Magnetic Resonance Imaging (MRI) PDF
Document Details
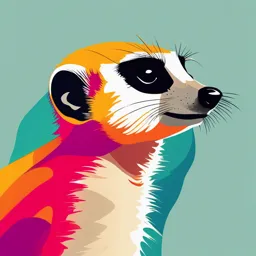
Uploaded by WillingPixie4700
Vinschool Grand Park
Tags
Summary
This document provides a detailed explanation of MRI, including its capabilities, principles and components, such as magnets, coils, and signal processing. It discusses the strengths of magnets and various aspects of image acquisition and processing.
Full Transcript
MRI Capabilities Chemical Differences: Displays variations in tissues as grey-scale intensities (e.g., detecting tumors). Blood Flow: Captures high-intensity vessel images in thin slices or 3D. Various Views: Provides axial, coronal, sagittal, oblique, or 3D renderings....
MRI Capabilities Chemical Differences: Displays variations in tissues as grey-scale intensities (e.g., detecting tumors). Blood Flow: Captures high-intensity vessel images in thin slices or 3D. Various Views: Provides axial, coronal, sagittal, oblique, or 3D renderings. Long Slices: Useful for sagittal views, especially for the spine. Principles of MRI Magnets and Electricity changing a magnetic field produces a current in a loop of wire (electromotive force, EMF). A current in a loop generates a magnetic field. Nuclear Spin Each nucleus has an intrinsic magnetic moment (μ=γS). Nuclei precess (rotate) in the magnetic field. MRI Process 1. Apply known gradients to B0 (the main magnetic field). 2. Detects EMF caused by precessing nuclei. 3. Use Discrete Fourier Transform (DFT) to reconstruct images. MRI Components Main Magnet: Provides the static magnetic field. Cryostats: Keeps the superconductor magnet cold. Shim Coils: Adjusts the static field to ensure uniformity. Gradient Coils: delivers changing magnetic fields. RF Coils: Delivers RF pulses to excite nuclei and records their precession frequencies. Patient Table: Supports the patient during the scan. Electronics: Processes NMR signals. Computer: Processes and reconstructs the images. Main Magnet - Magnet Strength Depending on the object size being studied using MR, the strength of the magnet can be very low or very high. Clinical Magnets: ○ Previously common: 1.5 T magnets. ○ Now widely used: 3 T magnets. The smaller the subject, the larger the magnet strength examples: ○ 7 T and 11.7 T for small animals. ○ 21.1 T or higher for chemistry NMR systems. World’s Strongest Magnet: ○ Located at the National High Magnetic Field Laboratory, Tallahassee, FL, USA. ○ Strength: 45 T, three stories tall. ○ Used for research in material science, physics, chemistry, and biology. Main Magnet - Magnet Types Superconductors in Clinical MRI: ○ Used in MRIs with magnetic fields of 1.5 T and above. ○ Operate at extremely cold temperatures (4 K = -269°C). Cooling System: ○ Surrounded by liquid helium in cryostats to maintain low temperatures. ○ Liquid helium can cool to milli Kelvin (mK). ○ Sometimes it is surrounded by a Dewar of liquid nitrogen (37 K = -196°C) to reduce helium boil-off. Operational Costs: ○ Magnets are costly to buy and costly to operate and maintain due to the need for liquid helium and nitrogen. Quenching: ○ In the absence of adequate liquid helium or liquid nitrogen, the magnet loses its magnetic field (called "quenched"). ○ Quenching can cause severe damage to the magnet's structure. Cryogenics Importance: ○ Maintaining very cold materials and ensuring they are in good condition is essential for MRI functionality. Magnet Information and Safety Magnetic Field Uniformity: ○ A uniform magnetic field is crucial for high-quality imaging. ○ Shimming: Adjusting currents in shim coils to achieve field uniformity. High Magnetic Fields can be hazardous to people and environment: ○ Can disrupt pacemakers (medical device implanted in the chest), affecting heart rhythm. ○ Magnetic fields can interfere with electronics. ○ objects can be attracted by magnetic fields. Safety Measures: ○ The 0.5 mT contour line (area with magnetic field influence) must be marked clearly and kept close to the magnet. 0.5 mT or 5 Gauss contours for magnets of 3.0 T, 1.5 T and 0.7 T MR Image Data (Simplified Notes) MRI uses radio frequency (RF) electromagnetic waves to form images. RF signals carry information through changes in: ○ Frequency: How fast the wave oscillates. ○ Amplitude: The height or strength of the wave. ○ Phase: The time lag between two waves. Example: If one sine wave is at its maximum while another is at its minimum, the phase difference is 180°. Fourier Transforms are mathematical methods used to process the raw data and reconstruct it into an image. Magnetic Field Gradients (Simplified Notes) NMR Signal & Magnetic Field: NMR signal frequency is proportional to the magnetic field strength. A shimmed magnet has a really good uniform magnetic field, so water hydrogen nuclei have nearly the same Larmor frequency. Adding a gradient (G) to the magnetic field introduces variation along one direction (x, y, or z). Gradient is required for NMR signal localization. Gradients: Strength: Measured in T/m or mT/m (magnetic field per unit length). Directions: z-direction: Along the main magnetic field (usually horizontal in clinical MRI). y-direction: Vertical (upward direction). x-direction: Horizontal, perpendicular to the z-direction. Magnetic Field Gradients (Simplified Notes) Gradient in the z-direction: Adding a gradient of strength Gz in the z-direction causes the Larmor frequency (ω) to change based on the position (z) of the nucleus: ω=γ(B0+Gz) γ\gamma: Gyromagnetic ratio ○ B0: Main magnetic field strength ○ z: Position in the z-direction Gradients in other directions: ○ Gradients can also be applied in the x- or y-direction, and the equation adjusts for the respective position (x or y). ○ Gradients can be applied in oblique directions, combining x, y, and z. Purpose:Gradients determine the frequency of nuclei based on their location, enabling spatial localization for imaging. # The Noise During MR Imaging (Simplified Notes) ○ The loud "machine-gun" sound during MR is due to rapid gradient field switching. Staccato sound. ○ Gradient coils expand slightly when the current rapidly switches on and off, producing a loud "click." ○ Rapid changes in current create rapidly changing magnetic fields, generating forces that make the coils move slightly. ○ This process repeats quickly during imaging, causing the continuous rapid-fire clicking sound. Amplification: ○ The enclosed MRI scanner amplifies the noise, making it louder for the patient inside. The RF Pulse (Simplified Notes) Purpose of RF Pulse: The RF pulse is used to excite a specific slice of tissue in MRI. In order to excite a sharp rectangular slice, a special pulse shape is needed. This is called sinc pulse. Perfect Slice: ○ If the pulse is infinitely long, it excites a perfect rectangular slice of tissue, where only nuclei with resonant frequencies in a certain range are excited. Practical Considerations: ○ Since an RF pulse cannot be infinitely long in practice, the slice will not be a perfect rectangle, but pulse shaping can improve the slice. ○ The strength and time duration of the RF pulse determines the flip angle of the magnetization. (how much the magnetization rotates). 3D Imaging (Simplified Notes) Adding a Third Gradient: ○ A phase gradient is added in the third direction (slice direction) to make the phase dependent on position in 3D. ○ This creates a 3D image by collecting one phase at a time. Slice Encoding Gradient: ○ The slice encoding gradient can be used to enhance the 3D data. Image Contrast (Simplified Notes) Signal Intensity: Signal intensity in MRI depends on the nuclear density (number of protons in a unit volume). It also depends on the pulse sequence used and specific factors like T1 and T2 relaxation times. The timing and sequence determine which factor (e.g., T1, T2) affects the signal the most. The image is then named based on the dominant parameter affecting the signal (e.g., T1-weighted or T2-weighted images). ○ Parameter Dependence: ○ Signal intensity can also depend on: Diffusion T2* Chemical composition (e.g., spectroscopic imaging) Perfusion Magnetization transfer Phase Weighted Images example: ○ T1-weighted image: Useful for anatomy on a clinical scanner. ○ T2-weighted image: Better for anatomy in high-field scanners. Contrast Agents (Simplified Notes) Contrast agents (e.g., gadolinium, manganese, iron compounds) are used to alter T1 and/or T2 relaxation times for better image clarity. The decrease in T1 or T2 is dependent on the concentration of the contrast agent. Harmful Effects of MRI (Simplified Notes) SAR (Specific Absorption Rate): Measures the energy absorbed by an RF field in a mass of tissue. (units: W/kg). ○ SAR increases with higher field strength and larger flip angles. Side Effects: ○ Rapidly changing gradient fields can cause: Skin twitching or pain. Dizziness and possible vomiting. ○ Moving near strong magnetic fields can cause metal objects to fly toward the magnet, posing risks. Functional Magnetic Resonance Imaging (fMRI) fMRI helps study brain activity during specific tasks, like: ○ Watching something new or familiar. ○ Listening to repetitive words. It is used to research brain networks and their interconnections. In recent years Researchers are trying to find links between brain functionality and neurodegenerative diseases like dementia or multiple sclerosis. Source of Signal in fMRI Oxyhemoglobin (fully oxygenated blood) is diamagnetic (repels magnetic fields). Deoxyhemoglobin (non-oxygenated blood) is paramagnetic (attracted to magnetic fields). Deoxyhemoglobin acts as a natural contrast agent. Its concentration changes with oxygen levels and tissue metabolism, reflecting brain activity. The signal produced is called BOLD (Blood Oxygen Level Dependent). BOLD Signal in fMRI BOLD signal changes are very small but become larger with higher magnetic field strength. Requires fast pulse sequences for accurate detection. Tasks must be repetitive with no learning or adaptation between tasks. fMRI is advancing in linking brain function to anatomical changes. Visual Stimulation in fMRI A subject views a picture at specific time intervals. Data is analyzed using SPM (Statistical Parametric Mapping) software. Maps brain activity during the task. Slice Axis A slice is usually assumed to be made in the z-direction for simplicity. This assumption is just for convenience; slices can be made in the x-, y-, or any arbitrary direction by changing the direction in the equations. Slice Axis- Encoding Gradient A slice-encoding gradient pulse is applied in the z-direction just before the RF pulse. This causes resonant frequencies of nuclei to vary in the z-direction: ω=γ(B0+Gz) During the RF pulse, a specific range of z-values can be excited to create a slice. The gradient is turned off after the RF pulse. Spatial Encoding 2D Image uses three orthogonal axes for spatial encoding: 1. Slice encoding direction 2. Frequency encoding direction 3. Phase encoding direction The directions can be chosen in any orientation, as long as they are perpendicular to each other. Slice Axis (z-direction for an axial image) A slice encoding gradient pulse is applied, causing Larmor frequencies of nuclei will be different based on their position along the gradient (z-axis). ω=γ(B0+Gz) The RF pulse frequency will then select a specific slice position. Slice Thickness Limitation Slice profile isn’t perfect, causing nuclei outside the slice to be excited, leading to blurring of the slice thickness. When imaging multiple slices in a pulse sequence: 1. One slice is excited, and partial data is collected. 2. While waiting for the magnetization (alignment of magnetic moments) to return to equilibrium, the next slice is excited and more data is collected. 3. Some nuclei near the slice boundary may be excited, causing cross-talk. Cross-talk happens when adjacent slices are excited before nuclei return to equilibrium. To reduce cross-talk, gaps are typically left between slices. The Phase Axis y The phase gradient is briefly switched on, causing the nuclear precession frequency to change depending on position along the phase gradient (usually in the y-direction). After the gradient pulse ends, the frequency returns to normal, but the phase difference remains. The phase difference is calculated using: ϕ=γ(B0+Gy)ty Larger phase differences can be achieved by increasing the gradient pulse strength or duration. The largest phase differences occur farthest from the center along the gradient direction. One line of k-space (phase space) is collected at a time, meaning one phase value is collected in each cycle. This process needs to be repeated for every line of k-space. For example, to create a 128x128 pixel image, 128x128 lines of k-space must be collected. The Frequency Axis x The frequency gradient encodes the position along the frequency axis (typically the x-direction). For the x-direction: ω=γ(B0+Gx) (B0 + Gx ) The frequency gradient stays on during data collection, also known as the "read-out" direction. The NMR system uses the frequency data to determine the position of the signal along the frequency axis. 2D-Data Collection Process - Summary Data is collected using spatial encoding in 3 directions: 1. Slice encoding (gradient in the z-direction) 2. Phase encoding (gradient in the y-direction) 3. Frequency encoding (gradient in the x-direction) and "Read-Out" data After collection, signals based on their phase and frequency are organized in k-space. An inverse Fourier transform of the data from k-space creates the image in xy space (or image space).