Microbial DNA Technologies PDF
Document Details
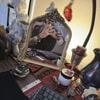
Uploaded by rafawar1000
Florida Atlantic University
David Planchet
Tags
Summary
This document discusses microbial DNA technologies. The chapter introduces DNA technologies that have made projects like cloning a gene in E. coli, assembling a custom gene sequence, and genome editing possible. It explores topics such as spinning stronger silk from spidroin protein, cloning a gene, recombinant DNA, and restriction enzymes.
Full Transcript
17 Microbial DNA Technologies Spinning Stronger Silk S pider silk is an astounding fiber, lightweight and elastic, yet strong enough to suspend a dangling spider or capture prey for its next meal. Materials scientists have long sought to replicate spider silk in the laboratory to exploit its propert...
17 Microbial DNA Technologies Spinning Stronger Silk S pider silk is an astounding fiber, lightweight and elastic, yet strong enough to suspend a dangling spider or capture prey for its next meal. Materials scientists have long sought to replicate spider silk in the laboratory to exploit its properties. Among the potential applications are lightweight bulletproof vests and medical devices like artificial ligaments, bandages, and sutures. Spider silk fibers are composed of many copies of a single protein, spidroin. In the silk gland, spidroin is in a highly concentrated solution, rather than a fiber. As the protein solution moves through the spider’s silk glands and spinning ducts, it is acidified and dehydrated. Individual protein molecules change conformation, aggregate, and elongate to become the silk fiber. Industrial production of fibers requires large quantities of spidroin, more than is feasible to extract from even an army of spiders. By inserting the gene that encodes spidroin into bacteria, yeast, or cultured animal cells, large amounts of recombinant spidroin protein are produced. Even goats have been engineered to make spidroin in their milk! But the challenge in making spider silk is to spin the spidroin solution to a fiber. It turns out that spidroin proteins from different types of spiders aren’t exactly the same. They vary somewhat in their amino acid sequence, and as a consequence, their properties vary. Although several types can be prepared as recombinant proteins, all have some regions that are insoluble or less responsive to the pH changes that occur during fiber spinning. The result is a silk that is less elastic or weaker than the original spider-made version. So scientists designed the ideal spidroin consisting of the N-terminal and middle portions of the protein from Euprosthenops australis, the nursery web spider, and the C-terminal portion from Araneus ventricosus, an orb-weaving spider. But how is a new hybrid, or chimeric, gene made? In this case, having the spidroin genes from both spider species already cloned made the task simpler. The polymerase chain reaction (PCR) makes many copies of a gene, or in this case, part of a gene. These parts can be assembled to build the complete gene, including a promoter “on” switch and an intact open reading frame that encodes the protein exactly as it has been designed. After introducing this hybrid gene to E. coli, flipping the “on” switch, and purifying the chimeric spidroin protein, 1 kilometer of spider silk fiber can be spun from the spidroin purified from just 1 liter of recombinant E. coli culture. ©David Planchet In this chapter, we introduce some of the DNA technologies that have made this type of project possible. From cloning a gene in E. coli (figure 17.1), to assembling a custom gene sequence, to genome editing, the field of biotechnology has been revolutionized by the ease and simplicity of in vitro DNA technologies. 1 Isolate DNA to be cloned. 2 Use a restriction enzyme or PCR to generate fragments of DNA. 3 Generate a recombinant molecule by inserting DNA fragments into a cloning vector. 4 Introduce recombinant molecule into new host, which expresses the gene. Linear vector Vector New host Figure 17.1 Steps in Cloning a Gene. Each step shown in this overview is discussed in more detail in this chapter. MICRO INQUIRY Which of the DNA molecules shown are recombinant? 405 wil11886_ch17_405-424.indd 405 23/10/18 12:15 pm 406 CHAPTER 17 | Microbial DNA Technologies Readiness Check: Based on what you have learned previously, you should be able to: ✓ Identify the key structural elements of DNA, RNA, and protein (section 13.2) ✓ Diagram the flow of cellular information from gene to protein as ✓ ✓ ✓ ✓ well as protein localization (e.g., subcellular localization or export) (sections 13.5, 13.7, and 13.8) Explain the general structural differences in a eukaryotic gene and a bacterial gene, including regulatory, coding, and noncoding sequences (sections 13.3, 13.5, and 15.3) Describe the capacity of proteins to recognize and bind to DNA (sections 13.2 and 14.2) List three differences between a plasmid and a chromosome (sections 3.6 and 16.6) Describe DNA repair and homologous recombination reactions (sections 16.3 and 16.4) such as vitamins, as discussed in chapter 42. The underlying principle behind these techniques is that the genetic code is universal. Whether it is from E. coli, a worm, or a tomato, DNA has the same structure and function. Genes can be moved from one organism to another and still encode the same protein. Similarly, enzymes that act on DNA can perform their catalytic role on DNA from any source. The earliest methods for DNA manipulation used enzymes and bacterial cells to modify and amplify DNA in a process known as DNA cloning. DNA molecules with segments that originate from different organisms are termed recombinant. More recent methods bypass the need for cells and use purified enzymes to create recombinant DNA molecules in vitro. As researchers envision more complex engineered cells, the components of the cloner’s toolbox (e.g., regulatory sequences, structural genes, and enzymes) are being standardized for widespread use. Restriction Enzymes 17.1 Key Discoveries Led to the Development of DNA Cloning Technology After reading this section, you should be able to: a. Explain how restriction enzymes recognize and digest DNA to create either blunt or sticky ends b. Diagram the reaction catalyzed by reverse transcriptase and describe its application to biotechnology c. List the three features of a cloning vector and why these elements are needed d. Differentiate between plasmids, phage-based cloning vectors, cosmids, and artificial chromosomes in terms of structure and application e. Explain how a piece of foreign DNA is recombined in vitro into a cloning vector f. Identify host cells commonly used for transformation g. Compare two common techniques by which recombinant DNA constructed in vitro is introduced into host cells Genetic engineering refers to the modification of the genetic code of a living organism. For centuries, crop improvement and breeding animals for desirable traits constituted the only form of genetic engineering. But in the mid to late twentieth century, the identification of DNA as the chemical basis for heredity and advances in laboratory methods for manipulating DNA opened new avenues for scientists. Properties like rapid growth and easy laboratory handling made microbes like E. coli and the yeast Saccharomyces cerevisiae popular genetic models. The application of DNA technologies to the study of microbes takes two forms. Microbes can be altered to allow scientists to ask direct questions about how they function in the environment and how the basic processes of life work. Additionally, microbes can be treated as factories for the production of biological molecules like insulin (a protein) and small molecules, wil11886_ch17_405-424.indd 406 One of the first breakthroughs leading to DNA cloning was the discovery by Werner Arber and Hamilton Smith in the late 1960s of bacterial enzymes that cut double-stranded DNA. These enzymes, known as restriction enzymes or restriction endonucleases, recognize and cleave specific sequences (figure 17.2). Each restriction enzyme has a four to eight base pair sequence as its substrate; and hundreds of different restriction enzymes have been purified and are commercially available. These enzymes can be used to prepare DNA fragments containing specific genes or portions of genes. For example, the restriction enzyme EcoRI, isolated by Herbert Boyer in 1969 from Escherichia coli, cleaves DNA between G and A in the base sequence 5′-GAATTC-3′ (figure 17.3). Because DNA is Figure 17.2 Restriction Endonuclease Bound to DNA. In this structure of HincII bound to DNA, the two strands of DNA are colored light and dark blue. The enzyme’s two identical subunits are colored red and yellow. The symmetry of the palindromic DNA substrate is mirrored in the symmetry of the enzyme. 23/10/18 12:15 pm 17.1 Key Discoveries Led to the Development of DNA Cloning Technology 407 Cut Cut GAA T TC C T T AAG GAA T TC C T T AAG Cut Cut 5′ 3′ sticky ends. In contrast, cleavage by restriction enzymes such as AluI and HincII leaves blunt ends. Because the reaction occurs in the common structural component of DNA (the phosphatesugar backbone), the information in DNA (the sequence of nitrogenous bases) is preserved. Analysis of restriction enzyme digests is typically done by agarose gel electrophoresis, as discussed in Techniques & Applications 17.1. A few restriction enzymes and the DNA sequence that they recognize are listed in table 17.1. Note that enzymes are named after the bacterium from which they originate. Restriction Endonucleases 3′ 5′ Genetic Cloning and cDNA Synthesis 5′ G CT TAA 3′ AA T TC G G CT TAA AA T TC G 3′ 5′ Figure 17.3 Restriction Endonuclease Action. The cleavage catalyzed by the restriction endonuclease EcoRl. The enzyme makes staggered cuts on the two DNA strands to form sticky ends. MICRO INQUIRY Examine the uncut piece of DNA shown in the upper half of this figure. Where would an exonuclease cleave? antiparallel, this sequence is also present on the complementary strand of DNA. When EcoRI hydrolyzes the phosphodiester bonds between the G and A residues, unpaired 5′-AATT-3′ remains at the end of each strand; such overhangs are termed Table 17.1 An important advance in cloning DNA came in 1972, when David Jackson, Robert Symons, and Paul Berg reported that they had successfully generated recombinant DNA molecules. They allowed the sticky ends of fragments to anneal—that is, to base pair with one another (figure 17.4), and then covalently joined the fragments with the enzyme DNA ligase (see figure 13.15). Within a year, cloning vectors into which foreign DNA fragments could be inserted had been developed. Such plasmids replicate within a microbial host, thereby maintaining the cloned fragment of DNA. Early Genetic Engineering Experiment Once it was demonstrated that foreign genes could be inserted into cloning vectors, biologists sought to clone specific genes from various organisms. However, it was evident that cloning eukaryotic DNA into bacterial hosts would be problematic. This is because eukaryotic pre-mRNA must Some Restriction Endonucleases and Their Recognition Sequences 1 The arrows indicate the sites of cleavage on each strand. MICRO INQUIRY: Which enzymes yield blunt ends? Which yield sticky ends with a 5′ overhang? Which generate a 3′ overhang? wil11886_ch17_405-424.indd 407 23/10/18 12:15 pm 408 CHAPTER 17 | Microbial DNA Technologies Untreated DNA in lane 1 TECHNIQUES & APPLICATIONS 17.1 Gel Electrophoresis Agarose and polyacrylamide gel electrophoresis is routinely used to separate DNA fragments by size. When DNA molecules are placed at the negative end of an electrical field, they (a) migrate toward the pole with a positive charge. Each fragment’s migration rate is determined by its molecular weight so that the smaller a fragment is, the faster it moves through the gel. Migration rate is also a function of gel density. In practice, this means that high concentrations of gel material (agarose or acrylamide) provide better resolution of small fragments and vice versa. Agarose provides good resolution of DNA molecules larger than 500 base pairs (bp), while polyacrylamide can resolve fragments that differ by 1 base pair. Plasmid DNA that has not been digested with restriction enzymes is usually DNA digested with one enzyme in lane 2 Restriction enzymes supercoiled and does not migrate to a position corresponding DNA digested with a different enzyme to its molecular weight. Comparison to molecular weight in lane 3 standards is therefore only valid for linear DNA. (–) 1 2 3 4 Wells No. of base pairs in band 10002 4001 Larger 3001 2017 1517 1200 1000 900 800 700 600 517 500 400 Untreated DNA in lane 1 DNA digested with one enzyme in lane 2 300 200 100 Restriction enzymes DNA digested with a different enzyme in lane 3 Smaller (+) (b) (a) DNA migrates toward positive electrode. Gel Electrophoresis of DNA. (a) A DNA fragment with recognition sites for two restriction endonucleases. Each enzyme recognizes a specific sequence and cuts the DNA at that position, to create two fragments that can be separated and sized on an agarose gel. (b) DNA is loaded into wells on one end of an a garose gel. When an electrical current1 is passed through 2 3 4 the gel, the negatively charged DNA migrates toward the positive pole. Larger fragments, measured in numbers of base pairs, (–) migrate more slowly than the smaller (shorter) fragments. The same DNA fragment is used in lanes 1, 2, and 3. It is Wells gel reveals the separation pattern of the DNA fragments. The size of a intact in lane 1, and cut with two different enzymes in lanes 2 and 3. The stained given DNA band can be determined by comparing it to a known setNo. ofofmolecular weight markers (lane 4) called a ladder. base pairs in band MICRO INQUIRY Approximate the sizes of the DNA fragments in lanes 1, 2, and 3 using the size standards in lane 4. Convince yourself that when the 10002 Larger 4001 The sizes of the fragments in each lane should add up to the size of the uncut fragment in lane 1 is digested with restriction enzymes, no DNA is lost. 3001 fragment. 2017 1517 1200 1000 900 800 700 600 517 500 400 be processed (e.g., introns spliced out), and bacteria lack the molecular machinery to perform this task. In 1970 Howard Temin and David Baltimore independently discovered300 the 200 100 enzyme that solved this dilemma. They isolated the enzyme (+) retroviruses. These viruses reverse transcriptase (RT) from have an RNA genome that is copied into DNA prior to replica(b) DNA migrates toward tion. The mechanism by which reverse transcriptase accompositive electrode. plishes this is outlined in figure 17.5. Processed mRNA can be extracted from a eukaryotic cell and used as a template for complementary DNA (cDNA) synthesis in vitro. The resulting wil11886_ch17_405-424.indd 408 cDNA can then be cloned, without the need for RNA processing. Retroviruses (section 26.7) cDNA Smaller Cloning Vectors Cloning depends on the propagation of many copies of the nucleotide sequence of choice. To accomplish this, cloning vectors, DNA elements that replicate and can carry additional genetic cargo, had to be developed. The major types of vectors are plasmids, bacteriophages and other viruses, cosmids, and 23/10/18 12:15 pm 17.1 Key Discoveries Led to the Development of DNA Cloning Technology 409 Plasmid vector Donor DNA G A C T T A A G A T lacZ gene GAATTC GAATTC GAATTC CTTAAG CTTAAG CTTAAG T Donor DNA cut with EcoRI C Plasmid cut with EcoRI AATTC G G G AATTC C TTAA G G C TTAA Donor DNA fragments C T T A A G AmpR C T A A T (a) A G CT A T T C G TA A AA T C T G G AmpR C T T A A Complementary base pairing followed by ligation Select AmpR colonies Transform E. coli G A A CT T TA T A C G AmpR (b) Screen transformants GAAT Blue colonies have vector only. (c) White colonies have vector with cloned DNA insert. C T G A C T T A Recombinant DNA molecule Figure 17.4 DNA Cloning. The construction of a recombinant plasmid. Treatment of both the cloning vector and the donor DNA with the same restriction endonuclease results in complementary sticky ends on both molecules. The scale of the sticky ends of the fragments and plasmid has been enlarged to illustrate base pairing. (a) The electron micrograph shows a plasmid that has been linearized by a restriction enzyme, and a donor DNA fragment. (b) The micrograph shows an intact plasmid. (c) After transformation, E. coli cells are plated on medium containing ampicillin and X-Gal. Ampicillin ensures that only ampicillinresistant transformants grow; X -Gal enables the visualization of colonies that were transformed with recombinant vector (vector + insert, white colonies). (a, b) ©Huntington Porter and David Dressler/Time Life Pictures/Getty Images; (c) ©Edvotek, Inc. www.edvotek.com MICRO INQUIRY What would you conclude if you obtained only blue colonies after cloning into a vector that enables blue/white screening of transformants? Why might such a result occur? wil11886_ch17_405-424.indd 409 23/10/18 12:15 pm 410 CHAPTER 17 | Microbial DNA Technologies A A A A A A 5′ 3′ mRNA Add a poly-dT primer. 3′ Plasmids 5′ T T T T T T A A A A A A 3′ 5′ Add reverse transcriptase + dNTPs to synthesize a complementary DNA strand. 3′ 5′ T T T T T T A A A A A A 3′ 5′ Add RNaseH to cut up the RNA and generate RNA primers. 5′ 3′ T T T T T T Add DNA polymerase and DNA ligase to synthesize the second DNA strand. 3′ 5′ T T T T T T A A A A A A 3′ 5′ Double-stranded cDNA Figure 17.5 Synthesis of cDNA. A poly-dT primer anneals to the 3′ end of mRNAs. Reverse transcriptase then catalyzes the synthesis of a complementary DNA strand (cDNA). RNaseH digests the mRNA into short pieces that are used as primers by DNA polymerase to synthesize the second DNA strand. The 5′ to 3′ exonuclease function removes all of the RNA primers except the one at the 5′ end (because there is no primer upstream from this site). This RNA primer can be removed by the subsequent addition of another RNase. After the double-stranded cDNA is made, it can be inserted into vectors, as described in figure 17.4. MICRO INQUIRY Why must introns be removed from eukaryotic DNA before it can be expressed in a bacterium? artificial chromosomes. Each type has its own advantages and applications, so the selection of the proper cloning vector is critical to the success of any cloning procedure. Most cloning vectors share three important features: an origin of replication; a region of DNA that bears unique r estriction sites, called a multicloning wil11886_ch17_405-424.indd 410 site; and a selectable marker. These elements are described next in the context of plasmids, the most frequently used cloning vectors. Construction of a Plasmid Vector Plasmids make excellent cloning vectors because they replicate autonomously (i.e., independently of the chromosome) and are easy to purify. They can be taken up by microbes by conjugation, transformation, or electroporation. Many different plasmids are used in biotechnology, all derived from naturally occurring plasmids that have been genetically engineered (figure 17.6). Plasmids (section 3.6); Bacterial conjugation requires cellcell contact (section 16.6); Bacterial transformation is the uptake of free DNA from the environment (section 16.7) The origin of replication (ori) allows the plasmid to replicate in the microbial host independently of the chromosome, and it dictates the copy number, or the number of plasmid molecules present in a cell. High copy number facilitates plasmid purification; an E. coli high copy number plasmid like pUC19 is present at hundreds of copies per cell. Some plasmids have two origins of replication, each recognized by different host organisms. These plasmids are called shuttle vectors because they can be transferred, or “shuttle,” from one host to another. YEp24 is a shuttle vector that can replicate in yeast (Saccharomyces cerevisiae) and in E. coli because it has the 2μ circle yeast replication element and the E. coli origin of replication (figure 17.6). Following the uptake of vector by host cells, it is necessary to discriminate between cells that successfully imported the plasmid (transformants) from those that did not (nontransformants). This is achieved by the presence of a gene on the plasmid encoding a protein needed for the cell to survive under certain conditions. Such a gene is called a selectable marker. In the case of pUC19, the selectable marker, AmpR, encodes the ampicillinresistance enzyme. Because E. coli is normally susceptible to ampicillin, only those cells that have taken up plasmid (i.e., transformants) will grow when plated on agar containing ampicillin. The shuttle vector YEp24 bears both the AmpR gene to be used when selecting E. coli transformants and URA3, which encodes a protein essential for uracil biosynthesis in yeast. Therefore this plasmid must be used in strains of S. cerevisiae that are uracil auxotrophs, otherwise it would be impossible to select, or identify, transformants. To clone a fragment of DNA into a plasmid, both the fragment and plasmid are cut with the same restriction enzyme so that compatible sticky ends are generated. It is essential that these restriction enzymes cut at only one place in the plasmid. Cleavage at a unique restriction site generates a linear plasmid. Alternatively, two different, unique sites may be cleaved and the DNA sequence between the two sites replaced with cloned DNA. Cloning vectors have been designed with many restriction sites clustered in a single region called the multicloning site (MCS) (figure 17.6). When the plasmid and the DNA to be cloned are cut with one of these enzymes, the compatible sticky ends hydrogen bond. Incubation in the presence of DNA ligase 23/10/18 12:15 pm 17.1 Key Discoveries Led to the Development of DNA Cloning Technology 411 BclI Aat-ZraI Aat-ZraI BclI MfeI 2µ pR Acm 2µ c SnaBI o ri o ri BspEI T et R BspEI EagI BamHI results in the synthesis of phosphodiester bonds between the cloned DNA fragment and the vector (figure 17.4). Unfortunately, the ligation of foreign DNA into a vector is never 100% efficient. When the ligation mixture is introduced into host cells, cells that carry a plasmid without the insert DNA must be distinguished from those that carry a plasmid into which DNA was successfully cloned. To accomplish this, the MCS in plasmids such as pUC19 is engineered within the 5′ end of the lacZ gene. lacZ encodes β-galactosidase (β-gal), an enzyme that cleaves the disaccharide lactose into galactose and glucose (see figure 14.2). An alternative substrate for the enzyme is X-Gal (5-bromo-4chloro-3-indolyl-β-D-galactopyranoside), a chemical that is colorless until hydrolyzed by β-gal, when it turns blue. When DNA has been inserted into the MCS, the lacZ gene is interrupted and a functional β-gal is not produced. Plating the cells on a selectivedifferential growth medium permits a rapid visual determination of whether the cloning procedure has been successful. Ampicillin in the medium requires that the cells carry the plasmid, and X-Gal in the medium turns colonies blue if they can synthesize β-gal. Colonies carrying plasmids with an insert in the MCS, then, will be white, as the insert prevents β-gal synthesis (figure 17.4c). There are a number of other clever ways in which cells with vector can be distinguished from those with vector plus insert; the detection of blue versus white colonies, though, is a common approach. Phage and Viral Vectors Phage vectors are phage genomes that have been genetically modified to include useful restriction enzyme recognition sites for the insertion of foreign DNA. Once DNA has been inserted, the recombinant phage genome is packaged into viral capsids and used to infect host cells. The resulting phage lysate consists of thousands of phage particles that carry cloned DNA as well as the genes needed for host lysis. Common vectors are derived from the E. coli bacteriophage lambda (λ), which has a double-stranded DNA genome. Bacteriophage lambda: a temperate bacteriophage (section 26.2) wil11886_ch17_405-424.indd 411 Figure 17.6 The Cloning SnaBI Vectors pUC19 and YEp24. YEp24 Restriction sites that are present (7,769bp) HpaI only once inHpaI each vector are shown. pUC19 replicates only in E. coli, while YEp24 replicates in UR UR both E. coli and S. cerevisiae. T et R MCS is multiple cloning site. EagI SmaI SphI SphI XbaI A3 YEp24 (7,769bp) PvuII XbaI A3 PvuII ori ori Amp R Amp R R p Am MfeI DNA le irc α cZ α cZ pUC19 (2,686bp) EcoRI SacI KpnI MCS SmaI BamHI XbaI SalI PstI SphI HindIII DNA le irc la EcoRI la SacI KpnI MCS SmaI BamHI XbaI pUC19 SalI (2,686bp) PstI SphI HindIII SmaI BamHI MICRO INQUIRY Which plasmid is a shuttle vector? Why? Genetic engineering of animal cells is accomplished by the use of viral vectors. These recombinant viruses are shuttle vectors that replicate in E. coli as bacterial plasmids, but they also direct the assembly of mature infective virus particles to shuttle the DNA to an animal cell. Commonly used viruses for mammalian cells are retroviruses, lentiviruses, and adenoviruses. Cosmids DNA inserts larger than about 15,000 bp to 25,000 bp cannot be stably maintained in plasmids and phage vectors, respectively. Instead, cosmids can be used to clone such large fragments of DNA. These engineered vectors have a cos site from phage and a selectable marker, origin of replication, and MCS from plasmids (thus the term “cos-mid”). These hybrid vectors replicate as plasmids within the host cell, but the presence of the cos sites means that the vector can be packaged into phage capsids and transferred to new host cells by transduction. Transduction is virus-mediated DNA transfer (section 16.8) Artificial Chromosomes Artificial chromosomes are used when particularly large fragments of DNA must be cloned, as when constructing a genomic library (section 17.3). In fact, the genomic library containing the human genome was constructed in bacterial artificial chromosomes (BACs), which then enabled nucleotide sequencing. BACs were also used in the construction of a synthetic microbial genome (Techniques & Applications 17.2). Like natural chromosomes, artificial chromosomes replicate only once per cell cycle. Yeast artificial chromosomes (YACs) were developed first and consist of a yeast telomere (TEL) at each end, a centromere sequence (CEN), a yeast origin of replication (ARS, autonomously replicating sequence), a selectable marker such as URA3, and an MCS to facilitate the insertion of foreign DNA (figure 17.7a). YACs are used when extraordinarily large DNA pieces (up to 1,000 kb) are to be cloned. BACs were developed, 23/10/18 12:15 pm 412 CHAPTER 17 | Microbial DNA Technologies TECHNIQUES & APPLICATIONS 17.2 How to Build a Microorganism Biotechnology has repeatedly demonstrated how useful the genetic modification of an organism can be. From the production of recombinant human insulin to recombinant indigo (the dye used in blue jeans), it is clear that genetic engineering can be used for the production of a wide range of products. But what if, rather than modifying one gene at a time, it were possible to design the ideal genome? The construction of such a genome would enable synthesis of the precise genotype best suited for the application at hand, whether that is generating biofuels or pharmaceuticals. The first step in reaching this goal is to build a genome from scratch. Molecular biologists at the J. Craig Venter Institute (JCVI) accomplished this in 2008 with one of the smallest bacterial genomes, that of Mycoplasma genitalium. The assembly of chemically synthesized DNA was performed in a series of BACs and then YACs to generate the entire bacterial chromosome in a yeast host. A year later, JCVI scientists showed it was possible to “transplant” a bacterial genome from a yeast host into a bacterium. So by 2010 the team was ready to go the distance and construct a synthetic genome and transplant it into a bacterial host. The JCVI researchers used M. mycoides as their model genome. At 1.1 million base pairs (Mb), this microbe’s genome is about twice the size of M. genitalium. The first step was to chemically synthesize 1,078 oligonucleotides, each 1,080 bp long (box figure). If lined up end to end, these oligonucleotides would represent the entire genome. Importantly, 80 bp at each end of every oligonucleotide overlapped with its neighbor, so within an E. coli host, these could be assembled in the same order as that found in the native chromosome. Assembly in groups of 10 generated 109 chromosomal fragments, each about 10,000 bp. These were then transformed into yeast, where they were joined into 11 larger cassettes of 100,000 bp each. Finally, these were recombined to yield the entire genome. In addition to the native genes, four nucleotide sequences, called watermarks or barcodes, were included. Just as a barcode is used to identify an object, these nucleotide sequence barcodes were added to identify the genome as artificial. The final step was to transplant the synthetic genome from the yeast host to the final bacterial host, M. capricolum. in part, because YACs tend to be unstable and may recombine with host chromosomes, causing mutations and rearrangement of the cloned DNA. Although BACs accept smaller DNA inserts of up to 300 kb they are generally more stable. They are based on the F fertility factor of E. coli (see figure 16.16). The example shown in figure 17.7b is typical in that it includes genes that wil11886_ch17_405-424.indd 412 Just as in any other cloning experiment, this depended on the presence of a selectable marker. In this case, a gene that conferred resistance to the antibiotic tetracycline was included in the synthetic genome. Upon selection of tetracycline-resistant M. capricolum cells bearing the M. mycoides genome, a series of verification tests were performed. These involved PCR and analysis of restriction endonuclease digestion patterns. Once it was confirmed that the host cell was carrying the synthetic genome, all that was left to do was to name the new organism. The JCVI scientists dubbed their new organism M. mycoides JCVI-syn1.0. Source: Gibson, D. G., et al., 2010. Creation of a bacterial cell controlled by a chemically synthesized genome. Science. 329:52. Synthesize 1,078 oligonucleotides. 1,080 bp each, ends overlap Assemble in groups of 10. 109 cassettes, each about 10,000 bp (10 K) Assemble in groups of 10. 11 cassettes, each about 100,000 bp (100 Kb) Assemble into complete genome. 1.1 Mb Genome Transplant genome. M. mycoides JCVI-syn1.0 cells M. capricolum cells ensure a replication complex will be formed (repE), as well as proper partitioning of one newly replicated BAC to each daughter cell (sopA, sopB, and sopC). It also includes features common to many plasmids such as an MCS within the lacZ gene for blue/ white colony screening and a selectable marker, in this case for resistance to the antibiotic chloramphenicol (CmR). 23/10/18 12:15 pm 17.2 Polymerase Chain Reaction Amplifies Targeted DNA 413 TEL TRP1 ARS CEN MCS URA3 TEL (a) Yeast artificial chromosome (YAC) R Cm sop C BsaH I ApaL I BamH I BstE I Hpa I Sph I Hind III Pci I Sfi I lacZα Ahd I Sca I cos so pA re (b) Bacterial artificial chromosome (BAC) Figure 17.7 Artificial Chromosomes Can Be Used as Cloning Vectors. (a) A yeast artificial chromosome and (b) the bacterial artificial chromosome pBeloBAC11. MICRO INQUIRY In what ways does the BAC shown here differ from the plasmid pUC19 shown in figure 17.6? Introducing Recombinant DNA into Host Cells In cloning procedures, the selection of a host organism is as important as the choice of cloning vector. E. coli is the most frequent bacterial host and S. cerevisiae is most common among eukaryotes. Host microbes engineered to lack restriction enzymes and recombination enzymes (RecA in bacteria, and RecA homologues in S. cerevisiae) make better hosts because it is less likely that the newly acquired DNA will be degraded or recombined with the host chromosome. There are several ways to introduce recombinant DNA into a host microbe. Chemical transformation and electroporation are two commonly employed techniques when the host microbe does not have the capacity to be transformed naturally. This is the case with E. coli and most Gram-negative bacteria as well as many Gram-positive bacteria and archaea. In chemical transformation, the host cells are made competent by treatment with divalent cations and then transformed by heat-shocking the cells in a solution containing plasmid. Bacterial transformation is the uptake of free DNA from the environment (section 16.7) Electroporation is a simple technique for transforming bacteria, plant, and animal cells. In this procedure, cells are mixed with the recombinant DNA and exposed to a brief pulse of high-voltage electricity. The plasma membrane becomes temporarily permeable and DNA molecules are taken up by some of the cells. The cells are then grown on media that select for the presence of the cloning vector. wil11886_ch17_405-424.indd 413 1. Describe restriction enzymes, sticky ends, and blunt ends. Can you think of a cloning situation where blunt-ended DNA might be more useful than DNA with sticky ends? 2. What is cDNA? Why is it necessary to generate cDNA before cloning and expressing a eukaryotic gene in a bacterium? 3. You want to clone a 6,000 bp DNA fragment in E. coli. Which cloning vectors would be appropriate? How will you select transformants? 4. Explain the selection for antibiotic resistance followed by blue versus white screening of colonies containing recombinant plasmids. Why must both antibiotic selection and color screening be used? 5. How can one prevent recombinant DNA from undergoing recombination in a bacterial host cell? ori2 pE sopB pBeloBAC11 (7,507 bp) Comprehension Check 17.2 Polymerase Chain Reaction Amplifies Targeted DNA After reading this section, you should be able to: a. Differentiate between a PCR cycle and step, and define the function of each of the three steps used in a PCR cycle b. Explain why PCR results in the amplification of a specific DNA sequence despite many competing sequences c. Explain why PCR generates billions of products that are all the same size d. Contrast real-time, quantitative PCR to end-point collection PCR and identify an application for each e. Summarize the importance of PCR in biology f. Explain how sequences at the 5′ end of an oligonucleotide primer become included in the product when they are not homologous to the template g. Explain how the design of oligonucleotide primers permits seamless cloning The polymerase chain reaction (PCR), invented by Kary Mullis in the 1980s, exploded onto the biotechnology landscape. It changed the way genes are cloned, nucleic acids sequenced, diseases diagnosed, and crimes solved. Why is PCR so versatile and important? Quite simply, it enables the rapid synthesis of billions of copies of a specific DNA fragment from a complex mixture of DNA molecules. By adapting DNA replication to be carried out entirely in vitro, multiple tasks involving enzymatic reactions and bacterial growth are bypassed. Figure 17.8 outlines how PCR works. To make large quantities of a particular gene or other DNA sequence, a process known as amplification, or DNA replication, is performed repetitively in vitro. DNA polymerase requires a template to copy, primers to provide a free 3′-OH, and each of the four deoxyribonucleotide triphosphates (dNTPs). Two oligonucleotide primers are designed so that each primer is complementary to one of the template strands, allowing for both strands to be copied during the reaction. The primers are positioned adjacent to, and flanking, the region to be amplified. Oligonucleotide 23/10/18 12:15 pm 414 CHAPTER 17 | Microbial DNA Technologies primers are single-stranded DNA molecules, generally between 15 and 30 nucleotides long, that are made with a DNA synthesizer. Because primers are based on flanking nucleotide sequences, only DNA with known (or nearly known) sequence can be amplified by PCR. The reaction mixture, then, includes the template DNA (often copies of an entire genome), two primers, a thermostable DNA polymerase, and dNTPs. PCR is a series of DNA replication reactions, called cycles. Each cycle precisely executes three steps in a machine called a thermocycler. In the first step, the double-stranded template DNA is denatured to single-stranded DNA by raising the temperature to about 95°C. Next the temperature is lowered to about 50°C so that the primers can hydrogen bond (anneal) to their complementary 5′ region on the template strands. Primers are included in the reaction at a huge molar excess because they must compete with the other template strand for binding. In the final step, the temperature is raised, usually to 68° to 72°C, so that DNA polymerase can e xtend the primers and synthesize copies of the target DNA sequence using dNTPs. Only sturdy DNA polymerases that can tolerate the repeated temperature fluctuations can be used in PCR. The most commonly used thermostable enzyme is Taq polymerase from the thermophilic bacterium Thermus aquaticus. At the end of one PCR cycle, the targeted sequences on both strands have been copied. When the three-step cycle is repeated (figure 17.8), the two strands from the first cycle are copied to produce four DNA molecules. These are amplified in the third 3′ Target sequence Genomic DNA 3′ 3′ 5′ Step 1 Denaturation: Heat briefly to separate DNA strands. Cycle 1 yields 2 molecules. Step 2 Annealing: Cool to allow primers to hydrogen bond with ends of target sequence. Step 3 Extension: DNA polymerase adds nucleotides to the 3′ end of each primer. Cycle 2 yields 4 molecules. Cycle 3 yields 8 molecules. wil11886_ch17_405-424.indd 414 Start with double-stranded template DNA, present in low amounts, plus dNTPs, Taq polymerase, and 2 primers that are present in high amounts and complementary to the ends of the region that will be amplified. 5′ Primer annealing site 3′ 3′ 5′ 5′ Primers T GC A C C AGC A T C CGA T C A CG T GG T CG T AGGC T AG 5′ 3′ Primer Figure 17.8 The Polymerase New nucleotides With each successive cycle, the relative amount of DNA fragments that end exactly at both primer sites increases. Therefore, after many cycles, the vast majority of DNA fragments only contain the region that is flanked by the 2 primer sites. Chain Reaction (PCR) Technique. During each cycle, oligonucleotides that are complementary to the ends of the targeted DNA sequence bind to the DNA and act as primers for the synthesis of this DNA region. The primers are usually 15 to 30 nucleotides in length. The region between the two primers is typically hundreds of nucleotides in length, not just several nucleotides as shown here. The net result of PCR is synthesis of many copies of DNA in the region flanked by the two primers. MICRO INQUIRY Why, after three cycles, are the majority of amplified DNA molecules (i.e., PCR products) the size defined by the distance between the primers? 23/10/18 12:15 pm 17.2 Polymerase Chain Reaction Amplifies Targeted DNA 415 cycle to yield eight double-stranded products. Thus, each cycle increases the number of target DNA molecules exponentially. Depending on the initial concentration of the template DNA and other parameters such as the G + C content of the DNA to be amplified, it is theoretically possible to produce about 1 million copies of targeted DNA sequence after 20 cycles and over 1 billion after 30 cycles. Pieces ranging in size from less than 100 base pairs to several thousand base pairs in length can be amplified, and the initial concentration of target DNA can be as low as 10−20 to 10−15 M. DNA replication in bacteria (section 13.3) Polymerase Chain Reaction PCR can be performed in either of two ways. If large quantities of a specific piece of DNA are needed, the reaction products are collected and purified at the end of a designated number of cycles. This is sometimes called end-point PCR, and the final number of DNA fragments amplified is not quantitative, meaning that the amount of final product does not reflect the amount of template DNA present at the start of the reaction. End-point PCRs are useful when looking for the presence or absence of a particular DNA, for example, in detecting a pathogen in a clinical laboratory. In contrast, real-time PCR is quantitative; in fact, it is referred to as qPCR. That is, the amount of DNA or RNA template (which is converted to DNA with reverse transcriptase prior to starting PCR) present in a given sample can be determined. This is accomplished by adding a fluorescently labeled probe to the reaction mixture and measuring its signal during the initial cycles. This is when the rate of DNA amplification is exponential. However, as the PCR cycles continue, substrates are consumed and polymerase efficiency declines. So although the amount of product increases, its rate of synthesis is no longer exponential (this is why end-point collection of PCR products is not quantitative). Specially designed thermocyclers record the amount of PCR product generated as it occurs, thus the term realtime PCR. Gene expression studies often rely on real-time PCR because mRNA transcripts can be copied by reverse transcriptase to cDNA, which is then quantified. Therefore the procedure monitors transcription of the gene targeted by the primers. PCR is an essential tool in many areas of molecular biology, medicine, and biotechnology. Because the primers used in PCR target specific DNA, PCR can isolate particular fragments of DNA (e.g., genes) from materials that contain many different genomes, such as soil, water, and blood. This explains why PCR has become an essential part of certain diagnostic tests, including those for Chlamydia, hepatitis, human papillomavirus infections, and other infectious agents and diseases. The tests are rapid, sensitive, and specific. PCR is also employed in forensic science, where it is used in criminal cases as part of DNA fingerprinting technology. Metagenomics provides access to uncultured microbes (section 18.3); Molecular characteristics (section 19.3) Seamless Cloning One limitation to conventional cloning is that it relies on the presence of naturally occurring restriction sites in DNA. A modification to PCR allows for the addition of custom restriction sites onto the ends of DNA to be cloned, thereby simplifying DNA assembly wil11886_ch17_405-424.indd 415 tasks. Remember that in PCR, the purpose of the oligonucleotide primers is to base-pair with the template and provide a free 3′ OH group for the addition of deoxyribonucleotides as the new DNA strand is synthesized. It is imperative that the primer hybridizes to the template precisely for 15 to 20 bases at the 3′ end. However, the 5′ end of the oligonucleotide primer is not required to bind to the template for replication to proceed (figure 17.9a). Scientists have exploited this feature of DNA replication to add DNA sequences to the 5′ end of the primer to include, for example, a restriction endonuclease recognition site. Initially the primer will bind and copy the template as illustrated in figure 17.8. In subsequent cycles, when the initial copies are used as templates, those additions on the 5′ end will be copied. Because of the exponential nature of PCR, at the end point of the reaction, all of the product will include the restriction enzyme recognition sequences at the 5′ end of each strand. The result is a DNA fragment copied exactly from the template, with the addition of convenient restriction sites at each end. Taking this idea one step further, Daniel Gibson developed a method in 2009 to eliminate the use of restriction endonucleases entirely. His method, termed Gibson assembly, uses the 5′ ends of oligonucleotide primers to specify the desired junction between the molecules. In a series of reactions diagrammed in figure 17.9b, a vector and insert fragment can be joined efficiently. The insert DNA molecule is prepared by PCR performed with primers that will add DNA that matches the vector. The addition of T5 exonuclease to both the linear vector and the insert cleaves the end of each molecule, leaving extended 3′ sticky ends. Just as in cloning with restriction enzymes, these single-stranded regions can hybridize. Two additional enzymes are added to the reaction, a DNA polymerase and a DNA ligase, to reform a circular molecule. Because the resulting product does not retain any scars or seams from restriction sites in the final product, this approach is sometimes called seamless cloning. With the advent of seamless cloning techniques, scientists are free to envision a DNA molecule and create it in vitro. The procedure diagrammed in figure 17.9b can be expanded to assemble multiple fragments by amplifying each fragment with primers that specify the junction between it and its intended neighbor. An entire operon can be synthesized from promoter and regulatory sequences and genes. Indeed, entire genomes have been synthesized by Gibson assembly, as illustrated in Techniques & Applications 17.2. Comprehension Check 1. Briefly describe the polymerase chain reaction. What is the difference between a step and a cycle? 2. Why is PCR used to detect infectious agents that are often hard to diagnose? 3. How would you use PCR to measure the concentration of a specific gene’s mRNA? 4. Why is it possible to visualize a PCR product on an agarose gel even if the template genome is present at such a low concentration that it cannot be seen? 5. Why is it technically challenging to assemble a large operon using restriction endonucleases? 23/10/18 12:15 pm 416 CHAPTER 17 | Microbial DNA Technologies 5′ AA Single-stranded primer G CT Primers (colored) are designed to base pair with the template at the 3′ end and specify a restriction site at the 5′ end. T 3′ 3′ ACG 5′ TC G Primer hybridized with template After multiple rounds of PCR, the product includes the sequences at the 5′ ends of the primers as double-stranded DNA. CTGCAG AAGCTT TTCGAA GACGTC (a) Introducing restriction sites to a PCR fragment Vector with location of insert indicated Insert DNA Linearize at the site of insertion. PCR amplify with primers to add vector sequences. 5′ 3′ 3′ 5′ 3′ 5′ 5′ 3′ Mix fragments and add T5 exonuclease to chew back 5′ ends. 3′ 5′ 5′ 3′ 3′ 5′ 3′ 5′ Sticky 3′ ends will hybridize. 3′ 5′ 3′ 5′ 5′ 3′ 5′ 3′ Add DNA polymerase and DNA ligase to seal gaps. (b) Gibson assembly for cloning Figure 17.9 Polymerase Chain Reaction Using Primers with a 5' Extension. wil11886_ch17_405-424.indd 416 23/10/18 12:16 pm 17.3 Genomic Libraries: Cloning Genomes in Pieces 417 17.3 Genomic Libraries: Cloning Genomes in Pieces After reading this section, you should be able to: a. Explain why genomic libraries are useful b. Outline the construction of a genomic library and how a clone of the gene of interest might be selected When cloning, the DNA to be inserted into a vector can be obtained in several ways. It can be synthesized by PCR using the microbe’s chromosome as template, as discussed in section 17.2, Plasmid AmpR Restriction enzyme cleavage Fragments a AmpR a b Recombinant plasmids AmpR AmpR Transform tryptophan auxotroph with plasmids. a or it can be amplified from DNA extracted from a natural environment, like soil or water. PCR requires knowledge of the nucleotide sequence of the DNA to be cloned. However, what if researchers want to clone a gene based on the function of its product but have no idea what its DNA sequence might be? A genomic library must then be constructed and screened. The goal of genomic library construction is to have all the DNA derived from a single organism or isolated from a single environment inserted into a set of cloning vectors. When cloning DNA directly from the environment in this way, a metagenomic library is produced. Genomic and metagenomic library construction starts by fragmenting the source DNA to a size compatible with the particular vector being used. Figure 17.10 illustrates the process with recombinant plasmids, and comparable strategies may be used for bacterial artiGenomic DNA ficial chromosomes and viral vectors. Each fragment is cloned into a separate vector so that each vector now carries a separate piece of chroRestriction enzyme cleavage mosome. When a collection of these recombinant vectors b is introduced (e.g., by transformation) into a population of host cells, each transformant carries a vector that has a different piece of foreign chromosome. Ideally the entire organism’s genome or all the DNA from the sample environment is represented in the collection of recombinant vectors. In this way, iso lated groups of genes can be analyzed and isolated (fig ure 17.10). Metagenomics provides access to uncultured microbes (section 18.3) b Plate bacteria, which grow separate clones. Select transformants on complete medium containing ampicillin. Master plate Replica plate onto medium lacking tryptophan but containing ampicillin Screen genomic library transformants for colonies with tryptophan biosynthetic gene. wil11886_ch17_405-424.indd 417 Only transformants containing the cloned tryptophan biosynthetic gene grow. Figure 17.10 Construction of a Genomic Library and Screening by Phenotypic Rescue. A genomic library is made by cloning fragments of an organism’s entire genome into a vector. For simplicity, only two genomic fragments and recombinant vectors are shown. In reality, a large mixture of vectors with inserts is generated. This mixture is then introduced into a suitable host. Phenotypic rescue is one way to screen the colonies for the gene of interest. It involves using a host with a genetic defect that can be complemented or “rescued” by the expression of a specific gene that has been cloned. MICRO INQUIRY Why are long fragments (e.g., 20,000 bp) of genomic DNA often desired when constructing a genomic library? 23/10/18 12:16 pm 418 CHAPTER 17 | Microbial DNA Technologies Genomic libraries are often constructed to find a gene or genetic element (e.g., small noncoding RNA) with a specific function. In this case, it is necessary to know something about the function of the target gene or genetic element. If the genomic library has been introduced into a microbe that expresses the foreign gene, it may be possible to assay each clone for a specific protein or phenotype. For example, to find genes that encode enzymes needed for the biosynthesis of the amino acid tryptophan from a newly isolated bacterium, the library could be expressed in an E. coli tryptophan auxotroph (figure 17.10). Recall that the growth of tryptophan auxotrophs requires the addition of this amino acid to a defined medium. Following introduction of the genomic library into host cells, those that now grow without tryptophan would be good candidates for the genomic library fragment that possesses the tryptophan biosynthetic genes. Success with this approach depends on the assumption that the activity of the cloned gene product is similar in both organisms. If this is not the case, the host must be the same species from which the library was prepared. In this example, a bacterial mutant lacking the trait in question (e.g., a tryptophan auxotroph) is used as the genomic library host. The genetic complementation of a deficiency in the host cell is sometimes called phenotypic rescue because the mutant phenotype is (at least partially) restored, or “rescued.” Detection and isolation of mutants (section 16.2) If a genomic library is prepared from a eukaryote, a cDNA library is usually constructed. This removes introns and only the protein-coding regions of the genome are cloned. cDNA is prepared (figure 17.5) and cloned into a suitable vector. After the library is introduced into the host microbe, host cells carrying the gene of interest are typically identified by phenotypic rescue or PCR amplification of the gene of interest after the vector has been isolated from candidate transformants. In some cases these approaches are not feasible. Then the researcher must develop a novel approach that suits the particular set of circumstances to screen the genomic library. 17.4 Expressing Foreign Genes in Host Cells After reading this section, you should be able: a. Explain the utility of expression vectors b. Outline the procedure by which a His-tagged protein is generated in vivo and purified in vitro c. Summarize the role of GFP in protein analysis, and differentiate between a transcriptional and a translational GFP fusion When a gene from one organism is cloned into another, it is said to be a heterologous gene. However, to be transcribed, a recombinant, heterologous gene must have a promoter that is recognized by the host RNA polymerase. Translation of its mRNA wil11886_ch17_405-424.indd 418 depends on proper ribosome binding. For instance, if the host is a bacterium and the gene has been cloned from a eukaryote, a bacterial promoter and ribosome binding site must be provided and introns removed. The problems of expressing recombinant genes in host cells are largely overcome with the help of specially constructed cloning vectors called expression vectors. These vectors enable the high-level transcription of a gene because it is cloned adjacent to a particularly “strong” promoter. Often expression vectors contain regulatory regions of the lac operon (or another inducible promoter) so that expression of the cloned genes can be controlled by the addition of inducer molecules. This is important because sometimes high-level expression of a heterologous protein can be toxic to the host cell. The presence of an inducible promoter allows the biotechnologist to grow cells to a certain density before inducing the expression of the cloned gene. Regulation of transcription initiation saves considerable energy and materials (section 14.2) Purification and Study of Recombinant Proteins It is often necessary to isolate the protein product of the cloned gene so that its structure and activity can be studied. In addition, it is sometimes desirable to determine the subcellular localization of a protein. Finally, as discussed in chapter 42, heterologous genes are often expressed for the production of commercially prepared recombinant proteins. Here we discuss some clever ways in which proteins can be purified and visualized in living cells. Protein Purification A common method by which proteins are purified is called polyhistidine tagging, or simply His-tagging. His-tagging involves adding a series of histidine amino acid residues to either the Nor C-terminus of a protein. Most often six residues are added; this is called a 6xHis-tag. Histidine is used because it has a high affinity for metal ions, so a protein bearing multiple consecutive histidine residues will bind preferentially to a solid-phase material, called a resin, which has exposed nickel or cobalt atoms. This permits the separation of the His-tagged protein from other cellular constituents. The process of purifying a His-tagged protein begins by adding the histidine residues to the protein. This is most commonly accomplished by cloning the protein into an expression vector that already has the histidine-encoding sequence present (figure 17.11, step 1). Alternatively, the histidine-encoding sequence can be amplified by PCR and added to the gene of interest. Once the protein-coding nucleotide sequence is fused to the histidine codons, the vector is introduced into the microbe of choice, usually E. coli (step 2). As E. coli grows, the His-tagged protein is produced (step 3). When the cells reach a sufficiently high density, they are lysed by treatment with detergent or enzymes, and all the soluble proteins in the cell—including the His-tagged 23/10/18 12:16 pm 17.4 Expressing Foreign Genes in Host Cells 419 DNA-encoding protein Hi ag s-t Protein-specific sequence 5′ ATG cat cat cac cat cac cac NNNNNNNN... 3′ His-tag Vector Start codon Protein-specific sequence 5′...NNNNNNN cat cat cac cat cac cac TAG 3′ His-tag Stop codon Ligation DNA-encoding protein Hi ag s-t 1 Clone the gene that encodes the protein to be purified into an expression vector with the 6 histidine residues next to the start codon (left) or terminator sequences (right), if the His-tag is to be at the N- or C-terminus, respectively. Vector 2 Introduce the newly constructed plasmid into E. coli or other suitable host by transformation or electroporation. Plasmid Chromosome 3 Culture the bacterial host so that each cell produces many His-tagged protein molecules. His-tagged protein 4 Lyse the bacteria and apply the soluble extracted material to a column with nickel- or cobalt-coated resin. 5 Wash the column with buffer to remove unwanted, unbound proteins and other cellular material. KDa 4 6 Release His-tagged protein from the column with a solution of imidazole. 5 6 94 67 43 30 Purified protein 20.1 14.1 7 Recovery and purity of the His-tagged protein are determined by gel electrophoresis. Lane 1: molecular weight markers, Lane 2: soluble proteins obtained in step 4, Lane 3: proteins eluted in step 5, Lane 4: His-tagged protein released in step 6. Figure 17.11 Construction and Purification of a Polyhistidine-Tagged Protein. wil11886_ch17_405-424.indd 419 23/10/18 12:16 pm 420 CHAPTER 17 | Microbial DNA Technologies protein—are collected. These proteins are mixed with the metal resin that is bound to a column (step 4) so that only the Histagged protein sticks to the resin. The remaining, unwanted material is washed from the resin (step 5). The His-tagged protein is then removed by passing the aromatic compound imidazole through the column (step 6). Depending on the purification system used, the His-tag can be cleaved from the protein while it is attached to the resin, or it can be removed following release of the protein. Often the protein is functional with the His-tag, so it may not be removed. His-tagging is used if the protein is soluble and is found in the cytoplasm. While His-tagging is certainly the most popular approach to purifying recombinant proteins, other approaches exist, especially for purifying membrane-associated proteins. Fluorescence Labeling What if the goal of cloning a gene is to study the regulation or function of the protein product in vivo? It is possible to visualize the activity of a specific promoter (i.e., when the promoter is on or off) as well as observe the localization of a protein by fluorescence microscopy. The development of fluorescent labeling of living cells began when it was discovered that the jellyfish Aequorea victoria produces a protein called green fluorescent protein (GFP). GFP is encoded by a single gene that, when translated, undergoes self-catalyzed modification to generate a strong, green fluorescence. This means that it is easily cloned and expressed in the organism of choice. An entire palette of fluorescent labels is now available. These include mutant versions of the GFP protein that glow throughout the blue-greenyellow spectrum. There are two ways in which the amino acid sequence that encodes fluorescent peptides can be attached to a gene. A transcriptional fusion is used to study gene regulation by replacing the entire protein coding sequence of the gene of interest with the fluorescence-encoding gene (figure 17.12a). This places the expression of the fluorescence gene under the control of the promoter of the replaced gene. Thus, when the promoter is turned “on,” it directs the transcription of the fluorescence gene and the cells glow. If the transcriptional fusion has been made in a multicellular organism, then not only is the timing of promoter activity seen but the specific cell type in which the gene is normally expressed is also determined. On the other hand, a translational fusion is used to determine where a particular protein is localized in the cell. Translational fusions add the GFP-coding sequence to the end of the structural gene of interest. This c reates a chimeric protein—a protein that consists of two parts: the protein being studied and GFP. Now whenever that protein is made, and wherever it goes in the cell, the protein will fluoresce. Of course, care must be taken to ensure that the protein fusion still functions like the original protein. One way to do this is to test for phenotypic rescue of a mutant lacking the structural gene of interest. Once it has been determined that the chimeric protein is fully functional, the cellular “address” of the protein can be determined (figure 17.12b). wil11886_ch17_405-424.indd 420 (a) (b) Figure 17.12 Fluorescent Protein Labeling. (a) A transcriptional fusion places the gene encoding a fluorescent peptide under the control of a promoter, replacing the coding sequence of the gene. In this case, the mCherry fluorescence gene is controlled by the promoter that normally governs transcription of a gene in the actinomycete Streptomyces coelicolor that is expressed only when aerial hyphae form cross walls (septa) that give rise to a chain of spores. Thus red fluorescence is seen uniformly throughout the chain of spores but not in hyphae without septae. (b) A translational fusion generates a chimeric protein consisting of the normal protein attached to the GFP label. Here the FtsZ gene has been fused to GFP. This shows that FtsZ localizes at the septa. Notice that the filament to the right is not forming septae, so FtsZ is not present. (a) ©Mark Buttner (b) ©Klas Flärdh MICRO INQUIRY What special considerations are necessary if one is constructing a translational fusion of a protein known to be exported? Comprehension Check 1. Describe how a genomic library might be screened for a gene that confers the production of an extracellular enzyme that degrades casein, a protein found in milk. (Hint: Agar with skim milk loses its opacity when casein is degraded.) 2. List several reasons why a cloned gene might not be expressed in a heterologous host cell. 3. Think of a situation in which you might want to keep the His-tag on a recombinant protein and a situation in which it would be important to remove it. 4. You are studying chemotaxis proteins in a newly described bacterium. You have cloned a gene that encodes the CheA protein. You need to be sure that this protein localizes to the inside face of the plasma membrane (see figure 14.22). Will you use a transcriptional or translational fusion? Explain your choice. 23/10/18 12:16 pm 17.5 Cas9 Nuclease Is a Precise Tool for Genome Editing 421 17.5 Cas9 Nuclease Is a Precise Tool for Genome Editing After reading this section, you should be able to: a. Distinguish the DNA recognition features of restriction endonucleases and Cas9 nuclease b. Explain how Cas9 nuclease can be directed to cut at a unique site in a genome c. Diagram how a new gene may be inserted into a chromosome by homologous recombination Cas9 genome editing has rapidly become one of the most widely used tools for altering genomes in vivo. Cas9 genome editing is often referred to as CRISPR or CRISPR-Cas9, referencing the bacterial genome element from which it was developed, but in fact, only the Cas9 component is used in editing. Cas9 nucleases are encoded in the genomes of most bacteria and archaea, where they are usually adjacent to a CRISPR locus, clustered regularly interspaced short palindromic repeats. As the mechanistic details of Cas9 function were discovered, two research groups, one led by Jennifer Doudna and Emmanuelle Charpentier and the other by Feng Zhang, sought to adapt Cas9 for genome editing. In this process, genomic DNA can be directly modified and the procedures are general enough to be used for any cell into which DNA can be introduced and expressed. Responses to viral infection (section 14.6) Like restriction enzymes, Cas9 is an endonuclease that cuts both strands of a target DNA. However, there is an important difference between the two types of nucleases, in terms of how they recognize their target sequence in double-stranded DNA. Restriction enzymes recognize four to eight base pairs through contacts between the DNA molecule and amino acid side chains in the enzyme (figure 17.2). Cas9, however, is a ribonucleoprotein consisting of a polypeptide and a guide RNA (gRNA). Recognition of target DNA for cleavage occurs by hybridization of about 20 bases between the gRNA and its complementary DNA sequence in the genome (figure 17.13). In microbes, the CRISPR locus is the source of the gRNA (see figure 14.27), and the Cas9 nuclease protects the cell from viral attack. Sequences in the CRISPR locus derive primarily from mobile genetic elements (bacteriophage and plasmids), so the Cas9 nuclease in a microbial cell specifically targets invading DNA for destruction. The extreme specificity conferred by the gRNA is the key to genome editing because each 20-base target sequence is almost certainly unique, even in a large eukaryotic genome. In contrast, a restriction enzyme that recognizes a few nucleotides will cut the genome, on average, every few thousand bases. gRNA Cas9 nuclease DNA:RNA hybrid Genomic DNA Cas9 nuclease cuts both DNA strands. The double-strand break is repaired by nonhomologous end joining. The double-strand break is repaired by homologous recombination with donor DNA. Donor DNA Figure 17.13 Genome Editing with Cas9 Nuclease. MICRO INQUIRY How could you assemble the donor DNA molecule for homologous recombination? wil11886_ch17_405-424.indd 421 23/10/18 12:16 pm 422 CHAPTER 17 | Microbial DNA Technologies Cas9 enzymes can be engineered to carry gRNAs with specified nucleotide sequences, thereby programming the recognition sequence for the nuclease. The gRNA directs Cas9 to hybridize with a single, desired site in a genome, making it the most precise mechanism available for targeting and cutting DNA. In eukaryotes, all of which lack a CRISPR/Cas system, the editing process begins by introducing the two components of the mature Cas9 endonuclease, the apoenzyme and the gRNA, to the host cells. These molecules may be added directly, or they may be added as cloned DNA regulated by an inducible promoter. In the latter case, upon induction, the Cas9-gRNA complex assembles and performs its DNA cutting function. Figure 17.13 illustrates how Cas9 recognizes and hydrolyzes a specific DNA sequence. A portion of the gRNA protrudes from the enzyme, available for hybridization. Upon locating its complement, the gRNA induces a conformational shift in the nuclease (protein) portion of Cas9, which then hydrolyzes phosphodiester bonds in both DNA strands. In the simplest case, a point mutation is created as the cell scrambles to repair the damage. Some microbes and all eukaryotes have a nonhomologous end joining (NHEJ) system that can rejoin the two chromosome pieces. But because it’s not a perfect repair, the fused DNA ends often have a deletion or insertion of a few base pairs. The consequence is usually a frameshift mutation in the gene that will result in an inactive protein. A limitation to this method is that the outcome differs in each cell. A precise Cas9 genome edit changes the nucleotides to a predetermined sequence (e.g., a mutated gene could be replaced with the wild-type allele). In this case, a donor sequence must be constructed in vitro using seamless cloning techniques. The donor DNA includes chromosomal regions flanking the gRNA binding site to provide regions of homology for recombination with the chromosome. The donor sequence is introduced to the cell at the same time as the Cas9/ gRNA molecules. Homologous recombination between the broken chromosome and the donor sequence will repair the double-stranded break and at the same time incorporate the DNA carried on the donor molecule into the genome. Selection and screening procedures to identify mutants are not required with Cas9 gene editing, unlike traditional cloning. Cells into which the Cas9-gRNA complex has been introduced can survive and reproduce only if the original target sequence is modified. Unrepaired double-stranded chromosome breaks are lethal. Molecular recombination: joining DNA molecules together (section 16.4) Although the molecular components of Cas9 editing originally derived from the bacterium Streptococcus pyogenes, this technique has been used most frequently in eukaryotic systems. There are several reasons for this. First, many bacteria (~45%) and most archaea (~85%) have homologues of the Cas9 editing system, complicating its use in wil11886_ch17_405-424.indd 422 these organisms. Second, other tools for genome editing already exist for many microbes (see figure 42.5). Third, most bacteria lack a NHEJ repair pathway for repairing the doublestranded break generated by Cas9 nuclease and cannot survive this treatment, but homologous repair can be used efficiently. Despite these challenges, Cas9 editing has been used successfully in some microorganisms that are difficult to manipulate genetically. Researchers have developed other applications for Cas9 nuclease that take advantage of its ability to bind chromosomal DNA in a single genomic location. An altered version of Cas9, termed dead Cas9 (dCas9), retains the DNA localization function conferred by the gRNA, but no longer cuts DNA. Instead, a dCas9-gRNA molecule bound to DNA can serve as a platform for other enzymes. In the simplest example, dCas9-gRNA bound to a promoter acts as a repressor to inhibit expression of target genes. Conversely, to activate transcription, dCas9-gRNA has been fused to the ω subunit of RNA polymerase so it can bind to the promoter of a target gene and recruit RNA polymerase. Genome editing has been demonstrated using dCas9-gRNA fused to additional DNA modifying enzymes. Because the dCas9 variant is used, there is no nuclease activity to break the phosphate-sugar backbone, but the other enzymes directly modify the base, for example, from C to T. The outcome is a precise change to the DNA sequence. Experiments like these have demonstrated the versatility of the dCas9-gRNA module as a homing device to direct a range of effector molecules to a specific chromosomal location. Transcription in bacteria (section 13.5); Regulatory proteins often control transcription initiation (section 14.2) Cas9 gene editing is a relatively new technique that holds much promise, and scientists are actively exploring modifications and improvements. Projects as varied as removing allergens from peanuts to curing genetic diseases in humans have been proposed as examples of what might be achievable. Importantly, Cas9 technology opens the prospect of engineering the human genome in germ line cells (e.g., egg cells or sperm cells), thereby inviting discussions on many fronts to address ethical concerns. Comprehension Check 1. Commercially grown mushrooms are sensitive to handling during harvest and may rapidly discolor, a reaction catalyzed by a single enzyme. Describe a strategy to extend the shelf life of mushrooms using Cas9 genome engineering. 2. A dinucleotide (e.g., GA or CC) appears in DNA with an average frequency of 1 in 16 (42) bases, and a trinucleotide appears with an average frequency of 1 in 64 (43) bases. Calculate how frequently you would expect a 20 bp sequence to occur. Compare this number to the size of the human genome (3 × 109 bp). 23/10/18 12:16 pm Key Concepts 423 Key Concepts 17.1 K ey Discoveries Led to the Development of DNA Cloning Technology Genetic engineering became possible after the discovery of restriction enzymes and reverse transcriptase, and the development of essential methods in nucleic acid chemistry. Restriction enzymes are important because they cut DNA at specific sequences, thereby releasing fragments of DNA that can be cloned or otherwise manipulated (figure 17.3 and table 17.1). There are four types of cloning vectors: plasmids, viruses, cosmids, and artificial chromosomes. Cloning vectors generally have at least three components: an origin of replication, a selectable marker, and a multicloning site (figures 17.6 and 17.7). A common approach to cloning is to digest both vector and DNA to be inserted with the same restriction enzyme or enzymes so that compatible sticky ends are generated. The vector and DNA to be cloned are then incubated in the presence of DNA ligase, which catalyzes the formation of phosphodiester bonds once the DNA fragment inserts into the vector. Once the recombinant plasmid has been introduced into host cells, cells carrying vector must be selected. This is often accomplished by allowing the growth of only antibiotic-resistant cells because the vector bears an antibiotic-resistance gene. Cells that took up vector with inserted DNA must then be distinguished from those that contain only vector. Often a blue-versus-white colony phenotype is used; this is based on the presence or absence, respectively, of a functional lacZ gene (figure 17.4). The bacterium E. coli and the yeast S. cerevisiae are the most common host species. DNA can be introduced into microbes by transformation or electroporation. 17.2 Polymerase Chain Reaction Amplifies Targeted DNA The polymerase chain reaction (PCR) allows small amounts of specific DNA sequences to be amplified, or increased in concentration thousands of times (figure 17.8). PCR consists of multiple cycles of three steps each: DNA denaturation, primer annealing, and DNA synthesis. PCR has numerous applications. It often is used to obtain genes for cloning and in diagnostic and forensic science. Including additional sequences at the 5′ end of an oligonucleotide primer in PCR results in the presence wil11886_ch17_405-424.indd 423 of any desired sequence, for example a restriction site, in the final product (figure 17.9). Gibson assembly is a series of reactions that permits seamless cloning, that is, overlap of specific fragments by enzymatic reactions that do not include restriction enzymes. 17.3 Genomic Libraries: Cloning Genomes in Pieces It is sometimes necessary to find a gene without the knowledge of the gene’s DNA sequence. A genomic library is constructed by cleaving an organism’s genome into many fragments, each of which is cloned into a vector to make a unique recombinant plasmid. Genomic libraries are often screened for the gene of interest by phenotypic rescue (genetic complementation) (figure 17.10). 17.4 Expressing Foreign Genes in Host Cells An expression vector has the necessary features to express in high levels any recombinant gene it carries. If a eukaryotic gene is to be expressed in a bacterium, cDNA is used because it lacks introns; a bacterial ribosome binding site must also be added to the 5′ end of the gene. Purification of recombinant proteins is often accomplished by fusing the coding sequence of a protein to six consecutive histidine residue codons found on some expression vectors. When introduced and expressed in bacteria, the His-tagged protein can be selectively purified (figure 17.11). Fluorescent proteins can be used to study the regulation of gene expression (transcriptional fusions) and protein localization (translational fusions) (figure 17.12). 17.5 Cas9 Nuclease Is a Precise Tool for Genome Editing The Cas9-gRNA nuclease is a precise DNA cutting tool. Because the localization component of the enzyme is an RNA molecule, it can be modified to direct the enzyme to any specified location in a genome. Repair of the double-stranded DNA break catalyzed by Cas9-gRNA allows for the nearby DNA to be altered by substitution or deletion when the desired change is introduced to the cell as a donor sequence. A variant of Cas9 that lacks nuclease activity but retains the guide RNA (dCas9-gRNA) is a convenient means to direct a range of other enzymes to a specific chromosomal target. 23/10/18 12:16 pm 424 CHAPTER 17 | Microbial DNA Technologies Active Learning 1. You are performing PCR to amplify a gene encoding a tRNA from a bacterium that has recently been grown in pure culture. You are expecting a product of 95 base pairs (bp). However, following PCR, you observe three bands on an agarose gel and only one is 95 bp. List at least two possible explanations (excluding experimental error). 2. You have cloned a structural gene required for riboflavin synthesis in E. coli. You find that an E. coli riboflavin auxotroph carrying the cloned gene on a vector makes less riboflavin than the wild-type strain. Why might this be the case? 3. Suppose you transformed E. coli with a plasmid vector carrying a gene that encodes human interferon, but none of the transformants produce interferon. Give as many plausible reasons as possible for this result. 4. You are interested in the activity and regulation of a protease made by the Gram-positive bacterium Geobacillus stearothermophilus. What would be the purpose of constructing each of the following: a Histagged protease, a transcriptional GFP fusion to the protease gene promoter, and a translational GFP fusion to the protease gene coding sequence? 5. The genome sequence of a recently isolated bacterium includes a gene that is predicted to encode a restriction endonuclease. List the steps you could take to express the gene in E. coli and purify the enzyme with a His-tag. How could you determine if the His-tag affects the activity of the enzyme? You’d also like to perform some experiments that involve introducing DNA to this new organism, and although it is naturally transformable, the restriction enzyme appears to be very active in degrading foreign wil11886_ch17_405-424.indd 424 DNA. Describe a strategy to develop a strain of this isolate that has lost the restriction enzyme activity. 6. Many Streptomyces spp. have genomic regions (gene clusters) that are predicted to encode a biosynthetic pathway. As members of this genus often produce antibiotics, there is much interest in exploring these cryptic genes. They are often not expressed under laboratory conditions. Cloning and expressing the genes in another organism have not been generally successful. Propose a CRISPR-based strategy to activate such a gene cluster directly in its native organism. 7. Mesoplasma florum is a wall-less bacterium related to Mycoplasma spp. There is considerable interest in developing a minimal genome host for applications like those described in Techniques & Applications 17.2. M. florum is attractive because it is not pathogenic to humans, it has a rapid growth rate, and has a very small genome: only 793 kb. However, genetic engineering tools do not exist for this organism, and no plasmids have ever been isolated. This prompted a group of scientists from the Université de Sherbrooke to build an M. florum-E. coli shuttle vector. What genetic elements are required for such a construct? Without a known plasmid, where could they get an origin of replication for M. florum? How do you think they could determine the copy number of the shuttle vector in E. coli and in M. florum? Read the original paper: Matteau, D., et al. 2017. Development of plasmids for Mesoplasma florum. Appl. Environ. Microbiol. 83:e02274-16. 23/10/18 12:16 pm