond to the S1P gradient and do not exit the lymphoid organ. After several days of proliferation, as T-cell activation wanes, CD69 expression decreases and S1PR1 reappears on the surface of effector T cells, allowing them to migrate out of the lymphoid tissue in response to the S1P gradient. © Garland Science design by blink studio limited The regulation of the exit of both naive and effector lymphocytes from secondary lymphoid organs by S1P is the basis for a new kind of potential immunosuppressive drug, FTY720 (fingolimod). FTY720 inhibits immune responses by preventing lymphocytes from returning to the circulation, thereby sequestering them in lymphoid tissues and causing rapid onset of lymphopenia (a lack of lymphocytes in the blood). In vivo, FTY720 becomes phosphorylated and mimics S1P as an agonist at S1P receptors. Phosphorylated FTY720 may inhibit lymphocyte exit by effects on endothelial cells that increase tight junction formation and close exit portals, or by chronic activation of S1P receptors, leading to inactivation and downregulation of the receptor. 9-8 T-cell responses are initiated in secondary lymphoid organs by activated dendritic cells. Secondary lymphoid organs were first shown to be important in the initiation of adaptive immune responses by ingenious experiments in which a flap of skin was isolated from the body wall so that it had blood circulation but no lymphatic drainage. Antigen placed in the flap did not elicit a T-cell response, showing that T cells do not become sensitized in the infected tissue itself. Rather, pathogens and their products must be transported to lymphoid tissues. Antigens introduced directly into the bloodstream are picked up by antigen-presenting cells in the spleen. Pathogens infecting other sites, such as a skin wound, are transported in lymph via lymphatic vessels and trapped in the lymph nodes nearest ERRNVPHGLFRVRUJ Development and function of secondary lymphoid organs—sites for the initiation of adaptive immune responses. to the site of infection (see Section 1-16). Pathogens infecting mucosal surfaces are transported directly across the mucosa into lymphoid tissues such as the tonsils or Peyer’s patches, as well as draining lymph nodes. In this chapter we will focus on T-cell activation by dendritic cells as it occurs in organs of the systemic immune system—lymph nodes and spleen. The activation of T cells by dendritic cells in the mucosal immune system follows the same principles, but differs in some details, described in Chapter 12, such as the route by which antigen is delivered and the subsequent circulation patterns of the effector cells. The delivery of antigen from a site of infection to lymphoid tissue is actively aided by the innate immune response. One effect of innate immunity is an inflammatory reaction that increases the rate of entry of blood plasma into infected tissues and thus increases the drainage of extracellular fluid into the lymph, taking with it free antigen that is carried to lymphoid tissues. Even more important for initiation of the adaptive response is the activation of tissue dendritic cells that have taken up particulate and soluble antigens at the site of infection (Fig. 9.12). Dendritic cells in peripheral tissues Dendritic cells in the lymphatic circulation Dendritic cells in lymphoid tissues T cell dendritic cell Immunobiology | chapter 9 | 09_009 Murphy et al | Ninth edition © Garland Science design by blink studio limited ERRNVPHGLFRVRUJ Fig. 9.12 Dendritic cells in different stages of activation and migration. The left panels show fluorescence micrographs of dendritic cells stained for MHC class II molecules in green and for a lysosomal protein in red. The right panels show scanning electron micrographs of single dendritic cells. Unactivated dendritic cells (top panels) have many long processes, or dendrites, from which the cells get their name. The cell bodies are difficult to distinguish in the left panel, but the cells contain many endocytic vesicles that stain both for MHC class II molecules and for the lysosomal protein; when these two colors overlap they give rise to a yellow fluorescence. Activated dendritic cells leave the tissues to migrate through the lymphatics to secondary lymphoid tissues. During this migration their morphology changes. The dendritic cells stop phagocytosing antigen, and staining for the lysosomal protein is beginning to be distinct from that for MHC class II molecules (center left panel). The dendritic cell now has many folds of membrane (center right panel), which gave these cells their original name of ‘veil’ cells. Finally, in the lymph nodes, dendritic cells express high levels of peptide:MHC complexes and co‑stimulatory molecules, and are very good at stimulating naive CD4 and naive CD8 T cells. At this stage, the activated dendritic cells do not phagocytose, and the red staining of the lysosomal protein is quite distinct from the green-stained MHC class II molecules displayed at high density on many dendritic processes (bottom left panel). The typical morphology of a mature dendritic cell is shown in the bottom right panel, as it interacts with a T cell. Fluorescent micrographs courtesy of I. Mellman, P. Pierre, and S. Turley. Scanning electron micrographs courtesy of K. Dittmar. 357 358 Chapter 9: T-cell-Mediated Immunity Dendritic cells (interdigitating reticular cells) bacterial antigen viral antigen virus infecting the dendritic cell Macrophages bacterium B cells microbial toxin Dendritic cells can be activated via their TLRs and other pathogen-recognition receptors (see Chapter 3), by tissue damage, or by cytokines produced during the inflammatory response. Activated dendritic cells migrate to the lymph node and express the co-stimulatory molecules that are required, in addition to antigen, for the activation of naive T cells. In the lymphoid tissues, these dendritic cells present antigen to naive T lymphocytes and prime antigen-specific T cells to divide and mature into effector cells that reenter the circulation. Macrophages, which are found in most tissues including lymphoid tissue, and B cells, which are located primarily in lymphoid tissue, can be similarly activated by pathogen-recognition receptors to express co-stimulatory molecules and act as antigen-presenting cells. The distribution of dendritic cells, macrophages, and B cells in a lymph node is shown schematically in Fig. 9.13. Only these three cell types express co-stimulatory molecules required to efficiently activate T cells, and they express these molecules only when activated in the context of infection. However, these cells activate T-cell responses in distinct ways. Dendritic cells take up, process, and present antigens from all types of sources, and are present mainly in the T-cell areas where they drive the initial clonal expansion and differentiation of naive T cells into effector T cells. By contrast, B cells and macrophages specialize in processing and presenting soluble antigens and antigens from intracellular pathogens, respectively; they interact mainly with effector CD4 T cells already primed by dendritic cells to recruit helper functions of those T cells. 9-9 Immunobiology | chapter 9 | 09_010 Fig. 9.13 Antigen-presenting cells are Murphy et al | Ninth distributed byedition type in specific areas of © Garland Science design by blink studio limited the lymph node. Dendritic cells are found throughout the cortex of the lymph node in the T-cell areas. Mature dendritic cells are by far the strongest activators of naive T cells, and can present antigens from many types of pathogens, such as bacteria or viruses as shown here. Macrophages are distributed throughout the lymph node but are concentrated mainly in the marginal sinus, where the afferent lymph collects before percolating through the lymphoid tissue, and also in the medullary cords, where the efferent lymph collects before passing via the efferent lymphatics into the blood. B cells are found mainly in the follicles and can contribute to neutralizing soluble antigens such as toxins. Dendritic cells process antigens from a wide array of pathogens. Dendritic cells primarily arise from myeloid progenitors within the bone marrow (see Fig. 1.3). They emerge from the bone marrow to migrate via the blood to tissues throughout the body, or directly to secondary lymphoid organs. There are two major classes of dendritic cells: conventional dendritic cells, and plasmacytoid dendritic cells (Fig. 9.14). The cell-surface markers and subset-specific transcription factors that distinguish these two classes, and the interferon-producing functions of plasmacytoid dendritic cells in the innate Conventional dendritic cell DC-SIGN Plasmacytoid dendritic cell MHC class II CCR7 MHC class II MHC class I ICAM-2 BDCA-2 CXCR3 B7.1 TLR-7 CD11c LFA-1 IFN-β CD58 CCL19 IFN-α ICAM-1 B7.2 TLR-9 Fig. 9.14 Conventional and plasmacytoid dendritic cells have different roles in the Immunobiology | chapter 9 | 09_011 Murphy et al response. | Ninth edition Mature conventional dendritic cells (left panel) are primarily concerned immune Garland Science design by blink studio limited © with the activation of naive T cells. There are several subsets of conventional dendritic cells, but these all process antigen efficiently, and when they are mature they express MHC proteins and co-stimulatory molecules for priming naive T cells. The cell-surface proteins expressed