Aircraft Landing Gear Systems Chapter 13 PDF
Document Details
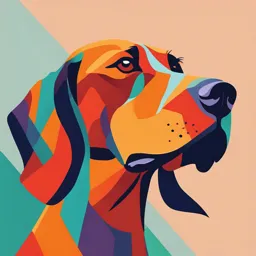
Uploaded by DaringDream
Tags
Summary
This chapter details the different types of aircraft landing gear, including tail wheel, tandem, and tricycle types. It also describes the components and functions of each type. The text includes diagrams to illustrate the various configurations.
Full Transcript
Chapter 13 Aircraft Landing Gear Systems Landing Gear Types Aircraft landing gear supports the entire weight of an aircraft during landing and ground operations. They are attached to primary structural members of the aircraft. The type of gear depends on the aircraft design and its intended use. Mos...
Chapter 13 Aircraft Landing Gear Systems Landing Gear Types Aircraft landing gear supports the entire weight of an aircraft during landing and ground operations. They are attached to primary structural members of the aircraft. The type of gear depends on the aircraft design and its intended use. Most landing gear have wheels to facilitate operation to and from hard surfaces, such as airport runways. Other gear feature skids for this purpose, such as those found on helicopters, balloon gondolas, and in the tail area of some tail dragger aircraft. Aircraft that operate to and from frozen lakes and snowy areas may be equipped with landing gear that have skis. Aircraft that operate to and from the surface of water have pontoon-type landing gear. Regardless of the type of landing gear utilized, shock absorbing equipment, brakes, retraction mechanisms, controls, warning devices, cowling, fairings, and structural members necessary to attach the gear to the aircraft are considered parts of the landing gear system. [Figure 13-1] Numerous configurations of landing gear types can be found. Additionally, combinations of two types of gear are common. Amphibious aircraft are designed with gear that allow landings to be made on water or dry land. The gear features pontoons for water landing with extendable wheels for landings on hard surfaces. A similar system is used to allow the use of skis and wheels on aircraft that operate on both slippery, frozen surfaces and dry runways. Typically, the skis are retractable to allow use of the wheels when needed. Figure 13-2 illustrates this type of landing gear. Note: References to auxiliary landing gear refer to the nose gear, tail gear, or outrigger-type gear on any particular aircraft. Main landing gear are the two or more large gear located close to the aircraft’s center of gravity. Figure 13-1. Basic landing gear types include those with wheels (a), skids (b), skis (c), and floats or pontoons (d). 13-1 Figure 13-2. An amphibious aircraft with retractable wheels (left) and an aircraft with retractable skis (right). Figure 13-3. Tail wheel configuration landing gear on a DC-3 (left) and a STOL Maule MX-7-235 Super Rocket. Landing Gear Arrangement Three basic arrangements of landing gear are used: tail wheel-type landing gear (also known as conventional gear), tandem landing gear, and tricycle-type landing gear. Tail Wheel-Type Landing Gear Tail wheel-type landing gear is also known as conventional gear because many early aircraft use this type of arrangement. The main gear are located forward of the center of gravity, causing the tail to require support from a third wheel assembly. A few early aircraft designs use a skid rather than a tail wheel. This helps slow the aircraft upon landing and provides directional stability. The resulting angle of the aircraft fuselage, when fitted with conventional gear, allows the use of a long propeller that compensates for older, underpowered engine design. The increased clearance of the forward fuselage offered by tail wheel-type landing gear is also advantageous when operating in and out of non-paved runways. Today, aircraft are manufactured with conventional gear for this reason and for the weight savings accompanying the relatively light tail wheel assembly. [Figure 13-3] The proliferation of hard surface runways has rendered the tail skid obsolete in favor of the tail wheel. Directional control is maintained through differential braking until the speed of the aircraft enables control with the rudder. A steerable tail wheel, connected by cables to the rudder or rudder pedals, is also a common design. Springs are incorporated for dampening. [Figure 13-4] Tandem Landing Gear Few aircraft are designed with tandem landing gear. As the name implies, this type of landing gear has the main gear and tail gear aligned on the longitudinal axis of the aircraft. Sailplanes commonly use tandem gear, although many only have one actual gear forward on the fuselage with a skid under the tail. A few military bombers, such as the B-47 and the B-52, have tandem gear, as does the U2 spy plane. The VTOL Harrier has tandem gear but uses small outrigger gear under the wings for support. Generally, placing the gear only under the fuselage facilitates the use of very flexible wings. [Figure 13-5] 13-2 Figure 13-4. The steerable tail wheel of a Pitts Special. Figure 13-5. Tandem landing gear along the longitudinal axis of the aircraft permits the use of flexible wings on sailplanes (left) and select military aircraft like the B-52 (center). The VTOL Harrier (right) has tandem gear with outrigger-type gear. Figure 13-6. Tricycle-type landing gear with dual main wheels on a Learjet (left) and a Cessna 172, also with tricycle gear (right). Tricycle-Type Landing Gear The most commonly used landing gear arrangement is the tricycle-type landing gear. It is comprised of main gear and nose gear. [Figure 13-6] Tricycle-type landing gear is used on large and small aircraft with the following benefits: 1. Allows more forceful application of the brakes without nosing over when braking, which enables higher landing speeds. 2. Provides better visibility from the flight deck, especially during landing and ground maneuvering. 3. Prevents ground-looping of the aircraft. Since the aircraft center of gravity is forward of the main gear, forces acting on the center of gravity tend to keep the aircraft moving forward rather than looping, such as with a tail wheel-type landing gear. The nose gear of a few aircraft with tricycle-type landing gear is not controllable. It simply casters as steering is accomplished with differential braking during taxi. However, nearly all aircraft have steerable nose gear. On light aircraft, 13-3 Figure 13-9. Triple bogie main landing gear assembly on a Boeing 777. 0 R Figure 13-7. A nose wheel steering tiller located on the flight deck. the nose gear is directed through mechanical linkage to the rudder pedals. Heavy aircraft typically utilize hydraulic power to steer the nose gear. Control is achieved through an independent tiller in the flight deck. [Figure 13-7] The main gear on a tricycle-type landing gear arrangement is attached to reinforced wing structure or fuselage structure. The number and location of wheels on the main gear vary. Many main gear have two or more wheels. [Figure 13-8] Multiple wheels spread the weight of the aircraft over a larger area. They also provide a safety margin should one tire fail. Heavy aircraft may use four or more wheel assemblies on each main gear. When more than two wheels are attached to a landing gear strut, the attaching mechanism is known as a bogie. The number of wheels included in the bogie is a function of the gross design weight of the aircraft and the surface type on which the loaded aircraft is required to land. Figure 13-9 illustrates the triple bogie main gear of a Boeing 777. The tricycle-type landing gear arrangement consists of many parts and assemblies. These include air/oil shock struts, gear alignment units, support units, retraction and safety devices, steering systems, wheel and brake assemblies, etc. A main landing gear of a transport category aircraft is illustrated in Figure 13-10 with many of the parts identified as an introduction to landing gear nomenclature. Figure 13-8. Dual main gear of a tricycle-type landing gear. Fixed & Retractable Landing Gear Further classification of aircraft landing gear can be made into two categories: fixed and retractable. Many small, singleengine light aircraft have fixed landing gear, as do a few light twins. This means the gear is attached to the airframe and remains exposed to the slipstream as the aircraft is flown. As discussed in Chapter 2 of this handbook, as the speed of 13-4 Beam hanger Walking beam Downlock spring bungee Main gear actuator Downlock actuator Ground speed brake cable (right gear only) Reaction link Trunnion link Uplock actuator Drag strut Universal side strut fitting Uplock spring bungeer Downlock Side strut Shock strut Uplock roller Damper hydraulic line Gravel deflector Main gear damper Axle Torsion links FW D INB D Figure 13-10. Nomenclature of a main landing gear bogie truck. an aircraft increases, so does parasite drag. Mechanisms to retract and stow the landing gear to eliminate parasite drag add weight to the aircraft. On slow aircraft, the penalty of this added weight is not overcome by the reduction of drag, so fixed gear is used. As the speed of the aircraft increases, the drag caused by the landing gear becomes greater and a means to retract the gear to eliminate parasite drag is required, despite the weight of the mechanism. A great deal of the parasite drag caused by light aircraft landing gear can be reduced by building gear as aerodynamically as possible and by adding fairings or wheel pants to streamline the airflow past the protruding assemblies. A small, smooth profile to the oncoming wind greatly reduces landing gear parasite drag. Figure 13-11 illustrates a Cessna aircraft landing gear used on many of the manufacturer’s light planes. 13-5 Figure 13-11. Wheel fairings, or pants, and low profile struts reduce parasite drag on fixed gear aircraft. The thin cross section of the spring steel struts combine with the fairings over the wheel and brake assemblies to raise performance of the fixed landing gear by keeping parasite drag to a minimum. Retractable landing gear stow in fuselage or wing compartments while in flight. Once in these wheel wells, gear are out of the slipstream and do not cause parasite drag. Most retractable gear have a close-fitting panel attached to them that fairs with the aircraft skin when the gear is fully retracted. [Figure 13-12] Other aircraft have separate doors that open, allowing the gear to enter or leave, and then close again. Note: The parasite drag caused by extended landing gear can be used by the pilot to slow the aircraft. The extension and retraction of most landing gear is usually accomplished with hydraulics. Landing gear retraction systems are discussed later in this chapter. Figure 13-13. Non-shock absorbing struts made from steel, aluminum, or composite material transfer the impact forces of landing to the airframe at a non-damaging rate. Shock Absorbing & Non-Shock Absorbing Landing Gear In addition to supporting the aircraft for taxi, the forces of impact on an aircraft during landing must be controlled by the landing gear. This is done in two ways: 1) the shock energy is altered and transferred throughout the airframe at a different rate and time than the single strong pulse of impact, and 2) the shock is absorbed by converting the energy into heat energy. Leaf-Type Spring Gear Many aircraft utilize flexible spring steel, aluminum, or composite struts that receive the impact of landing and return it to the airframe to dissipate at a rate that is not harmful. The gear flexes initially and forces are transferred as it returns to its original position. [Figure 13-13] The most common example of this type of non-shock absorbing landing gear are the thousands of single-engine Cessna aircraft that use it. Landing gear struts of this type made from composite materials are lighter in weight with greater flexibility and do not corrode. Figure 13-12. The retractable gear of a Boeing 737 fair into recesses in the fuselage. Panels attached to the landing gear provide smooth airflow over the struts. The wheel assemblies mate with seals to provide aerodynamic flow without doors. 13-6 of many individual small strands of elastic rubber that must be inspected for condition. Solid, donut-type rubber cushions are also used on some aircraft landing gear. [Figure 13-15] Shock Struts True shock absorption occurs when the shock energy of landing impact is converted into heat energy, as in a shock strut landing gear. This is the most common method of landing shock dissipation in aviation. It is used on aircraft of all sizes. Shock struts are self-contained hydraulic units that support an aircraft while on the ground and protect the structure during landing. They must be inspected and serviced regularly to ensure proper operation. Figure 13-14. Rigid steel landing gear is used on many early aircraft. Rigid Before the development of curved spring steel landing struts, many early aircraft were designed with rigid, welded steel landing gear struts. Shock load transfer to the airframe is direct with this design. Use of pneumatic tires aids in softening the impact loads. [Figure 13-14] Modern aircraft that use skid-type landing gear make use of rigid landing gear with no significant ill effects. Rotorcraft, for example, typically experience low impact landings that are able to be directly absorbed by the airframe through the rigid gear (skids). Bungee Cord The use of bungee cords on non-shock absorbing landing gear is common. The geometry of the gear allows the strut assembly to flex upon landing impact. Bungee cords are positioned between the rigid airframe structure and the flexing gear assembly to take up the loads and return them to the airframe at a non-damaging rate. The bungees are made There are many different designs of shock struts, but most operate in a similar manner. The following discussion is general in nature. For information on the construction, operation, and servicing of a specific aircraft shock, consult the manufacturer’s maintenance instructions. A typical pneumatic/hydraulic shock strut uses compressed air or nitrogen combined with hydraulic fluid to absorb and dissipate shock loads. It is sometimes referred to as an air/oil or oleo strut. A shock strut is constructed of two telescoping cylinders or tubes that are closed on the external ends. The upper cylinder is fixed to the aircraft and does not move. The lower cylinder is called the piston and is free to slide in and out of the upper cylinder. Two chambers are formed. The lower chamber is always filled with hydraulic fluid and the upper chamber is filled with compressed air or nitrogen. An orifice located between the two cylinders provides a passage for the fluid from the bottom chamber to enter the top cylinder chamber when the strut is compressed. [Figure 13-16] Most shock struts employ a metering pin similar to that shown in Figure 13-16 for controlling the rate of fluid flow Figure 13-15. Piper Cub bungee cord landing gear transfer landing loads to the airframe (left and center). Rubber, donut-type shock transfer is used on some Mooney aircraft (right). 13-7 Servicing valve Outer cylinder Tapered metering pin Orifice plate Orifice Torque arms Inner cylinder (piston) Wheel axle Towing eye Figure 13-16. A landing gear shock strut with a metering pin to control the flow of hydraulic fluid from the lower chamber to the upper chamber during compression. from the lower chamber into the upper chamber. During the compression stroke, the rate of fluid flow is not constant. It is automatically controlled by the taper of the metering pin in the orifice. When a narrow portion of the pin is in the orifice, more fluid can pass to the upper chamber. As the diameter of the portion of the metering pin in the orifice increases, less fluid passes. Pressure build-up caused by strut compression and the hydraulic fluid being forced through the metered orifice causes heat. This heat is converted impact energy. It is dissipated through the structure of the strut. On some types of shock struts, a metering tube is used. The operational concept is the same as that in shock struts with metering pins, except the holes in the metering tube control the flow of fluid from the bottom chamber to the top chamber during compression. [Figure 13-17] 13-8 Air valve Centering cam Lower cylinder Metering tube Inner cylinder Piston rod Piston Axle Figure 13-18. Axles machined out of the same material as the landing gear lower cylinder. Figure 13-17. Some landing gear shock struts use an internal metering tube rather than a metering pin to control the flow of fluid from the bottom cylinder to the top cylinder. Upon lift off or rebound from compression, the shock strut tends to extend rapidly. This could result in a sharp impact at the end of the stroke and damage to the strut. It is typical for shock struts to be equipped with a damping or snubbing device to prevent this. A recoil valve on the piston or a recoil tube restricts the flow of fluid during the extension stroke, which slows the motion and prevents damaging impact forces. Most shock struts are equipped with an axle as part of the lower cylinder to provide installation of the aircraft wheels. Shock struts without an integral axle have provisions on the end of the lower cylinder for installation of the axle assembly. Suitable connections are provided on all shock strut upper cylinders to attach the strut to the airframe. [Figure 13-18] The upper cylinder of a shock strut typically contains a valve fitting assembly. It is located at or near the top of the cylinder. The valve provides a means of filling the strut with hydraulic fluid and inflating it with air or nitrogen as specified by the manufacturer. A packing gland is employed to seal the sliding joint between the upper and lower telescoping cylinders. It is installed in the open end of the outer cylinder. A packing gland wiper ring is also installed in a groove in the lower bearing or gland nut on most shock struts. It is designed to keep the sliding surface of the piston from carrying dirt, mud, ice, and snow into the packing gland and upper cylinder. Regular cleaning of the exposed portion of the strut piston helps the wiper do its job and decreases the possibility of damage to the packing gland, which could cause the strut to a leak. To keep the piston and wheels aligned, most shock struts are equipped with torque links or torque arms. One end of the links is attached to the fixed upper cylinder. The other end is attached to the lower cylinder (piston), so it cannot rotate. This keeps the wheels aligned. The links also retain the piston in the end of the upper cylinder when the strut is extended, such as after takeoff. [Figure 13-19] Nose gear shock struts are provided with a locating cam assembly to keep the gear aligned. A cam protrusion is attached to the lower cylinder, and a mating lower cam recess is attached to the upper cylinder. These cams line up 13-9 Cylinder Upper locating cam Lower locating cam Torque arm Torque links Piston Figure 13-19. Torque links align the landing gear and retain the piston in the upper cylinder when the strut is extended. the wheel and axle assembly in the straight-ahead position when the shock strut is fully extended. This allows the nose wheel to enter the wheel well when the nose gear is retracted and prevents structural damage to the aircraft. It also aligns the wheels with the longitudinal axis of the aircraft prior to landing when the strut is fully extended. [Figure 13-20] Many nose gear shock struts also have attachments for the installation of an external shimmy damper. [Figure 13-21] Nose gear struts are often equipped with a locking or disconnect pin to enable quick turning of the aircraft while towing or positioning the aircraft when on the ramp or in a hangar. Disengagement of this pin allows the wheel fork spindle on some aircraft to rotate 360°, thus enabling the aircraft to be turned in a tight radius. At no time should the nose wheel of any aircraft be rotated beyond limit lines marked on the airframe. Nose and main gear shock struts on many aircraft are also equipped with jacking points and towing lugs. Jacks should always be placed under the prescribed points. When towing lugs are provided, the towing bar should be attached only to these lugs. [Figure 13-22] Fork Axle Figure 13-20. An upper locating cam mates into a lower cam recess when the nose landing gear shock strut is extended before landing and before the gear is retracted into the wheel well. Shock struts contain an instruction plate that gives directions for filling the strut with fluid and for inflating the strut. The instruction plate is usually attached near filler inlet and air valve assembly. It specifies the correct type of hydraulic fluid to use in the strut and the pressure to which the strut should be inflated. It is of utmost importance to become familiar with these instructions prior to filling a shock strut with hydraulic fluid or inflating it with air or nitrogen. Shock Strut Operation Figure 13-23 illustrates the inner construction of a shock strut. Arrows show the movement of the fluid during compression and extension of the strut. The compression stroke of the shock strut begins as the aircraft wheels touch the ground. As 13-10 Shimmy damper Figure 13-21. A shimmy damper helps control oscillations of the nose gear. Compression Stroke UN LO CK LO CK Air Extension Stroke Hydraulic fluid Figure 13-23. Fluid flow during shock strut operation is controlled by the taper of the metering pin in the shock strut orifice. Figure 13-22. A towing lug on a landing gear is the designed means for attaching a tow bar. the center of mass of the aircraft moves downward, the strut compresses, and the lower cylinder or piston is forced upward into the upper cylinder. The metering pin is therefore moved up through the orifice. The taper of the pin controls the rate of fluid flow from the bottom cylinder to the top cylinder at all points during the compression stroke. In this manner, the greatest amount of heat is dissipated through the walls of the strut. At the end of the downward stroke, the compressed air in the upper cylinder is further compressed which limits the compression stroke of the strut with minimal impact. During taxi operations, the air in the tires and the strut combine to smooth out bumps. Insufficient fluid, or air in the strut, cause the compression stroke to not be properly limited. The strut could bottom out, resulting in impact forces to be transferred directly to the airframe through the metallic structure of the strut. In a properly serviced strut, the extension stroke of the shock strut operation occurs at the end of the compression stroke. Energy stored in the compressed air in the upper cylinder causes the aircraft to start moving upward in relation to the ground and lower strut cylinder as the strut tries to rebound to its normal position. Fluid is forced back down into the lower cylinder through restrictions and snubbing orifices. The snubbing of fluid flow during the extension stroke dampens the strut rebound and reduces oscillation caused by the spring action of the compressed air. A sleeve, spacer, or bumper ring incorporated into the strut limits the extension stroke. 13-11 normal ground operating position. Make certain that personnel, work stands, and other obstacles are clear of the aircraft. If the maintenance procedures require, securely jack the aircraft. Efficient operation of the shock struts requires that proper fluid and air pressure be maintained. To check the fluid level, most struts need to be deflated and compressed into the fully compressed position. Deflating a shock strut can be a dangerous operation. The technician must be thoroughly familiar with the operation of the high-pressure service valve found at the top of the strut’s upper cylinder. Refer to the manufacturer’s instructions for proper deflating technique of the strut in question and follow all necessary safety precautions. Two common types of high pressure strut servicing valves are illustrated in Figure 13-24. The AN6287-1 valve in Figure 13-24A has a valve core assembly and is rated to 3,000 pounds per square inch (psi). However, the core itself is only rated to 2,000 psi. The MS28889-1 valve in Figure 13-24B has no valve core. It is rated to 5,000 psi. The swivel nut on the AN6287-1 valve is smaller than the valve body hex. The MS28889-1 swivel nut is the same size as the valve body hex. The swivel nuts on both valves engage threads on an internal stem that loosens or draws tight the valve stem to a metal seat. Servicing Shock Struts The following procedures are typical of those used in deflating a shock strut, servicing it with hydraulic fluid, and re-inflating the strut. 1. 2. Remove the cap from the air servicing valve. [Figure 13-25A] 3. Check the swivel nut for tightness. 4. If the servicing valve is equipped with a valve core, depress it to release any air pressure that may be trapped under the core in the valve body. [Figure 13-25B] Always be positioned to the side of the trajectory of any valve core in case it releases. Propelled by strut air pressure, serious injury could result. 5. Loosen the swivel nut. For a valve with a valve core (AN2687-1), rotate the swivel nut one turn (counter clockwise). Using a tool designed for the purpose, depress the valve core to release all of the air in the strut. For a valve without a valve core (MS28889), rotate the swivel nut sufficiently to allow the air to escape. 6. When all air has escaped from the strut, it should be compressed completely. Aircraft on jacks may need to have the lower strut jacked with an exerciser jack to achieve full compression of the strut. [Figure 13-26] 7. Remove the valve core of an AN6287 valve [Figure 13-25D] using a valve core removal tool. Position the aircraft so that the shock struts are in the 5/8-inch hex nut Valve assembly 3/4-inch hex body Yellow valve cap Core Valve core housing O-ring Valve seat 3/4-inch hex body Packing Pin Air orifice A. Valve core-type strut fitting AN6287-1 3/4-inch hex body Back-up ring Stem O-ring B. Strut fitting with no core MS 28889-1 Figure 13-24. Valve core-type (A) and core-free valve fittings (B) are used to service landing gear shock struts. 13-12 A D B E C F Figure 13-25. Steps in servicing a landing gear shock strut include releasing the air from the strut and removing the service valve from the top of the strut to permit the introduction of hydraulic fluid. Note that the strut is illustrated horizontally. On an actual aircraft installation, the strut is serviced in the vertical position (landing gear down). [Figure 13-27] Then, remove the entire service valve by unscrewing the valve body from the strut. [Figure 13-25E] 8. Fill the strut with hydraulic fluid to the level of the service valve port with the approved hydraulic fluid. 9. Re-install the air service valve assembly using a new O-ring packing. Torque according to applicable manufacturer’s specifications. If an AN2687-1 valve, install a new valve core. 10. Inflate the strut. A threaded fitting from a controlled source of high pressure air or nitrogen should be screwed onto the servicing valve. Control the flow with the service valve swivel nut. The correct amount of inflation is measured in psi on some struts. Other manufacturers specify struts to be inflated until extension of the lower strut is a certain measurement. Follow manufacturer’s instructions. Shock struts should always be inflated slowly to avoid excess heating and over inflation. 11. Once inflated, tighten the swivel nut and torque as specified. 12. Remove the fill hose fitting and finger tighten the valve cap of the valve. 13-13 Hydraulic fluid bleed hose Exerciser jack compression of the strut to expel the entrapped air. An example procedure for bleeding the shock strut follows. 1. Construct and attach a bleed hose containing a fitting suitable for making an airtight connection at the shock strut service valve port. Ensure a long enough hose to reach the ground while the aircraft is on jacks. 2. Jack the aircraft until the shock struts are fully extended. 3. Release any air pressure in the shock strut. 4. Remove the air service valve assembly. 5. Fill the strut to the level of the service port with approved hydraulic fluid. 6. Attach the bleed hose to the service port and insert the free end of the hose into a container of clean hydraulic fluid. The hose end must remain below the surface of the fluid. 7. Place an exerciser jack or other suitable jack under the shock strut jacking point. Compress and extend the strut fully by raising and lowering the jack. Continue this process until all air bubbles cease to form in the container of hydraulic fluid. Compress the strut slowly and allow it to extend by its own weight. Ensure that the hydraulic fluid is still at the appropriate level before moving on to the next step. 8. Remove the exerciser jack. Lower the aircraft and remove all other jacks. 9. Remove the bleed hose assembly and fitting from the service port of the strut. Figure 13-26. Air trapped in shock strut hydraulic fluid is bled by exercising the strut through its full range of motion while the end of an air-tight bleed hose is submerged in a container of hydraulic fluid. 10. Install the air service valve, torque, and inflate the shock strut to the manufacturer’s specifications. Landing Gear Alignment, Support, & Retraction Retractable landing gear consist of several components that enable it to function. Typically, these are the torque links, trunnion and bracket arrangements, drag strut linkages, electrical and hydraulic gear retraction devices, as well as locking, sensing, and indicating components. Additionally, nose gear have steering mechanisms attached to the gear. Figure 13-27. This valve tool features internal and external thread chasers, a notched valve core removal/installation tool, and a tapered end for depressing a valve core or clearing debris. Bleeding Shock Struts It may be necessary to bleed a shock strut during the service operation or when air becomes trapped in the hydraulic fluid inside the strut. This can be caused by low hydraulic fluid quantity in the strut. Bleeding is normally done with the aircraft on jacks to facilitate repeated extension and Alignment As previously mentioned, a torque arm or torque links assembly keeps the lower strut cylinder from rotating out of alignment with the longitudinal axis of the aircraft. In some strut assemblies, it is the sole means of retaining the piston in the upper strut cylinder. The link ends are attached to the fixed upper cylinder and the moving lower cylinder with a hinge pin in the center to allow the strut to extend and compress. 13-14