Molecular Biology I: Nucleic Acid Metabolism Lecture 8 Slides 1-55 PDF
Document Details
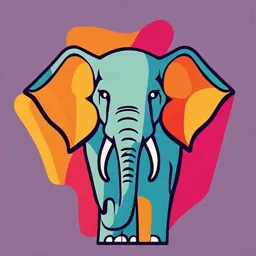
Uploaded by ZippyPelican
2024
Tags
Related
- Molecular Biology I: Nucleic Acid Metabolism Lecture 03 PDF
- Molecular Biology I: Nucleic Acid Metabolism Lecture 2 PDF
- 2024S1-Lecture-01-updated PDF: Molecular Biology I
- Molecular Biology I: Nucleic Acid Metabolism Lecture 06 (2024 S1)
- Molecular Biology I: Nucleic Acid Metabolism Lecture 07 PDF
- BIOL 3110 B - Molecular Biology I: Nucleic Acid Metabolism - 2024Fall - York University PDF
Summary
These lecture notes from a 2024 semester cover Nucleic Acid Metabolism in Molecular Biology, focusing on genome organization, chromatin structure, and different DNA sequencing techniques like Sanger and next-gen sequencing. Midterm 2 will cover material from slides 50 of Lecture 04 to 55 of Lecture 08 (this lecture).
Full Transcript
MOLECULAR BIOLOGY I: NUCLEIC ACID METABOLISM SC/BIOL 3110, 2024 S1 Lecture 08: Genome Organization Chromatin and Chromosome States in interphase cells 1 Course Announcements: I have scheduled an in-pe...
MOLECULAR BIOLOGY I: NUCLEIC ACID METABOLISM SC/BIOL 3110, 2024 S1 Lecture 08: Genome Organization Chromatin and Chromosome States in interphase cells 1 Course Announcements: I have scheduled an in-person Midterm 1 viewing session tomorrow (Fri May 31) in LSB Rm 108 from 1 to 3 pm. Please bring your student ID and the TAs will be doing the viewings with 4 students at a time. Midterm 2 is scheduled on Jun 4th (next Tues) from 10 to 11:30 in SLH A. Midterm 2 will cover material from slide 50 of Lecture 04 to slide 55 of Lecture 08 (this lecture). Format of Midterm 2 will be the same as Midterm 1. 2 Recap of last lecture: DNA sequencing methods – Sanger sequencing: Fluorescence-based Sanger sequencing 3 Recap of last lecture: Human Genome Project: Publically funded, involving 25 institutions Private company funded and executed Note: The human genome was sequenced using Sanger sequencing 4 Recap of last lecture: Next Gen sequencing: 1. Pyrosequencing Add one type of nt per round of synthesis Many parallel sequencing reactions at the same time, each can sequence 100 bp in under 3 hrs – faster than reversible termination sequencing since 5 less steps Recap of last lecture: Next Gen sequencing: 2. Reversible terminator approach (aka illumina sequencing) All 4 nucleotides added together per round of synthesis Removal of fluorescent tag and block after each round to allow incorporation of next dNTP 6 Recap of last lecture: Polymerase Chain Reaction (PCR): Need to figure our Tm to determine optimal annealing temp for BOTH forward and reverse primers 7 Recap of last lecture: Typical stages of a PCR reaction: Sometimes also referred to as the linear phase 8 Recap of last lecture: Quantitative Polymerase Chain Reaction (qPCR): Also known as Real Time PCR (RT-PCR) because can quantify amounts of PCR products (by monitoring fluorescence) in “real time”. SYBR green fluoresces only when bound to dsDNA à more double- stranded PCR products, more SYBR green fluorescence 9 Recap of last lecture: Real time Polymerase Chain Reaction (PCR): By comparing the Ct values of samples of unknown concentration with a series of standards, the amount of template DNA in an unknown reaction can be accurately determined à = absolute quantification 10 Recap of last lecture: Real time Polymerase Chain Reaction (PCR): Can also compare Ct values of two samples to get relative ratios of the amount of PCR templates in the different samples à = relative quantification DCt 11 Recap of last lecture: Quantitative PCR (qPCR): Real-time PCR using Taqman probes: Use additional sequence-specific primer that hybridizes to the PCR template for each round of PCR amplification/DNA synthesis Specifically monitor sequence-specific products and also allow for multiplexing of PCR reactions (i.e. probe multiple PCR products in single PCR reaction) 12 Recap of last lecture: Microarrays: Generate library of chemically synthesized oligonucleotides and spot on slides in specific order à = microarray Can wash away non-fully complementary targets by changing salt conc and temp of wash buffer 13 Recap of last lecture: Microarrays: Generate library of chemically synthesized oligonucleotides and spot on slides in specific order à = microarray mRNA Example: useful for testing differential expression levels of many genes in different cell types 14 Recap slide of RNA sequencing: RNA sequencing: E.g. useful for identifying the transcriptome of different cells/tissues exons 15 Recap of last lecture: Genome size comparison: C-value paradox (or C-value enigma) à the amount of haploid DNA in an organism does not correlate with evolutionary complexity Moreover, gene density (number of genes per unit length of DNA) drops for more complex organisms such as humans and more variable in eukaryotes 16 Recap of last lecture: Comparing genomes by Cot analysis: Cot value = Conc of DNA X time needed for renaturation X salt conc adjustment factor The rate of DNA re-association is proportional to the size of the genome, and to the amount of repeated sequences in the genome. 17 Recap of last lecture: Highly repetitive DNA (simple repeats): Examples include satellite, mini-satellite, and micro-satellite DNA Measure the optical density of the gradient from bottom to top: CsCl gradient à Isopycnic separation 18 Recap of last lecture: Highly repetitive DNA (complex): Transposable elements (TE) are also highly repeated sequences found in genomes of complex organisms. In fact, greater than 40% of the human genome is made up of transposable elements. e.g. LINES SINES estimates circa 2008 Not all TEs are transcribed – regulated by chromatin and epigenetic mechanisms 19 Recap of last lecture: Gene families (e.g. rRNA genes): The cluster and tandem organization of rDNA allow for coordinated transcription of these genes. Actively transcribing rRNA genes can be visualized by Electron Microscopy, first done in the 1960s by Oscar Miller (technique now known as Miller spreads). Artist’s to-scale drawing of a Miller spread Nascent RNAs emanating from each transcribing polymerase (typically 50-100 polymerases per transcription unit) give the characteristic “Christmas tree” appearance. EM micrograph showing “Christmas trees” of actively transcribing rRNA 20 Recap of last lecture: Globin gene clusters and expression profiles: 21 Recap of last lecture: Genome size comparison: Genome = collection of all genes; proteome = collection of all proteins ~ 20,000 protein-coding genes à highly complex proteome 22 Recap of last lecture: Packaging and organization of the prokaryotic genome: E. coli is the best studied and model organism of choice for prokaryotic research. Because E. coli has a single closed circular genome, it was often assumed that all bacteria have the same genome organization. However; bacteria can have circular, linear or multipartite genomes. Artist’s rendition of an E. coli cell Picture of E. coli by scanning EM 23 Recap of last lecture: How to pack the whole E. coli genome into a 2-by-0.5-micron cell: 1. The E. coli genome is supercoiled, which results in more compact dimensions. 2. E. coli DNA is wrapped around HU (and other) proteins to form the nucleoid. These DNA-protein complexes not only compact the genome, but together with Topoisomerase I and DNA gyrase, generate and maintain supercoiling of the DNA. 3. The supercoiled DNA/HU complexes form loops that radially extend from a central protein core. The bacterial genome consists of a large number of loops of duplex DNA. The loops can unwind and extend during active transcription 24 Packaging of the eukaryotic genome: 25 Packaging of the eukaryotic genome: Genome compaction and folding – the problem: The human genome has ~ 6.6 X 109 bp of DNA. For B-form DNA, the distance between base pairs is ~ 0.34 X 10-9 m Therefore, the total length of DNA in a human cell is ~ 2 m! All this has to fit into a nucleus of ~ 10 micron in diameter 26 Packaging of the eukaryotic genome: Genome compaction and folding – the problem: How? EM picture of a histone-depleted chromosome Scanning EM picture of chromosomes 27 Packaging of the eukaryotic genome: Genome compaction and folding – the problem: 28 Packaging of the eukaryotic genome: Historical figures (some we have talked about before): Walter Flemming: coined the term chromatin to describe the substance within the cell nucleus that is readily stained by dyes (~ 1881) had the foresight to say, Possibly chromatin is identical to nuclein, but if not, it follows that one carries the other. Albrecht Kossel: coined the term histon to describe the proteins he found by extracting avian erythrocyte nuclei using diluted acids (1884) first to notice that histones are acid-soluble (… still the best way to extract histones) Emil Heitz: defined the term heterochromatin as chromosomal material that remains condensed in interphase nuclei (1928) proposed that …euchromatin [true chromatin] is genicly active, heterochromatin is genicly passive” 29 Packaging of the eukaryotic genome: Historical figures: Vincent Allfrey: found that histones inhibited RNA synthesis in isolated thymus nuclei (1961) proposed that acetylation and methylation of histones may have possible roles in regulating RNA synthesis (1964) The Olins, and Christopher Woodcock: independently observed the “beads on a string” feature of chromatin by EM (1973) each “bead” is what we now call a nucleosome 30 Packaging of the eukaryotic genome: Historical figures: Roger Kornberg: In 1974, proposed that ~ 200 bp of DNA forms a complex with 4 histone pairs (based on EM images, nuclease digestion patterns, X-ray diffraction data, and biochemical purification of chromatin components). Treament of chromatin with micrococcal nuclease à preferentially cuts between the “beads”, i.e. cuts the linker DNA Chromatin was digested with increasing amounts of micrococcal nuclease, DNA was then extract and analyzed by agarose gel electrophoresis 31 ~ 200 bp Packaging of the eukaryotic genome: The nucleosome: The DNA-histone complex is called a nucleosome, and is defined as the repeating unit of chromatin. Each nucleosome contains 147 bp of DNA wrapped around 2 copies each of H2A, H2B, H3 and H4. Note that the 200 bp band protected from MNase digestion also includes the linker DNA between nucleosomes. Some nucleosomes contain a 5th histone called H1, which binds the linker DNA that enter and exit the core nucleosome particle. 32 Packaging of the eukaryotic genome: The nucleosome: The DNA-histone complex is called a nucleosome, and is defined as the repeating unit of chromatin. Each nucleosome contains 147 bp of DNA wrapped around 2 copies each of H2A, H2B, H3 and H4. Note that the 200 bp band protected from MNase digestion also includes the linker DNA between nucleosomes. Some nucleosomes contain a 5th histone called H1, which binds the linker DNA that enter and exit the core nucleosome particle. 33 Packaging of the eukaryotic genome: General properties of histones: Histones are small basic (i.e. positively charged) proteins that interact with each other to form an octameric structure called this histone octamer. Histones contain many lysines and arginines, which explain the positively charged nature of these proteins. (how does this complement the physical nature of DNA?) They are some of the most highly conserved proteins across species – so much so that antibodies that recognize human histones will often also recognize histones from other organisms (from yeast to flies to mice to human). 34 Packaging of the eukaryotic genome: General properties of histones: All histones have very similar domain structures: consisting of relatively unstructured N- terminal tails and highly structured globular domains (making up the histone folds) in the middle. H2A and H2B have longer C-terminal tails whereas H3 and H4 have very short C- terminal tails. The 4 core histones all share similar structures 35 Packaging of the eukaryotic genome: General properties of core histones: The histone fold of core histones (made up of the 3 central a-helices of each histone) form hand-shake motifs that allow dimerization of H2A with H2B, and H3 with H4. N C N-terminal tail helicies C-term tail histone fold = hand-shake motif 36 Packaging of the eukaryotic genome: Histone octamer assembly: Two H3-H4 dimers form a tetramer, which form the central disc of the octamer. Then two H2A-H2B dimers are added to the H3-H4 tetramer (one dimer on top and the other dimer on the bottom) to form the full octamer. 37 Packaging of the eukaryotic genome: Histone octamer assembly: The histone H3-H4 dimer and H2A-H2B dimer are formed from the hand-shake interaction. An H3-H4 tetramer forms the scaffold of the octamer onto which two H2A and H2B dimers are added 38 Packaging of the eukaryotic genome: Nucleosome assembly: The histone octamer binds and wraps ~ 1.7 turns of DNA (~ 147 bp of DNA) in a left- handed manner. The addition of one H1 molecule wraps another 20 bp, resulting in 2 full turns of DNA around the octamer. Note that nucleosome assembly requires the activities of histone chaperones and ATP-dependent chromatin remodeling complexes. Top view H3-H4 tetramer Side view 2x H2A- H2B dimers 39 Packaging of the eukaryotic genome: First order of DNA compaction: Nucleosomes are linked together by linker DNA. The oligonucleosomes form the 11 nm “beads on a string” structure. The wrapping of DNA around nucleosomes compact DNA by 6 – 8 fold. EM picture of the 11 nm beads on a string 40 Packaging of the eukaryotic genome: Second order of DNA compaction: The 11 nm fibre is further coiled into a shorter and thicker fibre termed the 30 nm fiber. H1 is needed to stabilize this higher order structure. The 30 nm fibre compacts DNA by ~ 50 - 100 fold. EM picture of a 30 nm fibre – note chromatin was spread in a moderate ionic strength buffer to maintain the higher order sturcture. Size marker = 50 nm 41 Packaging of the eukaryotic genome: Second order of DNA compaction: The 30 nm fibre is a coiled structure that has ~ 6 nucleosomes per turn. In vitro studies showed that addition of H1 and increased ionic strength of the surrounding buffer both promote the formation of the 30 nm fibre. The solenoid model has 6 nucleosomes 42 per turn Packaging of the eukaryotic genome: Higher orders of DNA compaction: Higher order structures beyond the 30 nm fibre are poorly understood and subject to much speculation. For example, it is thought that the solenoid 30 nm fibres further loops to form 300 nm domains that are anchored to a protein scaffold or nuclear matrix. 43 Packaging of the eukaryotic genome: Higher orders of DNA compaction: Hypothetical looping of the 30 nm solenoid anchored to a protein scaffold. These radial loops further coil to form a 700 nm structure. 44 Packaging of the eukaryotic genome: Higher orders of DNA compaction: Evidence for the high order structures is mostly based on EM pictures. Low magnification High magnification Histone- depleted chromosome spreads DNA loops protein scaffold The matrix attachment sites at the bases of the loops have topoisomerases to help regulate supercoiling of the DNA 45 Packaging of the eukaryotic genome: Higher orders of DNA compaction: See movies for animated description of chromatin compaction. E.g. http://www.hhmi.org/biointeractive/dna-packaging 46 Packaging of the eukaryotic genome: The metaphase chromosome is the highest compacted state of chromatin: Chromosomes are condensed during mitosis to facilitate chromosome segregation during cell division. DNA has to compact 10,000 fold to form mitotic chromosomes. Telomeres are the ends of chromosomes Centromeres are where the sister chromatids are attached together EM picture and schematic drawing of a typical metaphase chromosome 47 Packaging of the eukaryotic genome: Types of metaphase chromosomes: Our chromosomes are at the most compact state at metaphase à can arrest cells at metaphase, isolate chromosomes and analyze = cytogenetics Approximately, not exactly Positioning of the centromeres also help 48 classify/identify chromosomes Packaging of the eukaryotic genome: Metaphase chromosomes are useful diagnostic tools for cytogeneticists: Cytogenetics is the study of chromosomes and their abnormalities. Karyotype is the number and appearance of p arm chromosomes isolated from eukaryotic cells. Since 1960s, various staining protocols have been developed to produce reproducible patterns of dark and light bands along the length of each chromosome. These banding patterns become the barcodes with which cytogeneticists can easily identify chromosomes, detect subtle deletions, q arm inversions, insertions, translocations and more complex rearrangements. The molecular basis of the banding patterns is unknown. The human X chromosome can be divided into distinct regions by its banding pattern 49 Packaging of the eukaryotic genome: Human karyotypes: Karyotyping is a test to examine chromosomes in a sample of cells – can help identify genetic problems as the cause of a disorder or disease. Karyotype analysis of human chromosomes using G-banding 50 Packaging of the eukaryotic genome: Human karyotypes: Karyotyping is a test to examine chromosomes in a sample of cells – can help identify genetic problems as the cause of a disorder or disease. Sister chromatids Karyotype analysis of human chromosomes using G-banding 51 Packaging of the eukaryotic genome: Human karyotypes: Aneuploidy = aberrations in the normal chromosome number in an organism Trisomy 21 correlates with the development of Down syndrome Karyotype analysis of human chromosomes using G-banding 52 Packaging of the eukaryotic genome: Human karyotypes: Spectral karyotyping (SKY) utilizes fluorescent probes to “paint” human chromosomes à allow easier identification of chromosomes and easier detection of abnormalities such as translocations. Technique is based on the hybridization of fluorescent- DNA probes to specific human chromosomes 53 Packaging of the eukaryotic genome: Technique: Fluorescence In Situ Hybridization (FISH): The fluorescent labeling of SKY is based on the technique: FISH. “In situ” means in their natural positions within a chromosome. FISH can be applied to visualize specific genes on chromosomes, or, in the case of SKY, probes can be generated to label entire chromosomes. 54 Packaging of the eukaryotic genome: How to generate fluorescent probes for SKY: FACS = Fluorescence activated cell sorting 1. Use FACS to sort (based on DNA content, which is proportional to size of chromosomes) and isolate individual chromosomes from donor genomes. 2. Use PCR to amplify DNA from each chromosome and fluorescently label them at the same time. 3. Add non-fluorescently labeled Cot-1 DNA (human repetitive sequences) for blocking purpose. 4. Mix fluorescent probes with metaphase chromosomes to be analyzed and perform hybridization, detection, and analysis by General scheme for generating fluorescent probes for SKY fluorescence microscopy. 55