Ch.5 III Regulation of Chromosome Structure PDF
Document Details
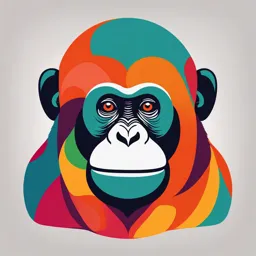
Uploaded by MindBlowingLagoon
Tags
Summary
This document discusses the regulation of chromosome structure, focusing on how DNA is packaged into chromatin and how cells access specific DNA regions. It covers mechanisms like chromatin remodeling and histone modifications, highlighting their roles in development and growth.
Full Transcript
THE REGULATION OF CHROMOSOME STRUCTURE So far, we have described how DNA is packed tightly into chromatin. But this packaging cannot be static. The DNA in chromosomes carries an enormous amount of encoded information, and it is essential that cells be able to selectively unpack its chromatin to acce...
THE REGULATION OF CHROMOSOME STRUCTURE So far, we have described how DNA is packed tightly into chromatin. But this packaging cannot be static. The DNA in chromosomes carries an enormous amount of encoded information, and it is essential that cells be able to selectively unpack its chromatin to access specific regions of DNA as needed. In this section, we discuss how a cell can expose localized regions of DNA, granting access to specific proteins and protein complexes, such as those involved in gene expression and in DNA replication and DNA repair. We then discuss how different “flavors” of chromatin structure are established and maintained—and how a cell can pass on some of this structure to its descendants, helping different cell types to sustain their identity. Although many of the details remain to be deciphered, the regulation and inheritance of chromatin structure play crucial roles in the development and growth of all eukaryotic organisms, including ourselves. Changes in Nucleosomes Allow Access to DNA Eukaryotic cells have several ways to adjust the local structure of their chromatin quickly and selectively. One mechanism takes advantage of a set of ATP-dependent chromatin-remodeling complexes. These large protein machines, present at about one copy for every five nucleosomes, can use the energy of ATP hydrolysis to change the position of nucleosomes on the DNA (Figure 5–27). By recognizing specific regions of DNA and https://nerd.wwnorton.com/nerd/231302/r/goto/cfi/90!/4?control=control-assignment<i=true 10/14/23, 9:06 AM Page 1 of 18 interacting with both the histone octamer and the DNA wrapped around it, ATP-dependent chromatin-remodeling complexes can render that DNA more accessible (or, in some cases, less accessible) to other proteins in the cell. During mitosis, many of these complexes are inactivated, which may help mitotic chromosomes maintain their tightly packed structure. (A) https://nerd.wwnorton.com/nerd/231302/r/goto/cfi/90!/4?control=control-assignment<i=true 10/14/23, 9:06 AM Page 2 of 18 (B) Figure 5–27 ATP-dependent chromatin-remodeling complexes locally reposition the DNA wrapped around nucleosomes. (A) The complexes use energy derived from ATP hydrolysis to pull on nucleosomal DNA, loosening its grip around the histone octamer. In this way, the enzyme can expose or hide a sequence of DNA, controlling its availability to other DNA-binding proteins. The blue stripes have been added to show how the DNA shifts its position. Many cycles of ATP hydrolysis are required to produce such a shift. (B) The structure of an ATP-dependent chromatin-remodeling complex, showing how the enzyme (green) cradles a nucleosome core particle, including a histone octamer (red, orange, blue, and dark green) and the DNA wrapped around it (gray). This large complex, purified from yeast, contains multiple subunits, including an ATP-driven motor (purple). (Dynamic Figure) The motor subunit (purple) of the ATP-dependent chromatin-remodeling complex https://nerd.wwnorton.com/nerd/231302/r/goto/cfi/90!/4?control=control-assignment<i=true 10/14/23, 9:06 AM Page 3 of 18 (below) repositions a piece of DNA (red) that is wound around a core of histone proteins (yellow), as shown by movement of the blue stripe. Another means of altering chromatin structure relies on the reversible chemical modification of histones, catalyzed by a large number of different histone-modifying enzymes. The tails of all four of the core histones are particularly subject to these covalent modifications, which include the addition (and removal) of acetyl, phosphate, or methyl groups (Figure 5–28A). These and other https://nerd.wwnorton.com/nerd/231302/r/goto/cfi/90!/4?control=control-assignment<i=true 10/14/23, 9:06 AM Page 4 of 18 modifications can have important consequences for the packing of the chromatin fiber. Acetylation of lysines, for instance, can reduce the affinity of the tails for adjacent nucleosomes, thereby loosening chromatin structure and allowing access to particular nuclear proteins. Figure 5–28 The pattern of modification of histone tails can determine how a stretch of chromatin is handled by the cell. (A) Schematic drawing showing the positions of the histone tails that extend from each nucleosome core particle. Each histone can be modified by the covalent attachment of a number of different chemical groups, mainly to the tails. The tail of histone H3, for example, can receive acetyl groups (Ac), methyl groups (M), or phosphate groups (P). The numbers denote the positions of the modified amino acids in the histone tail, with each amino acid designated by its one-letter code. Note that some amino acids, such as the lysine (K) at positions 9, 27, and 36 (red), can be modified by acetylation or methylation (but not by both at once). Lysines, in addition, can be modified with either one, two, or three methyl groups; trimethylation, for example, is shown in (B). Note that histone H3 contains 135 amino acids, most of which are in its globular portion (represented by the wedge); most modifications occur on the N-terminal tail, of which 36 residues are shown. (B) Different combinations of histone tail modifications can confer a specific meaning on the stretch of chromatin on which they occur, as indicated. Although 100 or so histone modifications have been cataloged, only a few have been linked definitively with a particular functional outcome in terms of chromatin structure or gene expression. Most importantly, however, these modifications generally serve as docking sites on the histone tails for a variety of regulatory proteins. Different patterns of modifications attract specific sets of non-histone chromosomal proteins to a particular stretch of chromatin—including different ATP-dependent chromatinhttps://nerd.wwnorton.com/nerd/231302/r/goto/cfi/90!/4?control=control-assignment<i=true 10/14/23, 9:06 AM Page 5 of 18 modifying complexes. Some of these proteins promote chromatin condensation, whereas others promote chromatin expansion and thus facilitate access to the DNA. Specific combinations of tail modifications, and the proteins that bind to them, have different functional outcomes for the cell: one pattern, for example, might mark a particular stretch of chromatin as newly replicated; another might indicate that the genes in that stretch of chromatin are being actively expressed; still others are associated with genes that are shut down, or silenced (Figure 5–28B). Histone-modifying enzymes thereby work in concert with ATP-dependent chromatinremodeling complexes to condense and relax stretches of chromatin, allowing local chromatin structures to change rapidly according to the needs of the cell. Both ATP-dependent chromatin-remodeling complexes and histone-modifying enzymes are themselves tightly regulated. In addition to recognizing different histone modifications, these enzymes can be brought to particular chromatin regions by interactions with proteins that bind to a specific nucleotide sequence in the DNA—or to an RNA transcribed from this DNA (a topic we return to in Chapter 8). Interphase Chromosomes Contain Both Highly Condensed and More Extended Forms of Chromatin The localized alteration of chromatin packing by remodeling complexes and histone modification has important effects on the large-scale structure of interphase chromosomes. Interphase chromatin is not uniformly packed. Instead, regions of the chromosome containing genes that are being actively expressed https://nerd.wwnorton.com/nerd/231302/r/goto/cfi/90!/4?control=control-assignment<i=true 10/14/23, 9:06 AM Page 6 of 18 are generally more extended, whereas those that contain silent genes are more condensed. The detailed structure of an interphase chromosome will differ from one cell type to the next, helping to determine which genes are switched on and which are shut down. Most cell types express only about half of the genes they contain, and many of these are active only at very low levels. The most highly condensed form of QUESTION 5–3 interphase chromatin is called Histone proteins are among the most highly conserved proteins in heterochromatin (from the Greek eukaryotes. Histone H4 proteins heteros, “different,” chromatin). This from a pea and a cow, for highly compact form of chromatin was example, differ in only 2 of 102 amino acids. Comparison of the first observed in the light microscope in gene sequences shows many the 1930s as discrete, strongly staining more differences, but only two regions within the total chromatin mass. change the amino acid sequence. These observations indicate that Heterochromatin makes up about 40% mutations that change amino acids must have been selected of a typical interphase chromosome. against during evolution. Why do About half of this heterochromatin you suppose that amino-acidremains permanently condensed—for altering mutations in histone genes are deleterious? example, in the regions around the centromere and the telomeres that cap chromosome ends (see Figure 5–14). The remaining heterochromatin contains genes whose activity has been silenced—including genes that were active in early embryogenesis, but that have been subsequently repressed when development is complete and they are no longer needed. About 60% of interphase chromatin is in the form of euchromatin (from the Greek eu, “true” or “normal,” chromatin). Although we use the term euchromatin to refer to chromatin that exists in a less condensed state than heterochromatin, it is now clear that both https://nerd.wwnorton.com/nerd/231302/r/goto/cfi/90!/4?control=control-assignment<i=true 10/14/23, 9:06 AM Page 7 of 18 euchromatin and heterochromatin are composed of mixtures of different chromatin structures (Figure 5–29). Thus, only about 20% of the human genome is packaged in the extended, “open” form of euchromatin associated with genes that are being actively expressed. Figure 5–29 The structure of chromatin varies along a single interphase chromosome. As schematically indicated by the path of the DNA molecule (represented by the central black line) and the different arbitrarily assigned colors, heterochromatin and euchromatin each represent a set of different chromatin structures with different degrees of condensation. The constitutive heterochromatin in the centromere and telomeres remains permanently condensed, whereas the facultative heterochromatin has been condensed in a manner that is only temporary. Although heterochromatin is more condensed than euchromatin, there are many stretches of euchromatin in which the resident genes are quiescent; these segments of “inactive” euchromatin (light green) are less extended than the “active” euchromatin containing genes that are expressed (dark green). Heterochromatin Can Spread Along a Chromosome to Silence Nearby Genes The regulation of these different forms of chromatin begins at the level of the nucleosome. Each type of chromatin structure is established and maintained by different sets of histone tail modifications, which attract distinct sets of non-histone chromosomal proteins. The modifications that direct the formation of the most common type of heterochromatin, for example, include the trimethylation of lysine 9 in the tail of histone H3 (see Figure 5– 28B). Once heterochromatin has been established, the heterochromatin-specific histone modifications can attract histone-modifying enzyme complexes that recognize a mark such https://nerd.wwnorton.com/nerd/231302/r/goto/cfi/90!/4?control=control-assignment<i=true 10/14/23, 9:06 AM Page 8 of 18 as a trimethylated lysine and then add the same modifications to adjacent nucleosomes on neighboring regions of DNA. These modifications can in turn recruit additional histone-modifying protein complexes, causing a wave of condensed chromatin to propagate along the chromosome. Such an extended region of heterochromatin can continue to spread until it encounters a barrier DNA sequence that stops the propagation (Figure 5–30). Figure 5–30 Heterochromatin-specific histone modifications allow heterochromatin to form and to spread. These modifications can attract “reader–writer” protein complexes containing https://nerd.wwnorton.com/nerd/231302/r/goto/cfi/90!/4?control=control-assignment<i=true 10/14/23, 9:06 AM Page 9 of 18 histone-modifying enzymes that identify and then reproduce the same histone modifications on neighboring nucleosomes. In this manner, heterochromatin can spread until it encounters a barrier DNA sequence that blocks further propagation into regions of euchromatin (Movie 5.3). As also shown, histones bearing heterochromatin-specific modifications also recruit additional heterochromatin-specific proteins that drive chromatin condensation. This process can be reversed by histone-modifying enzyme complexes that recognize and remove heterochromatin-specific marks (not shown). How do such barrier sequences curtail the expansion of heterochromatin? As one example, some barrier sequences attract histone-modifying enzyme complexes that add an acetyl group to lysine 9 of the histone H3 tail; this modification blocks the methylation of that lysine, preventing further heterochromatin formation at that site (see Figure 5–28B). Because heterochromatin is so compact, any genes that are packaged into heterochromatin tend to be silenced. The same is true for genes that become stranded in heterochromatin accidentally, when heterochromatin spreads into a region where it does not belong. Such inappropriate packaging of genes in heterochromatin can cause disease: in humans, the gene that encodes β-globin—a protein that forms part of the oxygen-carrying hemoglobin molecule—is situated near a region of heterochromatin. DNA sequencing of an individual with severe anemia revealed an inherited deletion of barrier sequence DNA, which allowed heterochromatin to spread and deactivate the βglobin gene. X-Inactivation Represents an Extreme Form of Gene Silencing Perhaps the most striking example of the use of heterochromatin to keep genes silenced is found in the interphase X chromosomes https://nerd.wwnorton.com/nerd/231302/r/goto/cfi/90!/4?control=control-assignment<i=true 10/14/23, 9:06 AM Page 10 of 18 of female mammals. In mammals, female cells contain two X chromosomes, whereas male cells contain one X and one Y. A double dose of X-chromosome products can be lethal, and female mammals have evolved a mechanism for permanently inactivating one of the two X chromosomes in each cell. At random, one or other of the two X chromosomes in each nucleus becomes highly condensed into heterochromatin early in embryonic development. Thereafter, the condensed and inactive state of that X chromosome is inherited in all of the many descendants of those cells (Figure 5–31). This process of X-inactivation, which is discussed further in Chapter 8, is responsible for the patchwork coloration of calico and tortoiseshell cats (Figure 5–32). https://nerd.wwnorton.com/nerd/231302/r/goto/cfi/90!/4?control=control-assignment<i=true 10/14/23, 9:06 AM Page 11 of 18 (A) https://nerd.wwnorton.com/nerd/231302/r/goto/cfi/90!/4?control=control-assignment<i=true 10/14/23, 9:06 AM Page 12 of 18 (B) Figure 5–31 One of the two X chromosomes is inactivated in the cells of mammalian females by heterochromatin formation. (A) Each female cell contains two X chromosomes, one from the https://nerd.wwnorton.com/nerd/231302/r/goto/cfi/90!/4?control=control-assignment<i=true 10/14/23, 9:06 AM Page 13 of 18 mother (Xm) and one from the father (Xp). At an early stage in embryonic development, one of these two chromosomes becomes condensed into heterochromatin in each cell, apparently at random. At each cell division, the same X chromosome becomes condensed (and inactivated) in all the descendants of that original cell. Thus, all mammalian females end up as mixtures (mosaics) of cells bearing either inactivated maternal or inactivated paternal X chromosomes. In most of their tissues and organs, about half the cells will be of one type, and the rest will be of the other. (B) In the nucleus of a female cell, the inactivated X chromosome can be seen as a small, discrete mass of chromatin called a Barr body, named after the physician who first observed it. In these micrographs of the nuclei of human fibroblasts, the inactivated X chromosome in the female nucleus (bottom micrograph) has been visualized by use of an antibody that recognizes proteins associated with the Barr body. The male nucleus (top) contains only a single X chromosome, which is not inactivated and thus not recognized by this antibody. Below the micrographs, a drawing shows the locations of both the active and the inactive X chromosomes in the female nucleus. (B, adapted from B. Hong et al., Proc. Natl. Acad. Sci. USA 98:8703–8708, 2001.) https://nerd.wwnorton.com/nerd/231302/r/goto/cfi/90!/4?control=control-assignment<i=true 10/14/23, 9:06 AM Page 14 of 18 Figure 5–32 The coat color of a tortoiseshell cat is dictated in large part by patterns of Xinactivation. In cats, one of the genes specifying coat color is located on the X chromosome. In female tortoiseshells (or “torties”), one X chromosome carries the form of the gene that specifies black fur, the other carries the form of the gene that specifies orange fur. Skin cells in which the X chromosome carrying the gene for black fur is inactivated will produce orange fur; those in which the X chromosome carrying the gene for orange fur is inactivated will produce black fur. The size of each patch will depend on the number of skin cells that have descended from an embryonic cell in which one or other of the X chromosomes was randomly inactivated during development (see Figure 5–31). (Cheryl Toepfer/Shutterstock.) Heterochromatin Can Be Inherited by https://nerd.wwnorton.com/nerd/231302/r/goto/cfi/90!/4?control=control-assignment<i=true 10/14/23, 9:06 AM Page 15 of 18 Subsequent Generations of Cells X-inactivation is an extreme example of a process that takes place in all eukaryotic cells—one that operates on a much finer scale to help control gene expression in cells and their progeny. When a cell divides, it can pass along its pattern of gene expression to its daughter cells. Such “cell memory” is critical for the establishment and maintenance of different cell types during the development of a complex multicellular organism. Because this memory system is not based on the nucleotide sequence of the underlying DNA—but on a pattern of gene activity—its transmission from one cell generation to the next is designated as epigenetic inheritance. One important form of cell memory Mutations in a particular gene on involves an inheritance of the the X chromosome result in color condensed chromatin structure that blindness in men. By contrast, silences genes—that is, the cell’s most women carrying the mutation have proper color vision heterochromatin. When a cell replicates but see colored objects with its DNA, each daughter double helix will reduced resolution, as though receive half of its parent’s histone functional cone cells (the photoreceptor cells responsible proteins, which contain the covalent for color vision) are spaced farther modifications that were present on the apart than normal in the retina. Can you give a plausible parent chromosome. The same enzyme explanation for this observation? complexes that are responsible for If a woman is color-blind, what could you say about her father? spreading heterochromatin along a About her mother? Explain your chromosome (see Figure 5–30) can answers. recognize these histone marks—such as the trimethyated lysine 9 on histone H3—and spread them to the neighboring nucleosomes. These modifications will then attract heterochromatin-specific proteins and reestablish the pattern of heterochromatin that was present in the parent cell (Figure 5–33). QUESTION 5–4 https://nerd.wwnorton.com/nerd/231302/r/goto/cfi/90!/4?control=control-assignment<i=true 10/14/23, 9:06 AM Page 16 of 18 In this manner, the specific regions of DNA that are packaged in heterochromatin tend to be maintained between one cell generation and the next. Figure 5–33 Heterochromatin can be inherited after DNA replication. When a chromosome is duplicated, the H3 and H4 histone proteins associated with the parent DNA are directly passed to the daughter helices. Thus, each daughter inherits half of these parent histones—along with their covalent modifications. Some of these modified histones attract “reader–writer” enzyme complexes that recognize the specific modification and spread it to the nucleosomes nearby—ultimately reestablishing the parental pattern of histone modifications and hence the parental chromatin structure. Because chromatin structure regulates gene expression, this form of epigenetic inheritance helps to provide daughter cells with the instructions they need to retain the identity of their parent cell. We will present some additional—very different—mechanisms involved in cell memory, and describe how changes in chromatin structure support the control of gene expression, in Chapter 8. https://nerd.wwnorton.com/nerd/231302/r/goto/cfi/90!/4?control=control-assignment<i=true 10/14/23, 9:06 AM Page 17 of 18 https://nerd.wwnorton.com/nerd/231302/r/goto/cfi/90!/4?control=control-assignment<i=true 10/14/23, 9:06 AM Page 18 of 18