CASAModB1-04ElectronicFundamentalsB1-Semiconductors.pdf
Document Details
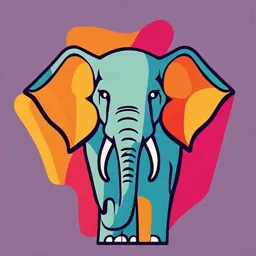
Uploaded by AccomplishedPlumTree
2022
CASA
Tags
Full Transcript
Introduction to Semiconductors (4.1) Learning Objectives 4.1.1.1.1 Describe semiconductor materials, electron configurations and their electrical properties (S). 4.1.1.1.2 Describe P and N type materials including the effects of impurities of conduction, majority and minority carri...
Introduction to Semiconductors (4.1) Learning Objectives 4.1.1.1.1 Describe semiconductor materials, electron configurations and their electrical properties (S). 4.1.1.1.2 Describe P and N type materials including the effects of impurities of conduction, majority and minority carriers (S). Summary Before diving into the types of semiconductors used in electronics, it is important to understand the characteristics of semiconductors and how they differ from conductors and insulators. The following content is a refresh from Electron Theory addressed in Module 03 Electrical Fundamentals as well as an introduction into how semiconductors operate and how they are created (doping). 2022-11-10 B1-04 Electronic Fundamentals Page 8 of 163 CASA Part 66 - Training Materials Only Conduction in Semiconductors Semiconductors A semiconductor is a material that is between conductors and insulators in its ability to conduct electrical current. A semiconductor in its pure (intrinsic) state is neither a good conductor nor a good insulator. The most common single-element semiconductors are silicon, germanium, and carbon. Compound semiconductors such as gallium arsenide are also commonly used. The single element semiconductors are characterized by atoms with four valence electrons. © Aviation Australia Semiconductor atoms (Germanium, Silicon and Carbon) 2022-11-10 B1-04 Electronic Fundamentals Page 9 of 163 CASA Part 66 - Training Materials Only Energy Bands Recall that the valence shell of an atom represents a band of energy levels and that the valence electrons are confined to that band. When an electron acquires enough additional energy, it can leave the valence shell, become a free electron, and exist in what is known as the conduction band. The difference in energy between the valence band and the conduction band is called an energy gap. This is the amount of energy that a valence electron must have in order to jump from the valence band to the conduction band. Once in the conduction band, the electron is free to move throughout the material and is not tied to any given atom. © Aviation Australia Energy bands The figure above shows energy diagrams for insulators, semiconductors, and conductors. Notice that insulators (left) have a very wide energy gap. Valence electrons do not jump into the conduction band except under breakdown conditions where extremely high voltages are applied across the material. Semiconductors (middle) have a much narrower energy gap. This gap permits some valence electrons to jump into the conduction band and become free electrons. By contrast, the energy bands in conductors (right) overlap. In a conductive material there is always a large number of free electrons. 2022-11-10 B1-04 Electronic Fundamentals Page 10 of 163 CASA Part 66 - Training Materials Only Semiconductor Atoms and Conductor Atoms Silicon vs Copper Let’s examine some of the primary reasons that Silicon is a semiconductor and Copper is a conductor. Atomic structure of the silicon atom and the copper atom are shown below. Notice that the valence shell of Silicon contains 4 electrons and the valence shell of Copper contains 1 electron. Aviation Australia Silicon and Copper atomic structure The valence electron in the copper atom “feels” an attractive force of +1 compared to a valence electron in the silicon atom which “feels” an attractive force of +4. So, there is four times more force trying to hold a valence electron to the atom in silicon than in copper. The copper’s valence electron is in the fourth shell, which is a greater distance from its nucleus than the silicon’s valence electron in the third shell. Recall that electrons farthest from the nucleus also have the most energy. Therefore, the valence electron in copper has less force holding it to the atom than the valence electrons in silicon. Also, the valence electron in copper has more energy than the valence electron in silicon. This means that it is easier for valence electrons in copper to acquire enough additional energy to escape from their atoms and become free electrons in the conduction band than it is in silicon. In fact, large numbers of valence electrons in copper already have sufficient energy to be free electrons at normal room temperature. 2022-11-10 B1-04 Electronic Fundamentals Page 11 of 163 CASA Part 66 - Training Materials Only Semiconductor Characteristics The atomic structures of silicon, germanium and carbon are shown below. Silicon is the most widely used material in diodes, transistors, integrated circuits, and other semiconductor devices. Notice that each of these elements have the characteristic four valence electrons. © Aviation Australia Germanium, Silicon and Carbon Why is silicon the preferred semiconductor in electronics? The valence electrons in germanium are in the fourth shell while those in silicon are in the third shell, closer to the nucleus. This means that the germanium valence electrons are at higher energy levels than those in silicon and, therefore, require a smaller amount of additional energy to escape from the atom. This property makes germanium more unstable at high temperatures, and is the reason that silicon is often preferred as a semiconductor to germanium. In contrast, carbon's valence band is much closer to the nucleus which creates insulator-like properties in a face-centered cubic crystallisation (such as diamond). The main factor in what makes a good semiconductor is the capability of the elements crystal to accept dopant (impurities) and behave differently. So for example, diamond, even heavily doped would be a large band gap semiconductor, or more insulator-like. So a lot of voltage needs to be pumped to make any use of it. This voltage may kill other devices and thus carbon is rarely used in electronic components. 2022-11-10 B1-04 Electronic Fundamentals Page 12 of 163 CASA Part 66 - Training Materials Only Covalent Bonds in Silicon The diagram below shows how each silicon atom positions itself with four adjacent silicon atoms to form a silicon crystal. A silicon atom with its four valence electrons shares an electron with each of its four neighbours. This effectively creates eight valence electrons for each atom and produces a state of chemical stability. Also, this sharing of valence electrons produces the covalent bonds that hold the atoms together; each shared electron is attracted equally by two adjacent atoms which share it. Silicon covalent bonding Covalent bonding in an intrinsic silicon crystal is shown below. An intrinsic crystal is one that has no impurities (pure silicon). Covalent bonding for germanium is similar because it also has four valence electrons. Intrinsic silicon crystal 2022-11-10 B1-04 Electronic Fundamentals Page 13 of 163 CASA Part 66 - Training Materials Only Conduction in Semiconductors The electrons of an atom can exist only within prescribed energy bands. Each shell around the nucleus corresponds to a certain energy band and is separated from adjacent shells by energy gaps in which no electrons can exist. This is shown for a silicon crystal with only unexcited (no external energy such as heat) silicon atoms. This condition occurs only at a temperature of 0 Kelvin. Aviation Australia Conduction in semiconductors 2022-11-10 B1-04 Electronic Fundamentals Page 14 of 163 CASA Part 66 - Training Materials Only Conduction Electrons and Holes An intrinsic (pure) silicon crystal at room temperature has sufficient heat (thermal) energy for some valence electrons to jump the gap from the valence band into the conduction band, becoming free electrons. Free electrons are also called conduction electrons. When an electron jumps to the conduction band, a vacancy is left in the valence band within the crystal. This vacancy is called a hole. This is illustrated in the energy diagram (left) and in the bonding diagram (right). Aviation Australia Electrons and holes For every electron raised to the conduction band by external energy, there is one hole left in the valence band, creating what is called an electron-hole pair. Recombination occurs when a conduction-band electron loses energy and falls back into a hole in the valence band. To summarise, a piece of intrinsic silicon at room temperature has, at any instant, a number of conduction-band (free) electrons that are unattached to any atom and are essentially drifting randomly throughout the material. There are also an equal number of holes in the valence band created when these electrons jump into the conduction band. 2022-11-10 B1-04 Electronic Fundamentals Page 15 of 163 CASA Part 66 - Training Materials Only Aviation Australia Pure silicon at room temperature contains a small number of drifting free electrons and their electron holes Electron and Hole Current When a voltage is applied across a piece of intrinsic silicon, the thermally generated free electrons in the conduction band, which are free to move randomly in the crystal structure, are now easily attracted toward the positive end. This movement of free electrons is one type of current in a semi- conductive material and is called electron current. Aviation Australia Electron and hole current 2022-11-10 B1-04 Electronic Fundamentals Page 16 of 163 CASA Part 66 - Training Materials Only Another type of current occurs at the valence level, where the holes created by the free electrons exist. Electrons remaining in the valence band are still attached to their atoms and are not free to move randomly in the crystal structure as the free electrons are. However, a valence electron can move into a nearby hole with little change in its energy level, thus leaving another hole where it came from. Effectively, the hole has moved from one place to another in the crystal structure. 2022-11-10 B1-04 Electronic Fundamentals Page 17 of 163 CASA Part 66 - Training Materials Only Doping in Semiconductors Doping Semi-conductive materials do not conduct current well and are of limited value in their intrinsic state. This is because of the limited number of free electrons in the conduction band and holes in the valence band. Intrinsic silicon (or germanium) must be modified to increase the number of free electrons or holes to increase its conductivity and make it useful in electronic devices. This is done by adding impurities to the intrinsic material, a process known as doping. Two types of extrinsic semi-conductive materials, N-type and P-type, are the key building blocks for most types of electronic devices. The conductivity of silicon and germanium can be drastically increased by the controlled addition of impurities to the intrinsic (pure) semi-conductive material. This process, called doping, increases the number of current carriers (electrons or holes). The two categories of impurities are N-type and P- type. Doping silicon 2022-11-10 B1-04 Electronic Fundamentals Page 18 of 163 CASA Part 66 - Training Materials Only N-Type Semiconductor To increase the number of conduction-band electrons in intrinsic silicon, pentavalent impurity atoms are added. These are atoms with five valence electrons such as arsenic (As), phosphorus (P), bismuth (Bi) and antimony (Sb). The following diagram demonstrates silicon structure with arsenic added an as impurity. N-type doping Four of arsenics valence electrons are used to form the covalent bonds with silicon atoms, leaving one extra electron. This extra electron becomes a conduction electron because it is not attached to any atom. Because the pentavalent atom gives up an electron, it is often called a donor atom. The number of conduction electrons can be carefully controlled by the number of impurity atoms added to the silicon. A conduction electron created by this doping process does not leave a hole in the valence band because it is in excess of the number required to fill the valence band. 2022-11-10 B1-04 Electronic Fundamentals Page 19 of 163 CASA Part 66 - Training Materials Only Majority and Minority Carriers Since most of the current carriers are electrons, silicon (or germanium) doped with pentavalent atoms is an N-type semiconductor (the N stands for the negative charge on an electron). The electrons are called the majority carriers in N-type material. Although the majority of current carriers in N-type material are electrons, there are also a few holes that are created when electron-hole pairs are thermally generated. These holes are not produced by the addition of the pentavalent impurity atoms. Holes in an N-type material are called minority carriers. P-Type Semiconductor To increase the number of holes in intrinsic silicon, trivalent impurity atoms are added. These are atoms with three valence electrons such as boron (B), indium (In) and gallium (Ga). Each trivalent atom (boron, in this case) forms covalent bonds with four adjacent silicon atoms. All three of the boron atom’s valence electrons are used in the covalent bonds; since four electrons are required, a hole results when each trivalent atom is added. © Aviation Australia P-type doping Because the trivalent atom can take an electron, it is often referred to as an acceptor atom. The number of holes can be carefully controlled by the number of trivalent impurity atoms added to the silicon. A hole created by this doping process is not accompanied by a conduction (free) electron. 2022-11-10 B1-04 Electronic Fundamentals Page 20 of 163 CASA Part 66 - Training Materials Only Majority and Minority Carriers Since most of the current carriers are holes, silicon (or germanium) doped with trivalent atoms is called a P-type semiconductor. Holes can be thought of as positive charges because the absence of an electron leaves a net positive charge on the atom. The holes are the majority carriers in P-type material. Although the majority of current carriers in P-type material are holes, there are also a few free electrons that are created when electron-hole pairs are thermally generated. These free electrons are not produced by the addition of the trivalent impurity atoms. Electrons in P-type material are the minority carriers. 2022-11-10 B1-04 Electronic Fundamentals Page 21 of 163 CASA Part 66 - Training Materials Only