Microbial Genetics PDF
Document Details
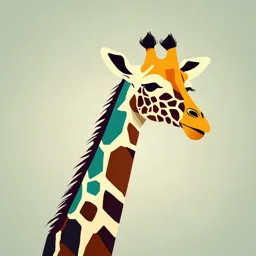
Uploaded by PromptIslamicArt4408
University of Mindanao
Tags
Summary
This document provides an overview of microbial genetics, covering topics such as the structure of DNA and RNA, genotype and phenotype, and the flow of genetic information. It also discusses the role of DNA and RNA in cellular processes.
Full Transcript
BIO 101 - MICROBIOLOGY MICROBIAL GENETICS Genetics is the science of heredity, studying genes and their role in carrying, replicating, and expressing information. The genetic information in a cell is called the genome, which includes chromosomes and plasmids. Chromosomes ar...
BIO 101 - MICROBIOLOGY MICROBIAL GENETICS Genetics is the science of heredity, studying genes and their role in carrying, replicating, and expressing information. The genetic information in a cell is called the genome, which includes chromosomes and plasmids. Chromosomes are structures containing DNA that carry hereditary information, including genes. Genes are segments of DNA (or RNA in some viruses) that code for functional products, primarily proteins but also RNA molecules (ribosomal RNA, transfer RNA, microRNA). DNA is a macromolecule made of repeating nucleotides, each consisting of a nucleobase (adenine, thymine, cytosine, guanine), deoxyribose (sugar), and a phosphate group. DNA forms a double helix with alternating sugar-phosphate backbones and nitrogenous bases held together by hydrogen bonds. Base pairing is specific: adenine pairs with thymine, and cytosine pairs with guanine, making the DNA strands complementary. DNA's structure explains biological information storage: o The linear sequence of nucleobases encodes genetic information, similar to a language with four "letters." o An average gene of 1000 bases can be arranged in 4¹⁰⁰⁰ ways, explaining genetic diversity. The genetic code determines how a nucleotide sequence is translated into the amino acid sequence of proteins. Complementary DNA structure allows for accurate duplication during cell division, ensuring correct inheritance of genetic information. Cellular metabolism focuses on translating genetic messages into proteins. Genes are copied into mRNA, which leads to protein production. Gene expression occurs when the product (e.g., protein) coded by a gene is produced. The flow of genetic information follows the central dogma: DNA → RNA → Protein, a concept proposed by Francis Crick in 1956. Genotype and Phenotype Genotype refers to the genetic makeup of an organism, including all its DNA, which encodes the organism's characteristics. Genotype represents the organism's potential properties but not the properties themselves. Phenotype refers to the actual expressed properties of the organism, such as the ability to perform specific chemical reactions. Phenotype is the manifestation of the genotype. For example, E. coli with the stx gene can produce the Shiga toxin protein. The phenotype of an organism can be viewed as its collection of proteins, as most of a cell's properties derive from protein structures and functions. In microbes, most proteins are either: o Enzymatic: catalyzing specific biochemical reactions. o Structural: participating in functional complexes like membranes or flagella. Phenotypes that rely on structural macromolecules (lipids, polysaccharides) are indirectly dependent on proteins, as these molecules are synthesized, processed, or degraded by enzymes. Describing phenotypes as protein-based is a useful simplification because proteins are central to most cellular properties. DNA and Chromosomes Bacterial Chromosomes: Bacteria typically have a single circular chromosome made of a circular DNA molecule with associated proteins. The chromosome is looped, folded, and attached at one or several points to the plasma membrane. E. coli has a chromosome with about 4.6 million base pairs, approximately 1 mm long—1000 times longer than the cell itself. Despite its length, the chromosome occupies only 10% of the cell’s volume due to supercoiling of the DNA. The genome is not entirely composed of genes. Short Tandem Repeats (STRs), noncoding regions consisting of two- to five-base sequences, occur throughout genomes, including that of E. coli. STRs are used in DNA fingerprinting. Complete base sequences of chromosomes can now be determined, allowing the identification of open reading frames (ORFs)—DNA regions likely to encode proteins, located between start and stop codons. Genomics refers to the sequencing and molecular characterization of genomes. Genomics is used in various applications, such as tracking viruses like Zika. 1|P a g e BIO 101 - MICROBIOLOGY THE FLOW OF GENETIC INFORMATION DNA Replication Vertical Gene Transfer: DNA replication enables the transfer of genetic information from one generation to the next, where offspring cells receive chromosomes identical to the parent’s. DNA Replication Process: o One "parental" double-stranded DNA molecule is converted into two identical offspring molecules. o DNA's complementary base structure (adenine pairs with thymine, guanine pairs with cytosine) allows one strand to act as a template for the other. o Replication begins with the relaxation of supercoiling by topoisomerase or gyrase, followed by unwinding of DNA strands by helicase. o Free nucleotides pair with the exposed bases on the parental DNA strands. o DNA polymerase adds nucleotides to the growing DNA strand. o The point where replication occurs is called the replication fork. o Semiconservative replication: Each new DNA molecule consists of one original strand and one newly synthesized strand. DNA Structure: o DNA strands are antiparallel (oriented in opposite directions). o The 3′ end has a hydroxyl group, while the 5′ end has a phosphate group. o DNA polymerases add new nucleotides only to the 3′ end. Leading and Lagging Strands: o The leading strand is synthesized continuously in the 5′ → 3′ direction. o The lagging strand is synthesized discontinuously in Okazaki fragments, which are later joined to form a continuous strand. RNA and Protein Synthesis Overview: Genetic information in DNA is used to make proteins that control cellular activities. Two main processes: transcription (DNA to RNA) and translation (RNA to protein). In prokaryotes, transcription and translation occur in the cytoplasm. Transcription in Prokaryotes: Transcription: synthesis of RNA from a DNA template using RNA polymerase. Three types of RNA involved in protein synthesis: ribosomal RNA (rRNA), transfer RNA (tRNA), and messenger RNA (mRNA). mRNA carries coded genetic information from DNA to ribosomes for protein synthesis. 2|P a g e BIO 101 - MICROBIOLOGY Complementary base pairing during transcription: guanine (G) pairs with cytosine (C), thymine (T) with adenine (A), and adenine with uracil (U) in RNA. Transcription starts at a promoter and ends at a terminator sequence on the DNA. Translation: Translation: decoding of mRNA into a sequence of amino acids (protein synthesis). The genetic code is based on codons (groups of three nucleotides on mRNA), each coding for a specific amino acid. 64 possible codons, 61 of which are sense codons (coding for amino acids), and 3 are nonsense (stop) codons (UAA, UAG, UGA). Start codon (AUG) initiates protein synthesis, coding for methionine (formylmethionine in bacteria). Each tRNA carries an anticodon that complements an mRNA codon and carries the corresponding amino acid. Ribosomes facilitate the assembly of amino acids into a polypeptide chain through peptide bonds. Process of Translation: Translation begins when a ribosome assembles on the mRNA at the start codon. The ribosome moves along the mRNA in the 5′ to 3′ direction, synthesizing protein by forming peptide bonds between amino acids. Translation ends when a stop codon is reached, releasing the newly synthesized protein and the ribosome subunits. Multiple ribosomes can translate a single mRNA molecule simultaneously in prokaryotes. In prokaryotes, translation can begin even before transcription is fully completed, as both processes occur in the cytoplasm. Transcription in Eukaryotes: Occurs in the nucleus of eukaryotic cells, and the mRNA must be fully synthesized and processed before leaving the nucleus. mRNA processing: Before translation begins, the RNA transcript undergoes modifications, including: o Splicing: Introns (noncoding regions) are removed, and exons (coding regions) are spliced together. o snRNPs (small nuclear ribonucleoproteins): These particles are responsible for removing introns and splicing exons in the RNA transcript. o In some cases, introns can act as ribozymes, catalyzing their own removal. Genes and Expression: Genes are sequences of nucleotides in DNA that carry biological information. Gene expression: Involves two main processes: o Transcription: DNA is transcribed into a temporary mRNA molecule. o Translation: mRNA directs the assembly of a protein by specifying the sequence of amino acids. 3|P a g e BIO 101 - MICROBIOLOGY ▪ The ribosome binds to mRNA, tRNAs deliver amino acids based on the codon sequence, and the ribosome assembles these into a polypeptide chain (protein). The Regulation of Bacterial Gene Expression Interdependence of Genetic and Metabolic Machinery: Bacterial cells carry out numerous metabolic reactions, all of which are catalyzed by enzymes synthesized through transcription and translation. Feedback inhibition is a mechanism that stops cells from performing unnecessary chemical reactions by inhibiting enzymes that have already been synthesized. Energy Efficiency in Protein Synthesis: Since protein synthesis is energy-intensive, cells regulate gene expression to only produce proteins when necessary. Constitutive genes: About 60–80% of genes are not regulated and are constantly active, producing products at a fixed rate. These genes typically code for essential enzymes needed for basic life processes, such as those involved in glycolysis. Regulation of Gene Expression: Other genes are regulated, ensuring enzymes are only produced when needed. An example is the Trypanosoma parasite, which causes African sleeping sickness. It has hundreds of genes for surface glycoproteins, but each cell expresses only one glycoprotein gene at a time. This enables the parasite to evade the host's immune response by changing surface proteins, allowing it to survive. Pre-transcriptional Control: o Genetic mechanisms like repression and induction regulate the transcription of mRNA, which controls the synthesis of enzymes. These mechanisms affect the amount of enzymes produced, not their activity. Operon Model of Gene Expression: o Proposed by François Jacob and Jacques Monod in the 1960s, the operon model explains gene expression through induction and repression. o An operon is a group of genes that are transcribed together and controlled by a single promoter. It consists of structural genes (which code for proteins), a promoter (where RNA polymerase initiates transcription), and an operator (which acts as a regulatory "switch"). Inducible Operons: o Example: lac operon in E. coli. ▪ It regulates the production of enzymes needed for lactose metabolism. ▪ Structural genes for β-galactosidase, lac permease, and transacetylase are transcribed together. ▪ When lactose is absent, a repressor protein (encoded by the I gene) binds to the operator region, blocking transcription. ▪ When lactose is present, it binds to the repressor, preventing the repressor from attaching to the operator, allowing transcription of the lactose-digesting enzymes. Repressible Operons: o Example: tryptophan operon. ▪ In this system, the structural genes are normally transcribed until they are turned off. ▪ When tryptophan is abundant, it acts as a corepressor, binding to the repressor protein, which then attaches to the operator to stop transcription, halting tryptophan production. These mechanisms allow bacteria to efficiently regulate enzyme production in response to environmental changes. Positive Regulation: Regulation of the lac operon is influenced not only by lactose but also by the level of glucose. This involves the cyclic AMP (cAMP) molecule, derived from ATP, which acts as a cellular alarm signal. Glucose is the preferred carbon source for cells, and enzymes for glucose metabolism are constitutive (produced constantly). When glucose is no longer available, cAMP accumulates in the cell and binds to the catabolic activator protein (CAP) at its allosteric site. CAP bound with cAMP then attaches to the lac promoter, facilitating the binding of RNA polymerase and initiating transcription of the lac operon. Transcription of the lac operon requires two conditions: 1. The presence of lactose (to inactivate the repressor). 2. The absence of glucose (to increase cAMP and activate CAP). 4|P a g e BIO 101 - MICROBIOLOGY Catabolite repression (also known as the glucose effect) describes the inhibition of the metabolism of alternative sugars (like lactose) when glucose is available. In the presence of glucose, cAMP levels remain low, preventing CAP from binding, which in turn blocks transcription of the lac operon. Epigenetic Control: Both eukaryotic and bacterial cells can regulate genes by methylation of certain nucleotides, which involves adding a methyl group (-CH3) to DNA. Methylation turns genes off, and these "off" genes are passed to offspring cells. However, unlike mutations, this change is not permanent and can be reversed, allowing the gene to be turned on in later generations. This process is known as epigenetic inheritance. Epigenetic changes can influence bacterial behavior, such as in biofilms, where bacteria may act differently due to gene regulation through methylation. Post-transcriptional Control: Regulatory mechanisms can inhibit protein synthesis after transcription has occurred. Riboswitches: o A specific part of an mRNA molecule that can bind to a substrate, altering its structure. o The structural change can either initiate or stop translation. o Both eukaryotes and prokaryotes utilize riboswitches for gene expression control. MicroRNAs (miRNAs): o Short, single-stranded RNA molecules, approximately 22 nucleotides long, that inhibit protein production in eukaryotic cells. o During development, miRNAs allow different cells (e.g., heart cells vs. skin cells) to produce distinct proteins despite having the same genes. o Each cell type produces specific miRNAs that direct which proteins are synthesized. Mechanism of miRNAs: o miRNAs base-pair with complementary mRNA, forming a double-stranded RNA complex. o This complex is enzymatically degraded, preventing the translation of the associated mRNA-encoded protein. Small Interfering RNAs (siRNAs): o Similar to miRNAs, siRNAs also play a role in post-transcriptional regulation, particularly in eukaryotes, and will be discussed in more detail later. CHANGES IN GENETIC MATERIAL DNA Changes: Bacterial DNA can undergo changes through: o Mutations: Spontaneous alterations in the DNA sequence. o Horizontal Gene Transfer: The acquisition of DNA from other organisms, leading to genetic variation. Genetic Variations: Changes in DNA lead to genetic variations, impacting microbial functions such as: o Biofilm Formation: The ability of bacteria to adhere to surfaces and form structured communities. o Pathogenicity: The potential of bacteria to cause disease. o Antibiotic Resistance: The ability of bacteria to survive and thrive despite the presence of antibiotics. Survival and Reproduction: Bacteria with new genotypes can be favored in both natural and human-influenced environments, promoting diversity among microorganisms. Natural Selection: The process by which the survival and reproduction of individuals with advantageous traits lead to the prevalence of those traits in a population over time, ensuring the survival of new genotypes. Mutation Definition: A mutation is a permanent alteration in the base sequence of DNA, which can lead to changes in the encoded product, such as proteins. Impact of Mutations: o Disadvantageous Effects: Some mutations may result in nonfunctional or less effective enzymes, potentially leading to loss of essential traits for the cell. o Beneficial Effects: In some cases, mutations may enhance enzyme activity, providing advantages to the cell. Types of Mutations 5|P a g e BIO 101 - MICROBIOLOGY 1. Silent Mutations: o Definition: Changes in the DNA base sequence that do not affect the activity of the encoded product. o Mechanism: Often occur due to substitution of one nucleotide for another, especially in the third position of the mRNA codon, resulting in the same amino acid being coded due to the genetic code's degeneracy. 2. Base Substitution (Point Mutation): o Definition: A single base in the DNA sequence is replaced by a different base. o Result: This substitution leads to a potentially altered mRNA and a change in the resulting protein. o Missense Mutation: When the substitution results in an amino acid change in the protein (e.g., sickle cell disease caused by a single nucleotide change leading to a change in hemoglobin structure). 3. Nonsense Mutation: o Definition: A base substitution that creates a premature stop codon in the mRNA. o Effect: Prevents the synthesis of a complete functional protein, resulting in only a fragment being produced. 4. Frameshift Mutations: o Definition: Caused by the deletion or insertion of one or a few nucleotide pairs, shifting the “translational reading frame.” o Effect: Alters the downstream amino acid sequence, often resulting in an entirely different and usually nonfunctional protein. It may lead to a premature stop codon during translation. Causes of Mutations Spontaneous Mutations: Base substitutions and frameshift mutations can occur naturally due to mistakes during DNA replication, without any external mutagens. Mutagens Definition: Mutagens are agents that induce mutations in DNA, either directly or indirectly, through environmental factors like chemicals and radiation. Types of Chemical Mutagens 1. Nitrous Acid: o Mechanism: Converts the base adenine into a modified form that pairs with cytosine instead of thymine. o Effect: When DNA replicates, adenine in the parent DNA changes from an AT base pair to a GC base pair in daughter DNA, leading to permanent changes in the genetic code. 2. Nucleoside Analogs: o Description: Structurally similar to normal nitrogenous bases but with altered base-pairing properties (e.g., 2- aminopurine, 5-bromouracil). o Mechanism: Incorporated into DNA during replication, leading to mistakes in base pairing, which result in base- pair substitutions in progeny cells. o Applications: Used in antiviral and antitumor drugs like AZT (azidothymidine) for HIV treatment. 3. Frameshift Mutagens: o Examples: Benzopyrene (found in smoke and soot) and aflatoxin (produced by Aspergillus flavus, a mold). o Mechanism: Cause small deletions or insertions in DNA by slipping between base pairs of the double helix, creating gaps or bulges. o Effect: During DNA replication, these gaps may lead to the insertion or deletion of base pairs, resulting in frameshift mutations. o Health Implications: Often potent carcinogens, which can lead to cancer due to their mutagenic effects. Radiation as a Mutagen Types of Radiation: o X-rays and Gamma Rays: ▪ Potent mutagens that ionize atoms and molecules. ▪ Cause electrons to be ejected from their shells, creating ions and free radicals. ▪ Result in oxidation of DNA bases, leading to replication errors and mutations. ▪ Can break covalent bonds in the DNA sugar-phosphate backbone, causing physical chromosome breaks. o Ultraviolet (UV) Light: ▪ Nonionizing radiation component of sunlight. ▪ Most mutagenic UV wavelength (260 nm) is absorbed by the ozone layer. ▪ Causes covalent bonds to form between adjacent pyrimidine bases, leading to thymine dimers. ▪ Thymine dimers hinder proper transcription and replication, potentially causing cell damage or death if not repaired. Repair Mechanisms: 6|P a g e BIO 101 - MICROBIOLOGY o Photolyases (Light-Repair Enzymes): ▪ Utilize visible light energy to separate thymine dimers back into individual thymines. o Nucleotide Excision Repair: ▪ Repairs mutations caused by UV and other factors. ▪ Involves enzymes that cut out incorrect bases and fill the gap with newly synthesized complementary DNA. o Methylases: ▪ Enzymes that add a methyl group to selected bases shortly after DNA strand synthesis. ▪ A repair endonuclease cuts the non-methylated strand, facilitating accurate repair. The Frequency of Mutation Mutation Rate: o Defined as the probability that a gene will mutate during cell division. o Typically expressed as a power of ten with a negative exponent due to the rarity of mutations. o Example: A mutation rate of 1/1,000,000 is expressed as 10−6 Spontaneous Mutation: o Occurs at a very low rate, approximately once in 109 replicated base pairs. o Average gene length is about 103 base pairs, leading to a spontaneous mutation rate of roughly one in 106 replicated genes. Random Occurrence: o Mutations occur randomly along chromosomes. o Random mutations at low frequencies are crucial for species adaptation and evolution, as they generate genetic diversity. o In large bacterial populations (e.g., > 107 cells), a few new mutant cells are produced each generation. Impact of Mutations: o Most mutations are harmful or neutral; only a few are beneficial. o Example: A mutation conferring antibiotic resistance is advantageous in environments with antibiotic exposure. o Beneficial mutations increase survival and reproduction, leading to evolutionary changes over time. Role of Mutagens: o Mutagens increase the spontaneous mutation rate by a factor of 10 to 1,000. o In the presence of mutagens, the mutation rate can rise from 10 −6 to between 10−5 and 10−3 per replicated gene. o Mutagens are used in research and commercial applications to promote the production of mutant cells for studying genetic properties and other purposes. Identifying Mutants Detection of Mutants: o Mutants can be identified by selecting or testing for altered phenotypes. o Mutant cells are rare compared to other cells in the population, making detection challenging. Use of Bacteria in Experiments: o Bacteria are preferred for experiments due to their rapid reproduction (over 10910^9109 organisms/mL). o Bacteria typically have only one copy of each gene, ensuring that the effects of a mutated gene are not masked by normal gene versions. Positive (Direct) Selection: o This method detects mutant cells by rejecting unmutated parent cells. o Example: To find penicillin-resistant bacteria, cells are plated on a medium containing penicillin. ▪ Mutant cells (resistant) will grow and form colonies, while normal (sensitive) cells cannot. Negative (Indirect) Selection: o This method selects cells that cannot perform specific functions using replica plating. o Example: To identify bacteria that cannot synthesize histidine: ▪ Cells are inoculated on a master plate with histidine. ▪ After incubation, a sterile pad (e.g., velvet) is pressed onto the master plate to transfer cells. ▪ The pad is then pressed onto two sterile plates: one with histidine and one without. ▪ Mutant colonies that can grow on the master plate but cannot synthesize histidine will not grow on the plate without histidine. Screening for Specific Mutants: o Due to the rarity of mutants, multiple plates must be screened to isolate specific mutants. Replica Plating: 7|P a g e BIO 101 - MICROBIOLOGY o An effective technique for isolating mutants that require new growth factors. o Mutants lacking enzymes for synthesizing certain nutrients are called auxotrophs. o Auxotrophs require specific growth factors (e.g., amino acids) absent in the parent strain. Identifying Chemical Carcinogens Definition of Carcinogens: o Carcinogens are substances that cause cancer in animals, including humans. o Many known mutagens have been identified as carcinogens. Sources of Carcinogens: o Chemicals found in the environment, workplace, and diet are implicated in causing cancer in humans. Challenges in Testing: o Traditional animal testing for carcinogens is time-consuming and expensive. Ames Test: o A faster, less expensive preliminary screening method using bacteria as indicators of carcinogenic potential. o Based on the observation that exposure of mutant bacteria to mutagens may cause reversions, which are new mutations that restore the original phenotype. Procedure: o The test specifically uses histidine auxotrophs of Salmonella (his- cells) that cannot synthesize histidine. o The his- bacteria are treated with the potential mutagen and incubated in the presence of rat liver extract, which contains activation enzymes. o If the substance is mutagenic, it will cause a higher rate of reversion to histidine-synthesizing cells (his+) compared to the spontaneous reversion rate. Evaluation: o The number of observed revertants indicates the degree of mutagenicity and potential carcinogenicity of the substance. o The Ames test can be performed in liquid media using a pH indicator in a 96-well plate, allowing for qualitative testing of multiple potential mutagens or different concentrations. Results and Applications: o Bacterial growth is indicated by a color change in the pH indicator. o The Ames test is routinely used to evaluate new chemicals and pollutants in air and water. o Approximately 90% of substances identified as mutagenic in the Ames test have also been shown to be carcinogenic in animals. o More mutagenic substances generally correlate with higher carcinogenic potential. GENETIC TRANSFER AND RECOMBINATION Genetic Recombination Definition: o Genetic recombination involves the exchange of genes between two DNA molecules, forming new gene combinations on a chromosome. Mechanism: o Donor DNA can be picked up by a cell, leading to a process called crossing over. o Crossing over shuffles genes on the chromosome, incorporating portions of donor DNA. Eukaryotic Recombination: o In eukaryotes, recombination occurs during the sexual reproductive cycle. o Crossing over typically takes place during the formation of reproductive cells, resulting in recombinant DNA. Bacterial Recombination: o Bacteria can undergo genetic recombination through various mechanisms, which will be detailed later. o Like mutations, recombination enhances genetic diversity in populations, contributing to evolutionary variation. Benefits of Recombination: o In highly evolved organisms, recombination is generally more beneficial than mutation, as it is less likely to disrupt gene function and can create advantageous gene combinations. Example: Salmonella: o Salmonella can produce two different flagellar proteins. o The production of a specific flagellar protein is determined by random recombination events within the chromosomal DNA, allowing the bacteria to evade immune responses. Gene Transfer Types: o Vertical Gene Transfer: 8|P a g e BIO 101 - MICROBIOLOGY ▪ Genes are passed from an organism to its offspring (common in plants and animals). o Horizontal Gene Transfer: ▪ Bacteria can transfer genes not only to their offspring but also laterally to other microbes of the same generation. ▪ This transfer is important for the spread of antibiotic resistance among bacteria. Horizontal Gene Transfer Mechanism: o Involves a donor cell that transfers a portion of its DNA to a recipient cell. o The transferred DNA may be integrated into the recipient's DNA, while the remainder is degraded by cellular enzymes. o The recipient cell that incorporates donor DNA is referred to as a recombinant. Frequency of Gene Transfer: o Genetic transfer between bacteria is infrequent, occurring in about 1% or less of an entire population. Plasmids and Transposons Overview Plasmids and transposons are genetic elements outside chromosomes, occurring in both prokaryotic and eukaryotic organisms, with a focus on their role in prokaryotes. They are known as mobile genetic elements due to their ability to move between chromosomes and cells. Plasmids Definition: Self-replicating, circular pieces of DNA that are about 1-5% the size of a bacterial chromosome. Occurrence: Primarily found in bacteria; some eukaryotic microorganisms, such as Saccharomyces cerevisiae, also contain plasmids. Types of Plasmids F Factor: o A conjugative plasmid that carries genes for sex pili and facilitates the transfer of the plasmid to another cell. Dissimilation Plasmids: o Code for enzymes that enable the catabolism of unusual sugars and hydrocarbons. o Example: Certain Pseudomonas species can use substances like toluene, camphor, and petroleum as carbon and energy sources due to plasmid-encoded enzymes. o These capabilities allow survival in diverse and challenging environments, with potential applications in environmental waste cleanup. Pathogenicity Plasmids: o Enhance bacterial pathogenicity by coding for proteins involved in toxin production and bacterial attachment. o Example: Plasmids in E. coli strains causing infant diarrhea and traveler’s diarrhea enable toxin production and attachment to intestinal cells. Bacteriocin-Encoding Plasmids: o Contain genes for bacteriocins, which are toxic proteins that kill other bacteria, serving as markers for bacterial identification in clinical labs. Resistance Factors (R Factors): o Important medical plasmids discovered during dysentery epidemics in Japan in the late 1950s, conferring antibiotic resistance. o R factors consist of two groups of genes: ▪ Resistance Transfer Factor (RTF): Involved in plasmid replication and conjugation. ▪ R-determinant: Contains resistance genes that code for enzymes inactivating certain drugs or toxins. Implications of R Factors R factors contribute to the growing populations of antibiotic-resistant bacteria due to selective survival from antibiotic use in medicine and agriculture. Resistance can be transferred between bacterial species, complicating treatment options for infectious diseases. Non-conjugative plasmids can also be transferred through integration with conjugative plasmids or transformation after release from dead cells. Genetic Engineering Plasmids are valuable tools in genetic engineering, facilitating the manipulation of genetic material in various applications. Transposons Definition 9|P a g e BIO 101 - MICROBIOLOGY Transposons are small segments of DNA, ranging from 700 to 40,000 base pairs, that can move or "transpose" from one region of a DNA molecule to another. Discovery Discovered by American geneticist Barbara McClintock in corn during the 1950s, transposons are found in all organisms and have been extensively studied in microorganisms. Movement Transposons can move within the same chromosome or to another chromosome or plasmid. Their frequent movement can potentially disrupt cellular functions by inserting themselves into genes and inactivating them. Frequency of Transposition Transposition occurs relatively rarely, with a frequency comparable to the spontaneous mutation rate in bacteria, estimated at 10⁻⁵ to 10⁻⁷ per generation. Structure Simple Transposons (Insertion Sequences - IS): o Contain a gene coding for the enzyme transposase, which catalyzes the cutting and resealing of DNA during transposition. o Include recognition sites, which are short inverted repeat sequences that the transposase enzyme recognizes as recombination sites. Complex Transposons: o Carry additional genes unrelated to the transposition process, such as those for enterotoxins or antibiotic resistance. o R factors, which are resistance plasmids, often consist of collections of transposons. Functional Importance Transposons can contain various genes, providing a natural mechanism for gene movement between chromosomes. They can also be transferred between cells via plasmids or viruses, facilitating horizontal gene transfer between organisms or even species. Example: The transfer of vancomycin resistance from Enterococcus faecalis to Staphylococcus aureus via the transposon Tn1546. Evolutionary Role Transposons serve as powerful mediators of evolution by facilitating genetic diversity and adaptation in organisms. Their ability to carry genes across different species highlights their significance in the development of antibiotic resistance and other traits. Transformation in Bacteria Definition: Transformation is the process where genes are transferred from one bacterium to another as "naked" DNA in solution. Historical Background: o First demonstrated over 70 years ago; pivotal in concluding that DNA is the genetic material. o Initial experiment conducted by Frederick Griffith in 1928 using Streptococcus pneumoniae strains. Griffith’s Experiment: o Used two strains: ▪ Virulent strain with a polysaccharide capsule (causes pneumonia). ▪ Avirulent strain lacking the capsule (does not cause disease). o Observations: ▪ Injections of live encapsulated bacteria killed mice. ▪ Injections of live nonencapsulated or dead encapsulated bacteria did not kill mice. ▪ Mixing dead encapsulated bacteria with live nonencapsulated bacteria resulted in mouse deaths, with living encapsulated bacteria found in their blood. Conclusion from Griffith’s Work: o Genetic material from dead encapsulated bacteria transformed live nonencapsulated bacteria into encapsulated, virulent strains. Further Research: o Subsequent experiments showed transformation could occur without using mice: ▪ Dead encapsulated bacteria were added to a broth of live nonencapsulated bacteria, resulting in the culture containing living encapsulated bacteria. Avery’s Experiment: o Oswald T. Avery and associates determined in 1944 that DNA was the transforming component responsible for the virulence of S. pneumoniae. 10 | P a g e BIO 101 - MICROBIOLOGY Mechanism of Transformation: o In nature, some bacteria release their DNA into the environment post-death and lysis. o Other bacteria can take up this DNA, integrating it into their chromosomes via recombination. o The protein RecA facilitates the exchange of strands between the recipient and donor DNA. Recombinant Cells: o A recipient cell with integrated donor DNA is termed a hybrid or recombinant cell, and all descendants will be identical. Natural Occurrence: o Transformation occurs naturally in a few bacterial genera, including: ▪ Bacillus, Haemophilus, Neisseria, Acinetobacter, certain strains of Streptococcus, and Staphylococcus. Competence: o A recipient cell is considered competent when it can take up donor DNA, resulting from alterations in the cell wall that increase permeability to large DNA molecules. Conjugation in Bacteria Definition: Conjugation is a mechanism of genetic material transfer between bacteria, mediated by a conjugative plasmid. Key Differences from Transformation: o Requires direct cell-to-cell contact. o Typically involves cells of opposite mating types (donor cells carry the plasmid; recipient cells usually do not). Mechanism: o In gram-negative bacteria, the plasmid encodes genes for the synthesis of sex pili, which facilitate contact between donor and recipient cells. o In gram-positive bacteria, sticky surface molecules promote direct contact. o During conjugation, the plasmid is replicated and a single-stranded copy of the plasmid DNA is transferred to the recipient, where a complementary strand is synthesized. Example with E. coli: o The F factor (fertility factor) is the first plasmid observed to be transferred during conjugation. o Donor cells with F factors (F+ cells) transfer the plasmid to recipient cells (F- cells), converting them into F+ cells. o Some F+ cells integrate the F factor into their chromosome, becoming Hfr cells (high frequency of recombination). o When an Hfr cell conjugates with an F- cell, it transfers a parental strand of its chromosome, beginning replication at the integrated F factor. o Typically, the chromosome breaks before complete transfer; donor DNA can recombine with the recipient’s DNA. Any unintegrated donor DNA is degraded. o An F- cell remains F- because it did not receive a complete F factor during conjugation, even after acquiring new chromosomal genes. Gene Mapping: o Conjugation is utilized for mapping gene locations on bacterial chromosomes. o For example, genes for threonine (thr) and leucine (leu) can be ordered based on acquisition during conjugation experiments. o If an F- cell acquires the ability to synthesize threonine after 1 minute of conjugation, the thr gene is located early on the chromosome. o If it acquires both thr and leu after 2 minutes, the order of genes is determined as thr, leu. Transduction in Bacteria Definition of Transduction: o A mechanism of genetic transfer between bacteria involving a virus (bacteriophage or phage) that infects bacteria. Process Overview: o Bacterial DNA is transferred from a donor cell to a recipient cell via a bacteriophage. Phage Life Cycle: o In the case of generalized transduction (specifically using E. coli as an example): ▪ Phage DNA and proteins are synthesized by the host bacterial cell. ▪ Phage DNA is typically packaged within the phage protein coat. ▪ There is potential for bacterial DNA, plasmid DNA, or DNA from another virus to be packaged instead. Generalized vs. Specialized Transduction: o Generalized Transduction: 11 | P a g e BIO 101 - MICROBIOLOGY ▪ All genes within a bacterium infected by a transducing phage have an equal chance of being packaged and transferred. o Specialized Transduction: ▪ Only specific bacterial genes are transferred. ▪ Example: Certain phages code for toxins produced by their bacterial hosts: ▪ Diphtheria toxin for Corynebacterium diphtheriae ▪ Erythrogenic toxin for Streptococcus pyogenes ▪ Shiga toxin for E. coli O157 Genes and Evolution Gene Regulation: o Gene activity is controlled by the cell’s internal regulatory mechanisms. Gene Alterations: o Genes can be changed or rearranged through processes such as: ▪ Mutation ▪ Transposition ▪ Recombination Diversity and Evolution: o These processes create genetic diversity among cell descendants. o Diversity serves as the raw material for evolution. Natural Selection: o Natural selection acts on diverse populations to promote the survival of organisms best suited to their environment. o It is the driving force behind evolutionary change. Microorganisms and Evolution: o The various types of microorganisms present today result from a long evolutionary history. o Microorganisms have evolved through genetic changes and adaptations to different habitats. 12 | P a g e