Microbial Biotechnology Lec. 5 Recombinant DNA Technology PDF
Document Details
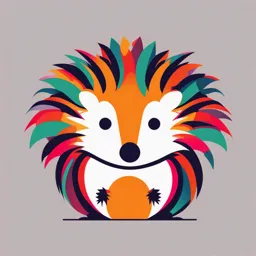
Uploaded by WellRoundedArtePovera
Philadelphia University
Dr. Marwan Abu-Halaweh
Tags
Summary
This document provides an overview of microbial biotechnology, focusing on recombinant DNA technology and the mechanisms of genetic control. It covers concepts like promoters, repressors, activators, and operons, using diagrams to illustrate the processes. Note that the document is a lecture, not a past paper.
Full Transcript
Microbial Biotechnology Lec. 5 Recombinant DNA Technology Dr. Marwan Abu-Halaweh Office 1016 Email:[email protected] Philadelphia University Promoter Reminder The promoter sequence) is recognised by RNA polymerase holoenzyme. The prom...
Microbial Biotechnology Lec. 5 Recombinant DNA Technology Dr. Marwan Abu-Halaweh Office 1016 Email:[email protected] Philadelphia University Promoter Reminder The promoter sequence) is recognised by RNA polymerase holoenzyme. The promotor lies adjacent to a segment of DNA called the activator-binding site. RNA polymerase is composed of 5 polypeptides (when it binds to promoters): ά 2β β σ. The σ factor is the protein which recognises the specific promoter sequences. The most important nucleotides are centered at –35 and – 10 relative to the transcription start-site, for promoters recognised by the classical "vegetative" E. coli sigma factor, s 70: 5'- TTGACA--17 bp spacing—TATAAT-5 to 9 bp- START Codon "-35" "-10" +1 Genetic Control Genetic control of enzyme activity refers to controlling transcription of the mRNA needed for an enzyme's synthesis. In prokaryotic cells, this involves the induction or repression of enzyme synthesis by regulatory proteins that can bind to DNA and either block or enhance the function of RNA polymerase, the enzyme required for transcription. The regulatory proteins are part of either an operon or a regulon. An operon is a set of genes transcribed as a polycistronic message that is collectively controlled by a regulatory protein. A regulon is a set of related genes controlled by the same regulatory protein but transcribed as monocistronic units. Regulatory proteins may function either as repressors or activators. Genetic Control genetic control are divided into three groups based on the functional activity of these groups: 1. Repressors: Regulatory proteins that block transcription of mRNA 2. Activators: Regulatory proteins that promote transcription of mRNA. 3. Translational control (antisense RNA: A strand of RNA complementary to a mRNA coding for some polypeptide or protein). Operon structure A. Monocistronic Promoter, ribosome binding site, start codon, structural gene, stop codon, terminator. B. Polycistronic Promoter, ribosome binding site, start codon, structural gene, stop codon, ribosome binding site, start codon, structural gene, stop codon, terminator. (See Trp operon, below) – a. If polycistronic gene products tend to be involved in the same pathway. – b. Means of achieving coordinate regulation (only one transcriptional unit). 1. Repressors Repressors are regulatory proteins that block transcription of mRNA. They do this by binding to a portion of DNA called the operator that lies downstream of a promoter. The binding of the regulatory protein to the operator prevents RNA polymerase from passing the operator and transcribing the coding sequence for the enzymes. This is called negative control. Repressors are allosteric proteins that have a binding site for a specific molecule. Inactive Repressors (trp Corepressor) The tryptophan is able to bind to a site on the allosteric repressor protein, changing its shape and enabling it to interact with the operator region. Once the repressor binds to the operator, RNA polymerase is unable to get beyond the operator and transcribe the genes for tryptophan biosynthesis. Therefore, when sufficient tryptophan is present, transcription of the enzymes that allows for its biosynthesis are turned off (see Fig. 3) and Fig. 4). B. Active Repressors Other repressors are synthesized in a form that readily binds to the operator and blocks transcription. However, the binding of a molecule called an inducer alters the shape of the regulatory protein in a way that now blocks its binding to the operator and thus permits transcription. An example of this is the lac operon that encodes for the three enzymes needed for the degradation of lactose by E. coli. E.coli will only sunthesize the three enzymes it requires to utilize lactose if that sugar is present in the surrounding environment. In this case, lactose functions as an inducer. In the absence of lactose, the repressor protein binds to the operator and RNA polymerase is unable to get beyond the operator and transcribe the genes for utilization of lactose and the three enzymes for degradation of lactose are not synthesized (see Fig. 5) and Fig. 6). Active Repressors (Lactose Inducer) When lactose, the inducer, is present, it binds to the allosteric repressor protein and causes it to change shape in such a way that it is no longer able to bind to the operator. Now RNA polymerase can transcribe the three genes required for the degradation of lactose and the bacterium is able to synthesize the enzymes needed for its utilization (see Fig. 7 and Fig. 8). An Inducible Operon in the Absence of an Inducer (The Lactose Operon) -The regulator gene codes for an active repressor protein. -The repressor protein then binds to the operator region of the operon. -With the active repressor protein bound to the operator region, RNA polymerase (the enzyme responsible for the transcription of genes) is unable to bind to the promoter region of the operon. -If RNA polymerase does not bind to the promoter region, the three enzyme genes (Z, Y, and A) are not transcribed into mRNA. -Without the transcription of the three enzyme genes, the three enzymes needed for the utilization of the sugar lactose by the bacterium are not synthesized. An Inducible Operon in the Presence of an Inducer (The Lactose Operon) - Lactose, the inducer molecule binds to the active repressor protein. - - The binding of the inducer alters the shape of the allosteric repressor causing it to become inactivated. - The inactivated repressor protein is then unable to bind to the operator region of the operon. - Since the inactive repressor protein is unable to bind to the operator region, RNA polymerase (the enzyme responsible for the transcription of genes) is now able to bind to the promoter region of the operon. - RNA polymerase is now able to transcribe the three enzyme genes (Z, Y, and A) into mRNA. - With the transcription of these genes, the three enzymes needed for the bacterium to utilize the sugar lactose are now synthesized. (The Z gene codes for beta-galactosidase, an enzyme that breaks down lactose into glucose and galactose. The Y gene codes for permease, an enzyme which transports lactose into the bacterium. The A gene codes for transacetylase, an enzyme which is thought to aid in the release of galactosides.) 2. Activators Activators are regulatory proteins that promote transcription of mRNA. Activators control genes that have a promotor to which RNA polymerase cannot bind. The activator is an allosteric protein synthesized in a form that cannot normally bind to the activator-binding site. As a result, RNA polymerase is unable to bind to the promoter and transcribe the genes (see Fig. 9). However, binding of a molecule called an inducer to the activator alters the shape of the activator in a way that now allows it to bind to the activator-binding site. The binding of the activator to the activator-binding site, enables RNA polymerase to bind to the promotor and initiate transcription (see Fig. 10 and Fig. 11). This is called positive control. Fig. 9: An Activator Protein in the Absence of an Inducer Fig. 10: An Activator Protein in the Presence of an Inducer, Step-1 Fig. 11: An Activator Protein in the Presence of an Inducer, Step-1 An Activator Protein in the Absence of an Inducer An Activator Protein in the Presence of an Inducer 3. Antisense RNA Bacteria also use translational control of enzyme synthesis. In this case, the bacteria produce antisense RNA (def) that is complementary to the mRNA coding for the enzyme. When the antisense RNA binds to the mRNA by complementary base pairing, the mRNA cannot be translated into protein and the enzyme is not made (see Fig. 12). Fig. 12: Antisense RNA During translational control of enzyme synthesis, bacteria produce antisense RNA that is complementary to the mRNA coding for the enzyme. When the antisense RNA binds to the mRNA by complementary base pairing, the mRNA cannot be translated into protein and the enzyme is not made. Noncompetitive Inhibition b. controlling the enzyme's activity (feedback inhibition). Enzyme activity can be controlled by competitive inhibition and noncompetitive inhibition. 1. With what is termed noncompetitive inhibition, the inhibitor is the end product of a metabolic pathway that is able to bind to a second site (the allosteric site) on the enzyme. Binding of the inhibitor to the allosteric site alters the shape of the enzyme's active site thus preventing binding of the first substrate in the metabolic pathway. In this way, the pathway is turned off (see Fig. 13). Fig. 13: Noncompetitive Inhibition with Allosteric Enzymes Noncompetitive Inhibition with Allosteric Enzymes When the end product (inhibitor) of a pathway combines with the allosteric site of the enzyme, this alters the enzyme's active site so it can no longer bind to the starting substrate of the pathway. This blocks production of the end product. Competitive Inhibition In the case of what is called competitive inhibition, the inhibitor is the end product of an enzymatic reaction. That end product is also capable of reacting with the enzyme's active site and prevents the enzyme from binding its normal substrate. As a result, the end product is no longer synthesized (see Fig14). Fig. 14: Competitive Inhibition of Enzyme Activity The end product (inhibitor) of a pathway binds to the active site of the first enzyme in the pathway. As a result, the enzyme can no longer bind to the starting substrate of the pathway. Gene cloning Gene cloning involve four steps: 1. The vector DNA is cleaved with one or more restriction enzyme. 2. The DNA to be cloned is joined to the vector, by ligase enzyme generating recombinant molecule. 3. The recombinant DNA are introduced into a host cell (called transformed) (e.g. bacteria). 4. The transformed colony is selected to amplified. Another method of amplifying genome is PCR. DNA Ligase Joins DNA Fragments Together to Produce a Recombinant DNA Molecule DNA ligase allows joining together any two DNA fragments. Because DNA has same chemical structure in all organisms, the simple maneuver allows DNAs from any source to be untied. Restriction Endonucleases Sticky end Blunt end Types of Vectors Plasmid Phage (virus) Cosmid Yeast Artificial Chromosome (YAC) Bacterial Artificial Chromosome (BAC) Vectors Vectors DNA molecule that is used to transfer foreign DNA fragments between cells. Investigators interested in cloning, however find it easier to manipulated, copy, and purify their recombinant DNA when it is maintained as an independent molecule, separate from the bacterial chromosome. To maintain foreign DNA in a bacterial cell, a bacterial plasmid is used as carrier, or vector. Plasmids Circular extrachromosomal DNA molecules naturally found in bacteria Not required for the survival of the bacteria. Essential under certain condition. Independent replicating. Can insert pieces up to 10kb Plasmid vectors characteristics 1. Should have selectable marker (antibiotic) 2. Should have unique restriction enzyme cleavage sites 3. Smaller plasmid are preferred for cloning. 4. Should be easily propagated in the desire host. 5. Should have large number of copies Examples of Plasmids Plasmid: pBR322 after F. Bolivar and R. Rodriguez Size (kb) :4.361 Cosmids Transformation Using bacterial cells to amplify the DNA of interest. Competent cells are able to take up foreign DNA and acquire genetic information. Ordinary Escherichia coli cells can be made competent through a treatment with Ca2+. – Competent cells have very fragile cell walls, and must be handled gently. During the transformation reaction: – Competent cells are combined with the ligation products. – Incubated on ice (DNA sticks to the outer cell walls.) – Heat Shocked (Membranes become more porous and allow DNA to enter.) Not all the competent cells will take up DNA. We will determine the frequency. – Incubated in LB broth at 37ºC for ≈ 45 min. Long enough to allow transcription and translation of ampicillin resistance gene. Candidate Identification The transformation culture is plated on special media to help identify which cells have received the recombinant plasmid. Two types of media: LB + X-gal, & LB+ X-gal + amp Selection: – Cells with the plasmid can grow on ampicillin media. – Cells without the plasmid cannot grow on ampicillin media. Screening: – Cells with a functional LacZ gene can convert X-gal to X + gal. X-gal -------------------------------> X + galactose ß-galactosidase – Colorless Blue Cells which produce ß-galactosidase form BLUE colonies. Cells which are able to grow on ampicillin without ß- galactosidase production form WHITE colonies. (Suspect fragment insert) Some possible products of the transformation reaction: Bacterial cell Genomic DNA Plasmid w/ insert Plasmid w/o insert No plasmid Ampicillin Ampicillin resistant No ampicillin resistant resistance Functional LacZ Nonfunctional No LacZ gene Blue colony on LacZ LB+X-gal+amp. No growth on White colony on Ampicillin LB+X-gal+amp. Antibiotic Resistance The medium in this petri dish contains the antibiotic Kanamycin The bacteria on the right contain Kanr, a plasmid that is resistant to Kanamycin, while the one on the left has no resistance Note the difference in growth Creating and Screening a Library Isolation of the genes encoding protein is a fundamental objective of molecular biotechnology. To identify genes DNA library for the selected organism can be generated by 1- using restriction enzyme to cut the DNA into smaller DNA fragments by using a four-cutter restriction enzyme (e.g. Sau3AI). 2- each fragments inserted into a vector. 3- then cloned and identify the cells contain the target fragments, which then isolated and characterized. Partial Restriction Endonuclease Digestion of DNAs Partial Digestion Profile Collect fragments of a given target size after digestions for different times or using different restriction endonuclease concentrations Size fractionate and combine fractions of desired target size The presence of the target DNA can be identified with a DNA probe. This depend on the formation of stable hydrogen bone between the probe and the target DNA. The binding process are carried out at high temperature. Then the probe which is labelled with radioactive isotopes or fluorescent material are incubated with bound DNA sample. If the sequence of the probe is complementary to the sample DNA sequence then the probe will hybridize with the sample DNA. Hybridization can be detected by autoradiography or fluorescence detector. Screening Colonies by Hybridization Nucleic acid probe Cells transferred to nylon membrane and lysed DNA binds to membrane, is denatured and probe hybridized Bound probe detected by autoradiography after washing membrane Screening by Immunological Assay Screening by Protein Activity This can be done when the target gene produce an enzyme that is not normally made by the host cell. A direct test can be devised to identify the members of a library that carry the particular gene encoding that enzyme. E.g. if you clone lipase gene, transformed cells are grown in the presence of trioleoglycerol and the florescent dye rhodamine B. as a result of hydrolysis of the substrate, positive colony have orange colour under UV light. e.g. if you clone amylase gene, transformed cells are grown in the presence of trioleoglycerol you can added starch to the medium where you will inoculate the bacteria contain the clone after that add iodine to test the degradation of starch based on the colour observed you can tell if your bacterial cell contain the target gene or not. Functional genetic Complementation By transfer host cell with genetic defect with plasmids of a DNA library constructed from a normal (wild type) organism and selecting the transformed cells that function normally. E.g. used E. coli with defect in one of its metabolic pathway. Cloning DNA Sequence That encode Eukaryotes protein Due to post translation modification and the presence of introns it is difficult to clone eukaryotes gene into prokaryote one. To solve this problem the following procedure can be follow. Instead of DNA, mRNA can be extracted and used as all introns region are already been removed. TO extract the mRNA from other ribosomal RNA a special isolation technique based on devising a chromatography methods where the beads are attached to poly TTTTT tail which will bind to the poly AAAAA tail present at the end of the mRNA. RNA can be eluted by treatment with buffer that breaks the A:T hydrogen bonds, thereby releasing the mRNA. Cloning DNA Sequence That encode Eukaryotes protein Before cloning mRNA must be converted into dsDNA. Which could be done into two steps: Reverse transcription of the mRNA, which will end with ssDNA. The newly formed ssDNA usually turned back on itself and few nucleotide are added to form a hairpin loop. The dsDNA can be synthesis by the addition of klenow fragment of E. coli DNA polymerase I. The Addition of nucleotide to the growing strand starting from the 3’ hydroxyl group at the end of the hairpin loop. After treating with different enzyme the mixture contain full length and short ds-CDNA. The cDNA can be cloned. After that identification procedure described earlier can be used to identify the transformed cell