Chapter 13 Landing Gear (PDF)
Document Details
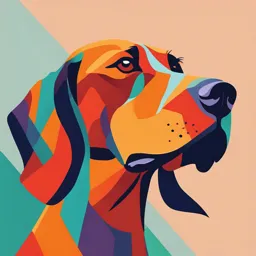
Uploaded by DaringDream
Tags
Summary
This chapter details various types of landing gear systems, including components like shimmy dampers and aircraft wheels. It explains the construction, inspection, and maintenance procedures for these systems and emphasizes the importance of proper torque on axle nuts. Safety precautions during tire removal are also addressed.
Full Transcript
Indicator rod connected to replenishing piston Replenishing piston Spring Replenishing chamber Valve orifice Abutment and valve assemblyy Rotating vane Fluid port Filler Fille er cap Replenishing check valve A B B A Abutment flange Rotating vane Key Fluid port Wing shaft Hollow dowel pin Dowel pin P...
Indicator rod connected to replenishing piston Replenishing piston Spring Replenishing chamber Valve orifice Abutment and valve assemblyy Rotating vane Fluid port Filler Fille er cap Replenishing check valve A B B A Abutment flange Rotating vane Key Fluid port Wing shaft Hollow dowel pin Dowel pin Packing spring P Hydraulic seal Wing shaft packing Mounting flange Wing shaft Spring retainer Figure 13-56. A typical vane-type shimmy damper. as the fluid can be forced through the orifice. Thus, the gear oscillation is dissipated by the rate of fluid flow. An internal spring-loaded replenishing reservoir keeps pressurized fluid in the working chambers and thermal compensation of the orifice size is included. As with the piston type shimmy damper, the vane-type damper should be inspected for leaks and kept serviced. A fluid level indicator protrudes from the reservoir end of the unit. Non-Hydraulic Shimmy Damper Non-hydraulic shimmy dampers are currently certified for many aircraft. They look and fit similar to piston-type shimmy dampers but contain no fluid inside. In place of the metal piston, a rubber piston presses out against the inner diameter of the damper housing when the shimmy motion is received through the shaft. The rubber piston rides on a very thin film of grease and the rubbing action between the piston and the housing provides the damping. This is known as surface-effect damping. The materials used to construct this type of shimmy damper provide a long service life without the need to ever add fluid to the unit. [Figure 13-57] Aircraft Wheels Aircraft wheels are an important component of a landing gear system. With tires mounted upon them, they support the entire weight of the aircraft during taxi, takeoff, and landing. The typical aircraft wheel is lightweight, strong, and made Figure 13-57. A non-hydraulic shimmy damper uses a rubber piston with lubricant that dampens via motion against the inner diameter of the unit housing. from aluminum alloy. Some magnesium alloy wheels also exist. Early aircraft wheels were of single piece construction, much the same as the modern automobile wheel. As aircraft tires were improved for the purpose they serve, they were made stiffer to better absorb the forces of landing without blowing out or separating from the rim. Stretching such a tire over a single piece wheel rim was not possible. A two-piece wheel was developed. Early two-piece aircraft wheels were essentially one-piece wheels with a removable rim to allow mounting access for the tire. These are still found on older aircraft. [Figure 13-58] Later, wheels with two nearly symmetrical halves were developed. Nearly all modern aircraft wheels are of this two-piece construction. [Figures 13-59 and 13-60] 13-33 Removable flange Bearing rollers Snap ring Flat base Grease retainer Fairing disc Drop center Lockring Removable flange Wheel casting Bearing rollers Fairing retaining screw Drop center wheel Flat base wheel Figure 13-58. Removable flange wheels found on older aircraft are either drop center or flat base types. to fit slots in the perimeter of the brake rotor. Some small aircraft wheels have provisions for bolting the brake rotor to the inner wheel half. Regardless, the inner wheel half is distinguishable from the outer wheel half by its brake mounting feature. [Figure 13-61] Figure 13-59. Two-piece split-wheel aircraft wheels found on modern light aircraft. Wheel Construction The typical modern two-piece aircraft wheel is cast or forged from aluminum or magnesium alloy. The halves are bolted together and contain a groove at the mating surface for an o-ring, which seals the rim since most modern aircraft utilize tubeless tires. The bead seat area of a wheel is where the tire actually contacts the wheel. It is the critical area that accepts the significant tensile loads from the tire during landing. To strengthen this area during manufacturing, the bead seat area is typically rolled to prestress it with a compressive stress load. Inboard Wheel Half Wheel halves are not identical. The primary reason for this is that the inboard wheel half must have a means for accepting and driving the rotor(s) of the aircraft brakes that are mounted on both main wheels. Tangs on the rotor are fitted into steel reinforced keyways on many wheels. Other wheels have steel keys bolted to the inner wheel halves. These are made Both wheel halves contain a bearing cavity formed into the center that accepts the polished steel bearing cup, tapered roller bearing, and grease retainer of a typical wheel bearing set-up. A groove may also be machined to accept a retaining clip to hold the bearing assembly in place when the wheel assembly is removed. The wheel bearings are a very important part of the wheel assembly and are discussed in a later section of this chapter. The inner wheel half of a wheel used on a high-performance aircraft is likely to have one or more thermal plugs. [Figure 13-62] During heavy braking, temperatures can become so great that tire temperature and pressure rise to a level resulting in explosion of the wheel and tire assembly. The thermal plug core is filled with a low melting point alloy. Before tire and wheel temperatures reach the point of explosion, the core melts and deflates the tire. The tire must be removed from service, and the wheel must be inspected in accordance with the wheel manufacturer’s instructions before return to service if a thermal plug melts. Adjacent wheel assemblies should also be inspected for signs of damage. A heat shield is commonly installed under the inserts designed to engage the brake rotor to assist in protecting the wheel and tire assembly from overheating. An overinflation safety plug may also be installed in the inner wheel half. This is designed to rupture and release all 13-34 Plug D INB Inside wheel half Outside wheel half Heat shield FW D O-ring Thermal plug Inboard wheel half Inflation valve A B Brake rotor key Inner wheel bearing Valve extension Outer wheel bearing Axle Brake assembly Transducer Tire Wheel balance weight Outboard wheel half Inboard wheel half A Wheel half tie bolts Camlocks (8 places) Hubcap fairing (outboard wheel only) B O-ring Bracket Valve Extension Valve extension assembly Figure 13-60. Features of a two piece aircraft wheel found on a modern airliner. of the air in the tire should it be over inflated. The fill valve is also often installed in the inner wheel half with the stem extending through holes in the outer wheel half to permit access for inflation and deflation. Outboard Wheel Half The outboard wheel half bolts to the inboard wheel half to make up the wheel assembly upon which the tire is mounted. The center boss is constructed to receive a bearing cup and bearing assembly as it does on the inboard wheel half. The outer bearing and end of the axle is capped to prevent contaminants from entering this area. Aircraft with anti-skid brake systems typically mount the wheel-spin transducer here. It is sealed and may also serve as a hub cap. The 737 outer wheel half illustrated in Figure 13-60 also has a hub cap fairing over the entire wheel half. This is to fair it with 13-35 Wheel Inspection An aircraft wheel assembly is inspected while on the aircraft as often as possible. A more detailed inspection and any testing or repairs may be accomplished with the wheel assembly removed from the aircraft. On Aircraft Inspection The general condition of the aircraft wheel assemblies can be inspected while on the aircraft. Any signs of suspected damage that may require removal of the wheel assembly from the aircraft should be investigated. Proper Installation Figure 13-61. Keys on the inner wheel half of an aircraft wheel used to engage and rotate the rotors of a disc brake. Thermal plug Thermal plug Inside wheel half Outside wheel half Heat shield Figure 13-62. Heavy use of the aircraft brakes can cause tire air temperature and pressure to rise to a level resulting in explosion of the wheel assembly. To alleviate this, thermal plug(s) mounted in the inner wheel half of a high performance aircraft wheels are made with a fusible core that melts and releases the air from the tire before explosion. the wind since the outer wheel half does not close behind a gear door on this aircraft. Hub caps may also be found on fixed gear aircraft. The outboard wheel half provides a convenient location of the valve stem used to inflate and deflate tubeless tires. Alternately, it may contain a hole through which a valve stem extension may pass from the inner wheel half or the valve stem itself may fit through such a hole if a tube-type tire is used. The landing gear area is such a hostile environment that the technician should inspect the landing gear including the wheels, tires, and brakes whenever possible. Proper installation of the wheels should not be taken for granted. All wheel tie bolts and nuts must be in place and secure. A missing bolt is grounds for removal, and a thorough inspection of the wheel halves in accordance with the wheel manufacturer’s procedures must be performed due to the stresses that may have occurred. The wheel hub dust cap and anti-skid sensor should also be secure. The inboard wheel half should interface with the brake rotor with no signs of chafing or excessive movement. All brake keys on the wheel must be present and secure. Examine the wheels for cracks, flaked paint, and any evidence of overheating. Inspect thermal plugs to ensure no sign of the fusible alloy having been melted. Thermal plugs that have permitted pressure loss in the tire require that the wheel assembly be removed for inspection. All other wheels with brakes and thermal plugs should be inspected closely while on the aircraft to determine if they too have overheated. Each wheel should be observed overall to ensure it is not abnormally tilted. Flanges should not be missing any pieces, and there should be no areas on the wheel that show significant impact damage. Axle Nut Torque Axle nut torque is of extreme importance on an aircraft wheel installation. If the nut is too loose, the bearing and wheel assembly may have excessive movement. The bearing cup(s) could loosen and spin, which could damage the wheel. There could also be impact damage from the bearing rollers which leads to bearing failure. [Figure 13-63] An over-torqued axle nut prevents the bearing from properly accepting the weight load of the aircraft. The bearing spins without sufficient lubrication to absorb the heat caused by the higher friction level. This too leads to bearing failure. All aircraft axle nuts must be installed and torqued in accordance with the airframe manufacturer’s maintenance procedures. 13-36 tire can create a catastrophic failure that could be lethal to the technician. It is also important to let aircraft tires cool before removal. Three hours or more is needed for cool down. Approach the wheel assembly from the front or rear, not broadside. Do not stand in the path of the released air and valve core trajectory when removing air from the tire as it could seriously injure the technician should it release from the valve stem. Scalloping Note: As a precautionary measure, remove only one tire and wheel assembly from a pair at a time. This leaves a tire and wheel assembly in place should the aircraft fall off its jack, resulting in less chance of damage to the aircraft and injury to personnel. Figure 13-63. Improper loose torque on the axle nut can cause excessive end play leading to bearing race damage known as scalloping. Eventually, this leads to bearing failure. Off Aircraft Wheel Inspection Discrepancies found while inspecting a wheel mounted on the aircraft may require further inspection with the wheel removed from the aircraft. Other items such as bearing condition, can only be performed with the wheel assembly removed. A complete inspection of the wheel requires that the tire be removed from the wheel rim. Observe the following caution when removing a wheel assembly from an aircraft. Caution: Deflate the tire before starting the procedure of removing the wheel assembly from the aircraft. Wheel assemblies have been known to explode while removing the axle nut, especially when dealing with high pressure, high performance tires. The torque of the nut can be the only force holding together a defective wheel or one with broken tie bolts. When loosened, the high internal pressure of the Loosening the Tire from the Wheel Rim After inflation and usage, an aircraft tire has a tendency to adhere to the wheel, and the bead must be broken to remove the tire. There are mechanical and hydraulic presses designed for this purpose. In the absence of a device specifically made for the job, an arbor press can be used with patience working sequentially around the wheel as close as possible to the bead. [Figure 13-64] As stated above, there should be no air pressure in the tire while it is being pressed off of the wheel. Never pry a tire off of the rim with a screwdriver or other device. The wheels are relatively soft. Any nick or deformation causes a stress concentration that can easily lead to wheel failure. Disassembly of the Wheel Disassembly of the wheel should take place in a clean area on a flat surface, such as a table. Remove the wheel bearing first and set aside for cleaning and inspecting. The tie bolts can then be removed. Do not use an impact tool to disassemble the tie bolts. Aircraft wheels are made of relatively soft Figure 13-64. Tire beads must be broken from the wheel to remove the tire. A mechanical removal tool designed for breaking the bead is shown in (A); a hydraulic press designed with the capacity for large aircraft wheels is shown in (B); and an arbor press is shown in (C). All are tools available to the technician for this purpose. 13-37 aluminum and magnesium alloys. They are not designed to receive the repeated hammering of an impact tool and will be damaged if used. Cleaning the Wheel Assembly Clean the wheel halves with the solvent recommended by the wheel manufacturer. Use of a soft brush helps this process. Avoid abrasive techniques, materials, and tools, such as scrapers, capable of removing the finish off of the wheel. Corrosion can quickly form and weaken the wheel if the finish is missing in an area. When the wheels are clean, they can be dried with compressed air. Cleaning the Wheel Bearings The bearings should be removed from the wheel to be cleaned with the recommended solvent, such as varsol, naptha, or Stoddard® solvent. Soaking the bearings in solvent is acceptable to loosen any dried-on grease. Bearings are brushed clean with a soft bristle brush and dried with compressed air. Never rotate the bearing while drying with compressed air. The high-speed metal to metal contact of the bearing rollers with the race causes heat that damages the metal surfaces. The bearing parts could also cause injury should the bearing come apart. Always avoid steam cleaning of bearings. The surface finish of the metals will be compromised leading to early failure. Wheel Bearing Inspection Once cleaned, the wheel bearing is inspected. There are many unacceptable conditions of the bearing and bearing cup, which are grounds for rejection. In fact, nearly any flaw detected in a bearing assembly is likely to be grounds for replacement. Figure 13-65. Galling is caused by rubbing of mating surfaces. The metal gets so hot it welds, and the surface metal is destroyed as the motion continues and pulls the metal apart in the direction of motion. Figure 13-66. Spalling is a chipped away portion of the hardened surface of a bearing roller or race. Common conditions of a bearing that are cause for rejection are as follows: Galling—caused by rubbing of mating surfaces. The metal gets so hot it welds, and the surface metal is destroyed as the motion continues and pulls the metal apart in the direction of motion. [Figure 13-65] Spalling—a chipped away portion of the hardened surface of a bearing roller or race. [Figure 13-66] Overheating—caused by lack of sufficient lubrication results in a bluish tint to the metal surface. The ends of the rollers shown were overheated causing the metal to flow and deform, as well as discolor. The bearing cup raceway is usually discolored as well. [Figure 13-67] Brinelling—caused by excessive impact. It appears as indentations in the bearing cup raceways. Any static overload Figure 13-67. Overheating caused by lack of sufficient lubrication results in a bluish tint to the metal surface. The ends of the rollers shown were overheated causing the metal to flow and deform, as well as discolor. The bearing cup raceway is usually discolored as well. 13-38 or severe impact can cause true brinelling that leads to vibration and premature bearing failure. [Figure 13-68] False Brinelling—caused by vibration of the bearing while in a static state. Even with a static overload, lubricant can be forced from between the rollers and the raceway. Submicroscopic particles removed at the points of metal-tometal contact oxidize. They work to remove more particles spreading the damage. This is also known as frictional corrosion. It can be identified by a rusty coloring of the lubricant. [Figure 13-69] Staining and surface marks—located on the bearing cup as grayish black streaks with the same spacing as the rollers and caused by water that has gotten into the bearing. It is the first stage of deeper corrosion that follows. [Figure 13-70] Etching and corrosion—caused when water and the damage caused by water penetrates the surface treatment of the bearing element. It appears as a reddish/brown discoloration. [Figure 13-71] Figure 13-69. False brinelling is caused by vibration of the bearing while in a static state. Even with a static overload, it can force the lubricant from between the rollers and the raceway. Submicroscopic particles removed at the points of metal-to-metal contact oxidize. They work to remove more particles spreading the damage. This is also known as frictional corrosion. It can be identified by a rusty coloring of the lubricant. Bruising—caused by fine particle contamination possibly from a bad seal or improper maintenance of bearing cleanliness. It leaves a less than smooth surface on the bearing cup. [Figure 13-72] The bearing cup does not require removal for inspection; however, it must be firmly seated in the wheel half boss. There should be no evidence that a cup is loose or able to spin. [Figure 13-73] The cup is usually removed by heating the wheel in a controlled oven and pressing it out or tapping it out with a non-metallic drift. The installation procedure is similar. The wheel is heated, and the cup is cooled with dry ice before it is tapped into place with a non-metallic hammer or drift. The outside of the race is often sprayed with Figure 13-70. Staining and surface marks on the bearing cup that are grayish black streaks with the same spacing as the rollers are caused by water that has gotten into the bearing. It is the first stage of deeper corrosion that will follow. Figure 13-68. Brinelling is caused by excessive impact. It appears as indentations in the bearing cup raceways. Any static overload or severe impact can cause true brinelling, which leads to vibration and premature bearing failure. Figure 13-71. Etching and corrosion is caused when water, and the damage caused by water, penetrates the surface treatment of the bearing element. It appears as a reddish/brown discoloration. 13-39 A pressure bearing lubricating tool Figure 13-72. Bruising is caused by fine particle contamination possibly from a bad seal or improper maintenance of bearing cleanliness. It leaves a less than smooth surface on the bearing cup. Figure 13-73. Bearing cups should be tight in the wheel boss and should never rotate. The outside of a bearing cup that was spinning while installed in the wheel is shown. primer before insertion. Consult the wheel manufacturer’s maintenance manual for specific instructions. Bearing Handling & Lubrication Handling of bearings is of the utmost importance. Contamination, moisture, and vibration, even while the bearing is in a static state, can ruin a bearing. Avoid conditions where these may affect bearings and be sure to install and torque bearings into place according manufacturer’s instructions. Proper lubrication is a partial deterrent to negative environmental impacts on a bearing. Use the lubricant recommended by the manufacturer. Use of a pressure bearing packing tool or adapter is also recommended as the best method to remove any contaminants from inside the bearing that may have remained after cleaning. [Figure 13-74] Inspection of the Wheel Halves A thorough visual inspection of each wheel half should be conducted for discrepancies specified in the wheel Figure 13-74. A pressure bearing lubricating tool. manufacturer’s maintenance data. Use of a magnifying glass is recommended. Corrosion is one of the most common problems encountered while inspecting wheels. Locations where moisture is trapped should be checked closely. It is possible to dress out some corrosion according to the manufacturer’s instructions. An approved protective surface treatment and finish must be applied before returning the wheel to service. Corrosion beyond stated limits is cause for rejection of the wheel. In addition to corrosion, cracks in certain areas of the wheel are particularly prevalent. One such area is the bead seat area. [Figure 13-75] The high stress of landing is transferred to the wheel by the tire in this contact area. Hard landings produce distortion or cracks that are very difficult to detect. This is a concern on all wheels and is most problematic in highpressure, forged wheels. Dye penetrant inspection is generally ineffective when checking for cracks in the bead area. There is a tendency for cracks to close up tightly once the tire is dismounted, and the stress is removed from the metal. Eddy 13-40 Key & Key Screw Inspection On most aircraft inner wheel halves, keys are screwed or bolted to the wheel to drive the brake disc(s). The drive keys are subject to extreme forces when the brakes are applied. As mentioned, there should be no movement between the wheel and the keys. The bolts should be checked for security, and the area around the keys should be inspected for cracks. There is also a limitation on how worn the keys can be since too much wear allows excessive movement. The wheel manufacturer’s maintenance instructions should be used to perform a complete inspection of this critical area. Bead seat area Figure 13-75. The bead seat areas of a light aircraft wheel set. Eddy current testing for cracks in the bead seat area is common. current inspection of the bead seat area is required. Follow the wheel manufacturer’s instruction when performing the eddy current check. The wheel brake disc drive key area is another area in which cracks are common. The forces experienced when the keys drive the disc against the stopping force of the brakes are high. Generally, a dye penetrant test is sufficient to reveal cracks in this area. All drive keys should be secure with no movement possible. No corrosion is permitted in this area. [Figure 13-76] Wheel Tie Bolt Inspection Wheel half tie bolts are under great stress while in service and require inspection. The tie bolts stretch and change dimension usually at the threads and under the bolt head. These are areas where cracks are most common. Magnetic particle inspection can reveal these cracks. Follow the maintenance manual procedures for inspecting tie bolts. Figure 13-76. Inspection for cracks in the wheel disc drive key area is performed with dye penetrant on many wheels. Fusible Plug Inspection Fusible plugs or thermal plugs must be inspected visually. These threaded plugs have a core that melts at a lower temperature than the outer part of the plug. This is to release air from the tire should the temperature rise to a dangerous level. A close inspection should reveal whether any core has experienced deformation that might be due to high temperature. If detected, all thermal plugs in the wheel should be replaced with new plugs. [Figure 13-77] Balance Weights The balance of an aircraft wheel assembly is important. When manufactured, each wheel set is statically balanced. Weights are added to accomplish this if needed. They are a permanent part of the wheel assembly and must be installed to use the wheel. The balance weights are bolted to the wheel halves and can be removed when cleaning and inspecting the wheel. They must be re-fastened in their original position. When a tire is mounted to a wheel, balancing of the wheel and tire assembly may require that additional weights be added. These are usually installed around the circumference of the outside of the wheel and should not be taken as substitutes for the factory wheel set balance weights. [Figure 13-78] Figure 13-77. Visually inspect the core of a thermal or fusible plug for deformation associated with heat exposure. Replace all of the plugs if any appear to have begun to deform. 13-41 disc brake system. Single, dual, and multiple disc brakes are common types of brakes. Segmented rotor brakes are used on large aircraft. Expander tube brakes are found on older large aircraft. The use of carbon discs is increasing in the modern aviation fleet. Balance weights Outboard wheel half Single Disc Brakes Inboard wheel half Figure 13-78. Two piece aircraft wheels are statically balanced when manufactured and may include weights attached to each wheel half that must stay with the wheel during its entire serviceable life. Aircraft Brakes Very early aircraft have no brake system to slow and stop the aircraft while it is on the ground. Instead, they rely on slow speeds, soft airfield surfaces, and the friction developed by the tail skid to reduce speed during ground operation. Brake systems designed for aircraft became common after World War I as the speed and complexity of aircraft increased, and the use of smooth, paved runway surfaces proliferated. All modern aircraft are equipped with brakes. Their proper functioning is relied upon for safe operation of the aircraft on the ground. The brakes slow the aircraft and stop it in a reasonable amount of time. They hold the aircraft stationary during engine run-up and, in many cases, steer the aircraft during taxi. On most aircraft, each of the main wheels is equipped with a brake unit. The nose wheel or tail wheel does not have a brake. In the typical brake system, mechanical and/or hydraulic linkages to the rudder pedals allow the pilot to control the brakes. Pushing on the top of the right rudder pedal activates the brake on the right main wheel(s) and pushing on the top of the left rudder pedal operates the brake on the left main wheel(s). The basic operation of brakes involves converting the kinetic energy of motion into heat energy through the creation of friction. A great amount of heat is developed and forces on the brake system components are demanding. Proper adjustment, inspection, and maintenance of the brakes is essential for effective operation. Types & Construction of Aircraft Brakes Modern aircraft typically use disc brakes. The disc rotates with the turning wheel assembly while a stationary caliper resists the rotation by causing friction against the disc when the brakes are applied. The size, weight, and landing speed of the aircraft influence the design and complexity of the Small, light aircraft typically achieve effective braking using a single disc keyed or bolted to each wheel. As the wheel turns, so does the disc. Braking is accomplished by applying friction to both sides of the disc from a non-rotating caliper bolted to the landing gear axle flange. Pistons in the caliper housing under hydraulic pressure force wearable brake pads or linings against the disc when the brakes are applied. Hydraulic master cylinders connected to the rudder pedals supply the pressure when the upper halves of the rudder pedals are pressed. Floating Disc Brakes A floating disc brake is illustrated in Figure 13-79. A more detailed, exploded view of this type of brake is shown in Figure 13-80. The caliper straddles the disc. It has three cylinders bored through the housing, but on other brakes this number may vary. Each cylinder accepts an actuating piston assembly comprised mainly of a piston, a return spring, and an automatic adjusting pin. Each brake assembly has six brake linings or pucks. Three are located on the ends of the pistons, which are in the outboard side of the caliper. They are designed to move in and out with the pistons and apply pressure to the outboard side of the disc. Three more linings are located opposite of these pucks on the inboard side of the caliper. These linings are stationary. The brake disc is keyed to the wheel. It is free to move laterally in the key slots. This is known as a floating disc. When the brakes are applied, the pistons move out from the outboard cylinders and their pucks contact the disc. The disc Figure 13-79. A single disc brake is a floating-disc, fixed caliper brake. 13-42 O-ring packing Lining puck O-ring gasket Bleed valve Brake disc Cylinder head Gasket Washer Brake housing Pin grip Washer Bleed screw Adjusting pin nut Bleeder adapter Internal retainer ring Spring guide Gasket Screw O-ring packing Gasket Fluid inlet bushing Piston Adjusting pin Brake return spring Figure 13-80. An exploded view of a single-disc brake assembly found on a light aircraft. Fluid inlet port Wheel Key Piston Disk Return spring Automatic adjusting pin or wear indicator pin Pressure plate lining puck Backing plate lining puck Adjusting pin grip Brake housing Figure 13-81. A cross-sectional view of a Goodyear single-disc brake caliper illustrates the adjusting pin assembly that doubles as a wear indicator. 13-43 slides slightly in the key slots until the inboard stationary pucks also contact the disc. The result is a fairly even amount of friction applied to each side of the disc and thus, the rotating motion is slowed. When brake pressure is released, the return spring in each piston assembly forces the piston back away from the disc. The spring provides a preset clearance between each puck and the disc. The self-adjusting feature of the brake maintains the same clearance, regardless of the amount of wear on the brake pucks. The adjusting pin on the back of each piston moves with the piston through a frictional pin grip. When brake pressure is relieved, the force of the return spring is sufficient to move the piston back away from the brake disc, but not enough to move the adjusting pin held by the friction of the pin grip. The piston stops when it contacts the head of the adjusting pin. Thus, regardless of the amount of wear, the same travel of the piston is required to apply the brake. The stem of the pin protruding through the cylinder head serves as a wear indicator. The manufacturer’s maintenance information states the minimum length of the pin that needs to be protruding for the brakes to be considered airworthy. [Figure 13-81] The brake caliper has the necessary passages machined into it to facilitate hydraulic fluid movement and the application of pressure when the brakes are utilized. The caliper housing also contains a bleed port used by the technician to remove unwanted air from the system. Brake bleeding, as it is known, should be done in accordance with the manufacturer’s maintenance instructions. Fixed-Disc Brakes Even pressure must be applied to both sides of the brake disc to generate the required friction and obtain consistent wear properties from the brake linings. The floating Fixed disc Brake lining ng Fixed disc disc accomplishes this as described above. It can also be accomplished by bolting the disc rigidly to the wheel and allowing the brake caliper and linings to float laterally when pressure is applied. This is the design of a common fixed-disc brake used on light aircraft. The brake is manufactured by the Cleveland Brake Company and is shown in Figure 13-82. An exploded detail view of the same type of brake is shown in Figure 13-83. The fixed-disc, floating-caliper design allows the brake caliper and linings to adjust position in relationship to the disc. Linings are riveted to the pressure plate and backplate. Two anchor bolts that pass through the pressure plate are secured to the cylinder assembly. The other ends of the bolts are free to slide in and out of bushings in the torque plate, which is bolted to the axle flange. The cylinder assembly is bolted to the backplate to secure the assembly around the disc. When pressure is applied, the caliper and linings center on the disc via the sliding action of the anchor bolts in the torque plate bushings. This provides equal pressure to both sides of the disc to slow its rotation. A unique feature of the Cleveland brake is that the linings can be replaced without removing the wheel. Unbolting the cylinder assembly from the backplate allows the anchor bolts to slide out of the torque plate bushings. The entire caliper assembly is then free and provides access to all of the components. Maintenance requirements on all single disc brake systems are similar to those on brake systems of any type. Regular inspection for any damage and for wear on the linings and discs is required. Replacement of parts worn beyond limits is always followed by an operational check. The check is performed while taxiing the aircraft. The braking action for each main wheel should be equal with equal application of pedal pressure. Pedals should be firm, not soft or spongy, Cylinder assembly Torque e plate Pressure plate Figure 13-82. A Cleveland brake on a light aircraft is a fixed-disc brake. It allows the brake caliper to move laterally on anchor bolts to deliver even pressure to each side of the brake disc. 13-44 Pressure plate Rivets (8) Backplate Torque plate Cylinder assembly Pistons Backplate lining Bushings Pressure plate lining Anchor bolts (2) Figure 13-83. An exploded view of a dual-piston Cleveland brake assembly. Housing assembly Wheel axle Bleeder screw Washer Spring Self-adjusting pin Piston Brake lining Brake disc Brake lining Center carrier Brake lining Brake disc Brake lining Housing back plate Figure 13-84. A dual-disc brake is similar to a single-disc brake. It uses a center carrier to hold brake linings against each of the discs. 13-45 when applied. When pedal pressure is released, the brakes should release without any evidence of drag. Dual-Disc Brakes Dual-disc brakes are used on aircraft where a single disc on each wheel does not supply sufficient braking friction. Two discs are keyed to the wheel instead of one. A center carrier is located between the two discs. It contains linings on each side that contact each of the discs when the brakes are applied. The caliper mounting bolts are long and mount through the center carrier, as well as the backplate which bolts to the housing assembly. [Figure 13-84] Multiple-Disc Brakes Large, heavy aircraft require the use of multiple-disc brakes. Multiple-disc brakes are heavy duty brakes designed for use with power brake control valves or power boost master cylinders, which is discussed later in this chapter. The brake assembly consists of an extended bearing carrier similar to a torque tube type unit that bolts to the axle flange. It supports the various brake parts, including an annular cylinder and piston, a series of steel discs alternating with copper or bronze-plated discs, a backplate, and a backplate retainer. The steel stators are keyed to the bearing carrier, and the copper or bronze plated rotors are keyed to the rotating wheel. Hydraulic pressure applied to the piston causes the entire stack of stators and rotors to be compressed. This creates enormous friction and heat and slows the rotation of the wheel. [Figure 13-85] As with the single and dual-disc brakes, retracting springs return the piston into the housing chamber of the bearing carrier when hydraulic pressure is relieved. The hydraulic fluid exits the brake to the return line through an automatic adjuster. The adjuster traps a predetermined amount of fluid in the brakes that is just sufficient to provide the correct clearances between the rotors and stators. [Figure 13-86] Brake wear is typically measured with a wear gauge that is not part of the brake assembly. These types of brake are typically found on older transport category aircraft. The rotors and stators are relatively thin, only about 1 »8-inch thick. They do not dissipate heat very well and have a tendency to warp. Segmented Rotor-Disc Brakes The large amount of heat generated while slowing the rotation of the wheels on large and high-performance aircraft is problematic. To better dissipate this heat, segmented rotordisc brakes have been developed. Segmented rotor-disc brakes are multiple-disc brakes but of more modern design than the type discussed earlier. There are many variations. Most feature numerous elements that aid in the control Automatic adjuster O-ring Bleeder valve (typical) Bearing carrier and sleeve Retractor spring assembly (typical 10 places) Felt wiper ring Felt wiper ring O-ring Backup ring Annular piston Retractor plate Nut (typical 10 places) Flinger ring Bearing retaining nut Snap ring Pressure plate and Insulator Rotor Stator Rotor Stator Rotor Stator Rotor Backup plate Backup plate retainer Locking piece Figure 13-85. A multiple disc brake with bearing carrier upon which the parts of the brake are assembled including an annular cylinder and piston assembly that apply pressure evenly to a stack of rotors and stators. and dissipation of heat. Segmented rotor-disc brakes are heavy-duty brakes especially adapted for use with the high pressure hydraulic systems of power brake systems. Braking is accomplished by means of several sets of stationary, high friction type brake linings that make contact with rotating segments. The rotors are constructed with slots or in sections with space between them, which helps dissipate heat and give the brake its name. Segmented rotor multiple-disc brakes are the standard brake used on high performance and air carrier aircraft. An exploded view of one type of segmented rotor brake assembly is shown in Figure 13-87. The description of a segmented rotor brake is very similar to the multiple-disc type brake previously described. The brake 13-46 Rotor (typical four places) Retainer Valve stem Packing To shuttle e valve valvve Retracting plate Automaticc adjuster Backup plate retainer B Felt wiperr Stator (typical three places) Landing gear strut Retracting springs (ten each) Pressure plate Packing Wheel bearing Brake pressure Return Pressure due to retracting springs Compensator piston Annulax piston Retainer Wheel bearing Bearing retaining nut Check valve C Brake Applied Brake Released Figure 13-86. A multiple-disc brake with details of the automatic adjuster. assembly consists of a carrier, a piston and piston cup seal, a pressure plate, an auxiliary stator plate, rotor segments, stator plates, automatic adjusters, and a backing plate. The carrier assembly, or brake housing with torque tube, is the basic unit of the segmented rotor brake. It is the part that attaches to the landing gear shock strut flange upon which the other components of the brake are assembled. On some brakes, two grooves or cylinders are machined into the carrier to receive the piston cups and pistons. [Figure 13-87] Most segmented rotor-disc brakes have numerous individual cylinders machined into the brake housing into which fit the same number of actuating pistons. Often, these cylinders are supplied by two different hydraulic sources, alternating every other cylinder from a single source. If one source fails, the brake still operates sufficiently on the other. [Figure 13-88] External fittings in the carrier or brake housing admit the hydraulic fluid. A bleed port can also be found. A pressure plate is a flat, circular, high-strength steel, nonrotating plate notched on the inside circumference to fit over the stator drive sleeves or torque tube spines. The brake 13-47 Piston return spring Adjuster pin nut Adjuster pin sleeve Adjuster clamp Carrier assembly Piston cup Brake assembly pin Adjuster pin Drive sleeve bolts Rotor assembly Stator plate Rotor assembly Torque tube Stator plate Piston Rotor assembly Pressure plate Backing plate Stator drive sleeve Brake lining disk (typical) Figure 13-87. Exploded and detail views of segmented rotor brakes. actuating pistons contact the pressure plate. Typically, an insulator is used between the piston head and the pressure plate to impede heat conduction from the brake discs. The pressure plate transfers the motion of the pistons to the stack of rotors and stators that compress to slow the rotation of the wheels. On most designs, brake lining material attached directly to the pressure plate contacts the first rotor in the stack to transfer the motion of the piston(s). [Figure 13-87] An auxiliary stator plate with brake lining material on the side opposite the pressure plate can also be used. Figure 13-88. Many modern segmented rotor disc brakes use a Any number of alternating rotors and stators are sandwiched under hydraulic pressure against the backing plate of the brake assembly when the brakes are applied. The backing plate is a heavy steel plate bolted to the housing or torque tube at a fixed dimension from the carrier housing. In most cases, it has brake lining material attached to it and contacts the last rotor in the stack. [Figure 13-87] housing machined to fit numerous individual actuating pistons. Stators are flat plates notched on the internal circumference 13-48 to be held stationary by the torque tube spines. They have wearable brake lining material riveted or adhered to each side to make contact with adjacent rotors. The liner is typically constructed of numerous isolated blocks. [Figure 13-87] The space between the liner blocks aids in the dissipation of heat. The composition of the lining materials vary. Steel is often used. Rotors are slit or segmented discs that have notches or tangs in the external circumference that key to the rotating wheel. Slots or spaces between sections of the rotor create segments that allow heat to dissipate faster than it would if the rotor was solid. They also allow for expansion and prevent warping. [Figure 13-87] Rotors are usually steel to which a frictional surface is bonded to both sides. Typically, sintered metal is used in creating the rotor contact surface. Segmented multiple-disc brakes use retraction spring assemblies with auto clearance adjusters to pull the backplate away from the rotor and stator stack when brake pressure is removed. This provides clearance so the wheel can turn unimpeded by contact friction between the brake parts but keeps the units in close proximity for rapid contact and braking when the brakes are applied. The number of retraction devices varies with brake design. Figure 13-89 illustrates a brake assembly used on a Boeing 737 transport category aircraft. In the cutaway view, the number and locations of the auto adjustment retraction mechanisms can be seen. Details of the mechanisms are also shown. Instead of using a pin grip assembly for auto adjustment, an adjuster pin, ball, and tube operate in the same manner. They move out when brake pressure is applied, but the ball in the tube limits the amount of the return to that equal to the brake lining wear. Two independent wear indicators are used on the brake illustrated. An indicator pin attached to the backplate protrudes through the carrier. The amount that it protrudes with the brakes applied is measured to ascertain if new linings are required. Note: Other segmented multiple-disc brakes may use slightly different techniques for pressure plate retraction and wear indication. Consult the manufacturer’s maintenance information to ensure wear indicators are read correctly. Carbon Brakes The segmented multiple-disc brake has given many years of reliable service to the aviation industry. It has evolved through time in an effort to make it lightweight and to dissipate the frictional heat of braking in a quick, safe manner. The latest iteration of the multiple-disc brake is the carbon-disc brake. It is currently found on high performance and air carrier aircraft. Carbon brakes are so named because carbon fiber materials are used to construct the brake rotors. [Figure 13-90] Carbon brakes are approximately forty percent lighter than conventional brakes. On a large transport category aircraft, this alone can save several hundred pounds in aircraft weight. The carbon fiber discs are noticeably thicker than sintered steel rotors but are extremely light. They are able to withstand temperatures fifty percent higher than steel component brakes. The maximum designed operating temperature is limited by the ability of adjacent components to withstand the high temperature. Carbon brakes have been shown to withstand two to three times the heat of a steel brake in nonaircraft applications. Carbon rotors also dissipate heat faster than steel rotors. A carbon rotor maintains its strength and dimensions at high temperatures. Moreover, carbon brakes last twenty to fifty percent longer than steel brakes, which results in reduced maintenance. The only impediment to carbon brakes being used on all aircraft is the high cost of manufacturing. The price is expected to lower as technology improves and greater numbers of aircraft operators enter the market. Expander Tube Brakes An expander tube brake is a different approach to braking that is used on aircraft of all sizes produced in the 1930s–1950s. It is a lightweight, low pressure brake bolted to the axle flange that fits inside an iron brake drum. A flat, fabric-reinforced neoprene tube is fitted around the circumference of a wheellike torque flange. The exposed flat surface of the expander tube is lined with brake blocks similar to brake lining material. Two flat frames bolt to the sides of the torque flange. Tabs on the frames contain the tube and allow evenly spaced torque bars to be bolted in place across the tube between each brake block. These prevent circumferential movement of the tube on the flange. [Figure 13-91] The expander tube is fitted with a metal nozzle on the inner surface. Hydraulic fluid under pressure is directed through this fitting into the inside of the tube when the brakes are applied. The tube expands outward, and the brake blocks make contact with the wheel drum causing friction that slows the wheel. As hydraulic pressure is increased, greater friction develops. Semi-elliptical springs located under the torque bars return the expander tube to a flat position around the flange when hydraulic pressure is removed. The clearance between the expander tube and the brake drum is adjustable by rotating an adjuster on some expander tube brakes. Consult the manufacturer’s maintenance manual for the correct clearance setting. Figure 13-92 gives an exploded view of an expander tube brake, detailing its components. Expander tube brakes work well but have some drawbacks. 13-49 Torque tube backing plate Reline indicator pin (2 places) see detail B Rotor (3 places) Pressure port Stator (4 places) Piston (6 places) Bleeder valves Torque tube Carrier Rivet (typical) Pressure cylinder (6 places) Lining block (14 places) surface Automatic adjuster assembly (4 places) see detail A Retaining ring Recessed washer Pressure plate Return spring Mounting flange Reverse bleed or drain plug Adjuster ball Carrier Tube L Return spring Carrier Housing Keywasher Adjuster pin Reline indicator pin Retainer Retaining pin Sleeve nut Pressure plate Pin retainer Rivet DETAIL A DETAIL B Figure 13-89. The multiple-disc brake assembly and details from a Boeing 737. They tend to take a setback when cold. They also have a tendency to swell with temperature and leak. They may drag inside the drum if this occurs. Eventually, expander brakes were abandoned in favor of disc brake systems. Brake Actuating Systems The various brake assemblies, described in the previous section, all use hydraulic power to operate. Different means of delivering the required hydraulic fluid pressure to brake assemblies are discussed in this section. There are three basic actuating systems: 13-50 Systems on different aircraft vary, but the general operation is similar to those described. Independent Master Cylinders In general, small, light aircraft and aircraft without hydraulic systems use independent braking systems. An independent brake system is not connected in any way to the aircraft hydraulic system. Master cylinders are used to develop the necessary hydraulic pressure to operate the brakes. This is similar to the brake system of an automobile. Figure 13-90. A carbon brake for a Boeing 737. Brake block Torque bar Frame Torque bar bolt Brake frame bolt Adjuster Brake spider Figure 13-91. An expander tube brake assembly. 1. An independent system not part of the aircraft main hydraulic system; 2. A booster system that uses the aircraft hydraulic system intermittently when needed; and 3. A power brake system that only uses the aircraft main. hydraulic system(s) as a source of pressure. In most brake actuating systems, the pilot pushes on the tops of the rudder pedals to apply the brakes. A master cylinder for each brake is mechanically connected to the corresponding rudder pedal (i.e., right main brake to the right rudder pedal, left main brake to the left rudder pedal). [Figure 13-93] When the pedal is depressed, a piston inside a sealed fluidfilled chamber in the master cylinder forces hydraulic fluid through a line to the piston(s) in the brake assembly. The brake piston(s) push the brake linings against the brake rotor to create the friction that slows the wheel rotation. Pressure is increased throughout the entire brake systems and against the rotor as the pedal is pushed harder. Many master cylinders have built-in reservoirs for the brake hydraulic fluid. Others have a single remote reservoir that services both of the aircraft’s two master cylinders. [Figure 13-94] A few light aircraft with nose wheel steering have only one master cylinder that actuates both main wheel brakes. This is possible because steering the aircraft during taxi does not require differential braking. Regardless of the set-up, it is the master cylinder that builds up the pressure required for braking. A master cylinder used with a remote reservoir is illustrated in Figure 13-95. This particular model is a Goodyear master cylinder. The cylinder is always filled with air-free, contaminant-free hydraulic fluid as is the reservoir and the line that connects the two together. When the top of the rudder pedal is depressed, the piston arm is mechanically moved forward into the master cylinder. It pushes the piston against the fluid, which is forced through the line to the brake. When pedal pressure is released, the return springs in the brake assembly retract the brake pistons back into the brake housing. The hydraulic fluid behind the pistons is displaced and must return to the master cylinder. As it does, a return spring in the master cylinder move the piston, piston rod and rudder pedal back to the original position (brake off, pedal not depressed). The fluid behind the master cylinder piston flows back into the reservoir. The brake is ready to be applied again. Hydraulic fluid expands as temperature increases. Trapped 13-51 Torque bar Return spring Brake block Return spring shield Expander tube Tube shield Torque bar bolt Frame Torque flange Frame Nozzle Adjuster Adjuster bolt Brake frame bolt Figure 13-92. An exploded view of an expander tube brake. Rudder pedals Brake master cylinders (2) Figure 13-93. Master cylinders on an independent brake system are directly connected to the rudder pedals or are connected through mechanical linkage. fluid can cause a brake to drag against the rotor(s). Leaks may also result. When the brakes are not applied, fluid must be allowed to expand safely without causing these issues. A compensating port is included in most master cylinders to facilitate this. In the master cylinder in Figure 13-95, this port is opened when the piston is fully retracted. Fluid in the brake system is allowed to expand into the reservoir, which has the capacity to accept the extra fluid volume. The typical reservoir is also vented to the atmosphere to provide positive pressure on the fluid. The forward side of the piston head contains a seal that closes off the compensating port when the brakes are applied so that pressure can build. The seal is only effective in the forward 13-52 is relieved by a spring in the mechanical linkage. Brake pedals Reservoir A common requirement of all braking systems is for there to be no air mixed in with the hydraulic fluid. Since air is compressible and hydraulic fluid essentially is not, any air under pressure when the brakes are applied causes spongy brakes. The pedals do not feel firm when pushed down due to the air compressing. Brake systems must be bled to remove all air from the system. Instructions for bleeding the brakes are in the manufacturer’s maintenance information. Brake systems equipped with Goodyear master cylinders must be bled from the top down to ensure any air trapped behind the master cylinder piston is removed. Master cylinders Parking brake valves (operated by a single control handle Left brake assembly Right brake assembly Figure 13-94. A remote reservoir services both master cylinders on some independent braking systems. direction. When the piston is returning, or is fully retracted to the off position, fluid behind the piston is free to flow through piston head ports to replenish any fluid that may be lost downstream of the master cylinder. The aft end of the master cylinder contains a seal that prevents leakage at all times. A rubber boot fits over the piston rod and the aft end of the master cylinder to keep out dust. A parking brake for this remote reservoir master cylinder brake system is a ratcheting mechanical device between the master cylinder and the rudder pedals. With the brakes applied, the ratchet is engaged by pulling the parking brake handle. To release the brakes, the rudder pedals are depressed further allowing the ratchet to disengage. With the parking brake set, any expansion of hydraulic fluid due to temperature An alternative common arrangement of independent braking systems incorporates two master cylinders, each with its own integral fluid reservoir. Except for the reservoir location, the brake system is basically the same as just described. The master cylinders are mechanically linked to the rudder pedals as before. Depressing the top of a pedal causes the piston rod to push the piston into the cylinder forcing the fluid out to the brake assembly. The piston rod rides in a compensator sleeve and contains an O-ring that seals the rod to the piston when the rod is moved forward. This blocks the compensating ports. When released, a spring returns the piston to its original position which refills the reservoir as it returns. The rod end seal retracts away from the piston head allowing a free flow of fluid from the cylinder through the compensating ports in the piston to the reservoir. [Figure 13-96] The parking brake mechanism is a ratcheting type that operates as described. A servicing port is supplied at the top of the master cylinder reservoir. Typically, a vented plug is installed in the port to provide positive pressure on the fluid. Piston head ports Boot Inlet from reservoir Piston rod Compensating port Seal Piston Seal To brake Return spring Figure 13-95. A Goodyear brake master cylinder from an independent braking system with a remote reservoir. 13-53 A Compensator port Toggle Spool valve To system return manifold To system pressure manifold Poppet Compensator port Piston B To brake wheel cylinder Piston rod Compensator sleeve Piston rod to brake pedal Brakes on O-ring and washer Figure 13-97. A master cylinder for a boosted brake system augments foot pedal pressure with aircraft system hydraulic pressure during heavy braking. attached to the rudder pedals. However, the boosted brake master cylinder operates differently. [Figure 13-97] Figure 13-96. A common master cylinder with built-in reservoir is shown. Illustration A depicts the master cylinder when the brakes are off. The compensating port is open to allow fluid to expand into the reservoir should temperature increase. In B, the brakes are applied. The piston rod-end seal covers the compensating port as it contacts the piston head. Boosted Brakes In an independent braking system, the pressure applied to the brakes is only as great as the foot pressure applied to the top of the rudder pedal. Boosted brake actuating systems augment the force developed by the pilot with hydraulic system pressure when needed. The boost is only during heavy braking. It results in greater pressure applied to the brakes than the pilot alone can provide. Boosted brakes are used on medium and larger aircraft that do not require a full power brake actuating system. When the brakes are applied, the pressure from the pilot’s foot through the mechanical linkage moves the master cylinder piston in the direction to force fluid to the brakes. The initial movement closes the compensator poppet used to provide thermal expansion relief when the brakes are not applied. As the pilot pushes harder on the pedal, a spring-loaded toggle moves a spool valve in the cylinder. Aircraft hydraulic system pressure flows through the valve to the back side of the piston. Pressure is increased, as is the force developed to apply the brakes. When the pedal is released, the piston rod travels in the opposite direction, and the piston returns to the piston stop. The compensating poppet reopens. The toggle is withdrawn from the spool via linkages, and fluid pushes the spool back to expose the system return manifold port. System hydraulic fluid used to boost brake pressure returns through the port. A boosted brake master cylinder for each brake is mechanically 13-54 A Pilot’s pedals Autobrake pressure control Shuttle valve Fuse Normal brake metering valve Normal anti-skid valves Alternate brake metering valve Alternate antiskid valve Shuttle valve Hydraulic system pressure B Brake check valve Power brake control valves Brake accumulator Emergency brake valve Antiskid control valves Shuttle valves Brake assemblies Air/oil transfer tube Emergency IR cylinder Accumulator air pressure Return Hydraulic pressure Figure 13-98. The orientation of components in a basic power brake system is shown in A. The general layout of an airliner power brake system is shown in B. Power Brakes Large and high-performance aircraft are equipped with power brakes to slow, stop, and hold the aircraft. Power brake actuating systems use the aircraft hydraulic system as the source of power to apply the brakes. The pilot presses on the top of the rudder pedal for braking as with the other actuating systems. The volume and pressure of hydraulic fluid required cannot be produced by a master cylinder. Instead, a power brake control valve or brake metering valve receives the brake pedal input either directly or through linkages. 13-55 To inboard brake To return Brake metering valve sleeve Brake metering valve slide “A” system pressure Autobrake piston Valve body Autobrake Compensating chamber Crank stop Springseat Return spring “B” system pressure Link assembly To outboard brake To return Brake input shaft Crank Figure 13-99. A brake metering valve from a Boeing 737. A machined slide or spool moves laterally to admit the correct amount of hydraulic system fluid to the brakes. The pressure developed is in proportion to the amount the rudder/brake pedal is depressed and the amount the slide is displaced. The slide/spool also simultaneously controls the return of fluid to the hydraulic system return manifold when brake pressure is released. The valve meters hydraulic fluid to the corresponding brake assembly in direct relation to the pressure applied to the pedal. an emergency brake power source. An airliner power brake system is illustrated in Figure 13-98B. Many power brake system designs are in use. Most are similar to the simplified system illustrated in Figure 13-98A. Power brake systems are constructed to facilitate graduated brake pressure control, brake pedal feel, and the necessary redundancy required in case of hydraulic system failure. Large aircraft brake systems integrate anti-skid detection and correction devices. These are necessary because wheel skid is difficult to detect on the flight deck without sensors. However, a skid can be quickly controlled automatically through pressure control of the hydraulic fluid to the brakes. Hydraulic fuses are also commonly found in power brake systems. The hostile environment around the landing gear increases the potential for a line to break or sever, a fitting to fail, or other hydraulic system malfunctions to occur where hydraulic fluid is lost en route to the brake assemblies. A fuse stops any excessive flow of fluid when detected by closing to retain the remaining fluid in the hydraulic system. Shuttle valves are used to direct flow from optional sources of fluid, such as in redundant systems or during the use of Brake Control Valve/Brake Metering Valve The key element in a power brake system is the brake control valve, sometimes called a brake metering valve. It responds to brake pedal input by directing aircraft system hydraulic fluid to the brakes. As pressure is increased on the brake pedal, more fluid is directed to the brake causing a higher pressure and greater braking action. A brake metering valve from a Boeing 737 is illustrated in Figure 13-99. The system in which it is installed is diagramed in Figure 13-100. Two sources of hydraulic pressure provide redundancy in this brake system. A brake input shaft, connected to the rudder/brake pedal through mechanical linkages, provides the position input to the metering valve. As in most brake control valves, the brake input shaft moves a tapered spool or slide in the valve so that it allows hydraulic system pressure to flow to the brakes. At the same time, the slide covers and uncovers access to the hydraulic system return port as required. 13-56 System return System pressure Brake pressure Ground interconnect valve M “B” system pressure “A” system pressure To system return System “B” solenoid valve 132 Charging valve Main landing gear “UP” line Automatic wheel snubbing Accumulator 132 System “A” solenoid valve Brake valve feel augmentation actuator 2 3 1 3 2 1 Brakes ON Parking brake shutoff valve M Transmitter Gauge Brake metering valve Shuttle valve Antiskid valve Sealing disconnect fitting (type) Outboard wheel Swivel (type) Pressure comparator Pressure comparator Inboard wheel Left gear Right gear Figure 13-100. The power brake system on a Boeing 737. When the rudder/brake pedal is depressed, the slide in the metering valve moves to the left. [Figure 13-99] It covers the return port so pressure can build in the brake system. The hydraulic supply pressure chamber is connected to the brake system pressure chamber by the movement of the slide, which due to its taper, unblocks the passage between these two. As the pedal is depressed further, the valve slide moves farther to the left. This enables more fluid to flow to the brakes due to the narrowing shape of the slide. Brake pressure increases with the additional fluid. A passage in the slide directs brake pressure fluid into a compensating chamber at the end of the slide. This acts on the end of the slide creating a return force that counters the initial slide movement and gives feel to the brake pedal. As a result, the pressure and return ports are closed and pressure proportional to the foot pressure on the pedal is held on the brakes. When the pedal is released, a return spring and compensating chamber pressure drive the slide to the right into its original position (return port open, supply pressure chamber and brake pressure chambers blocked from each other). The metering valve operates as described simultaneously for the inboard and the outboard brakes. [Figure 13-99] The design of the link assembly is such that a single side of the metering valve can operate even if the other fails. Most brake control valves and metering valves function in a similar manner, although many are single units that supply only one brake assembly. The auto brake, referenced in the metering valve diagram, is connected into the landing gear retraction hydraulic line. Pressurized fluid enters this port and drives the slide slightly to the left to apply the brakes automatically after takeoff. This stops the wheels from rotating when retracted into the wheel wells. Auto brake pressure is withheld from this port when the landing gear is fully stowed since the retraction system is depressurized. 13-57 The majority of the rudder/brake pedal feel is supplied by the brake control or brake metering valve in a power brake system. Many aircraft refine the feel of the pedal with an additional feel unit. The brake valve feel augmentation unit, in the above system, uses a series of internal springs and pistons of various sizes to create a force on the brake input shaft movement. This provides feel back through the mechanical linkages consistent with the amount of rudder/ brake pedal applied. The request for light braking with slight pedal depression results in a light feel to the pedal and a harder resistance feel when the pedals are pushed harder during heavy braking. [Figure 13-101] Emergency Brake Systems As can be seen in Figure 13-100, the brake metering valves not only receive hydraulic pressure from two separate hydraulic systems, they also feed two separate brake assemblies. Each main wheel assembly has two wheels. The inboard wheel brake and the outboard wheel brake, located in their respective wheel rims, are independent from each other. In case of hydraulic system failure or brake failure, each is independently supplied to adequately slow and stop the aircraft without the other. More complicated aircraft may involve another hydraulic system for back-up or use a similar alternation of sources and brake assemblies to maintain braking in case of hydraulic system or brake failure. Note: In the segmented rotor brake section above, a brake assembly was described that had alternating pistons supplied Port No. 3 system “A” pressure Brake metering valve shaft by independent hydraulic sources. This is another method of redundancy particularly suitable on, but not limited to, single main wheel aircraft. In addition to supply system redundancy, the brake accumulator is also an emergency source of power for the brakes in many power brake systems. The accumulator is pre-charged with air or nitrogen on one side of its internal diaphragm. Enough hydraulic fluid is contained on the other side of the diaphragm to operate the brakes in case of an emergency. It is forced out of the accumulator into the brakes through the system lines under enough stored pressure to slow the aircraft. Typically, the accumulator is located upstream of the brake control/metering valve to capitalize on the control given by the valve. [Figure 13-102] Some simpler power brake systems may use an emergency source of brake power that is delivered directly to the brake assemblies and bypasses the remainder of the brake system completely. A shuttle valve immediately upstream of the brake units shifts to accept this source when pressure is lost from the primary supply sources. Compressed air or nitrogen is sometimes used. A pre-charged fluid source can also be used as an alternate hydraulic source. Parking Brake The parking brake system function is a combined operation. The brakes are applied with the rudder pedals and a ratcheting system holds them in place when the parking Port No. 1 brake pressure Spring-loaded check valve Spring Crank arm Port No. 2 to return Pressure regulator Slide assembly Roller Piston Bleed port Piston assembly Figure 13-101. The power brake system on a Boeing 737. 13-58 System A brake accumulator System B brake accumulator Brake pressure gauge and accumulator air charging valve UP D FW Nitrogen g charging g g valve Accumulator pre-charge pressure gauge Parking brake lever Figure 13-103. The parking brake lever on a Boeing 737 center pedestal throttle quadrant. Brake accumulator Figure 13-102. Emergency brake hydraulic fluid accumulators are precharged with nitrogen to deliver brake fluid to the brakes in the event normal and alternate hydraulic sources fail. brake lever on the flight deck is pulled. [Figure 13-103] At the same time, a shut-off valve is closed in the common return line from the brakes to the hydraulic system. This traps the fluid in the brakes holding the rotors stationary. Depressing the pedals further releases the pedal ratchet and opens the return line valve. Brake Deboosters Some aircraft brake assemblies that operate on aircraft hydraulic system pressure are not designed for such high pressure. They provide effective braking through a power brake system but require less than maximum hydraulic system pressure. To supply the lower pressure, a brake debooster cylinder is installed downstream of the control valve and anti-skid valve. [Figure 13-104] The debooster reduces some pressure from the control valve to within the working range of the brake assembly. Brake deboosters are simple devices that use the application of force over different sized pistons to reduce pressure. [Figure 13-105] Their operation can be understood through the application of the following equation: Pressure = Force/Area High-pressure hydraulic system input pressure acts on the small end of a piston. This develops a force proportional to the area of the piston head. The other end of the piston is larger and housed in a separate cylinder. The force from the smaller piston head is transferred to the larger area of the other end of the piston. The amount of pressure conveyed by the larger end of the piston is reduced due to the greater area over which the force is spread. The volume of output fluid increases since a larger piston and cylinder are used. The reduced pressure is delivered to the brake assembly. The spring in the debooster aids in returning the piston to the ready position. If fluid is lost downstream of the deboost cylinder, the piston travels further down into the cylinder when the brakes are applied. The pin unseats the ball and allows fluid into the lower cylinder to replace what was lost. Once replenished, the piston rises up in the cylinder due to pressure build-up. The ball reseats as the piston travels above the pin and normal braking resumes. This function is not meant to permit leaks in the brake assemblies. Any leak discovered must be repaired by the technician. A lockout debooster functions as a debooster and a hydraulic fuse. If fluid is not encountered as the piston moves down in the cylinder, the flow of fluid to the brakes is stopped. This prevents the loss of all system hydraulic fluid should a rupture downstream of the debooster occur. Lockout deboosters have a handle to reset the device after it closes as a fuse. If not reset, no braking action is possible. 13-59