Semiconductor Fundamentals (PDF)
Document Details
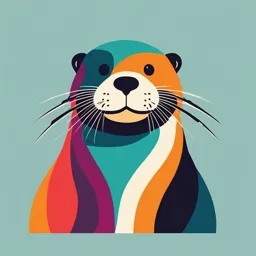
Uploaded by HardierMeadow
null
2022
Tags
Summary
This document provides an introduction to semiconductors, covering their fundamental properties, components, and advantages. The text details the function of semiconductor devices like diodes, transistors, and integrated circuits. It explains the importance of semiconductors in various electronic equipment and discusses the advantages of solid-state components over traditional thermionic valves.
Full Transcript
4.1.1 - Diodes Introduction To acquire a basic understanding of semiconductors, you must have some basic knowledge of atomic theory and the structure of semiconductors. We will discuss the basic materials used in manufacturing both discrete devices, such as diodes, transistors and integrated circuit...
4.1.1 - Diodes Introduction To acquire a basic understanding of semiconductors, you must have some basic knowledge of atomic theory and the structure of semiconductors. We will discuss the basic materials used in manufacturing both discrete devices, such as diodes, transistors and integrated circuits. Electrons in the valence band are loosely bound to atom. When they get some energy, these electrons can break free from the atom. For example, diodes can be used as rectifiers to produce pulsating DC from AC, a transistor can be used as a variable resistance to vary the current in a heating element and an integrated circuit can be used to amplify and demodulate a radio signal. All of these components are made of special materials we know as semiconductors. Semiconductor devices are extremely small, lightweight components which consume only a small amount of power and are highly efficient and reliable. The thermionic valves that were once widely used in practically all types of electronic equipment have been almost completely replaced by the newer and better semiconductor devices. These electrons are then free to move in the material and not bound to atom. The energy levels of such electrons make the conduction band (band of energy due to free electrons) Let’s consider some of the specific reasons for this significant transition from the use of thermionic valves to semiconductor components in electronic equipment. The Importance of Semiconductors Semiconductors essentially serve as the basic building materials which are used to construct some very important electronic components. These semiconductor components are, in turn, used to construct electronic circuits and equipment. The three most commonly used semiconductor devices are: ➢ Diodes ➢ Transistors ➢ Integrated circuits The primary function of semiconductor devices in electronic equipment is to control currents or voltages in such a way as to produce a desired end result for the circuit designed. Module 4B1 ETBN 0480 May 2022 Edition 3 Electronic Fundamentals Semiconductor Advantages The small size of the solid-state component also makes it suitable for use in portable electronic equipment. Although equipment of this type can be constructed with thermionic valves, such equipment would be much larger and heavier. Components made of semiconductor materials are often referred to as solid-state components because they are made from solid materials. Because of this construction, these components are more rugged than thermionic valves which are made of glass, metal, and ceramic materials and are able to operate under extremely hazardous environmental conditions, making them more reliable. A typical transistor is only a fraction of an inch high and wide while a vacuum tube of comparable performance may be an inch or more wide and several inches high. The small size also means a significant weight saving. The solid-state construction also eliminates the need for filaments or heaters as found in all thermionic valves. This means that additional power is not required to operate the filaments and component operation is cooler and more efficient. By eliminating the filaments, a prime source of trouble is also avoided because they generally have a limited life expectancy. The absence of filaments also means that a warm up period is not required before the device can operate properly. In other words, the solid-state component is ready to the instant it receives electrical power. The very nature of a solid-state component makes it suitable for production in mass quantities which brings about a high cost saving. In fact, a large number of solid-state components can be constructed as easily and quickly as a single component. The most sophisticated semiconductor devices are integrated circuits. These are complete circuits where all of the components are constructed with semiconductor materials in a single micro miniature package. Solid-state components are also able to operate with very low voltages (between 1 and 25 volts) while thermionic valves usually require an operating voltage of 100 volts or more. These devices not only replace individual electronic circuits but also complete pieces of equipment or entire systems. This means that solid-state components generally use less power than thermionic valves and are, therefore, more suitable for use in portable equipment which obtains its power from batteries. The lower voltages are also much safer to work with. Pocket-size radios, hand held calculators, and small battery operated television receivers are typical examples of devices which take advantage of highly efficient, power saving components. Module 4B1 ETBN 0480 May 2022 Edition Solid-state components are much less expensive than comparable vacuum tube components. 4 Entire computers and radio receivers can be constructed as a single device no larger than a typical transistor. Integrated circuits have taken us one step farther in improving electronic equipment through the use of semiconductor materials. All electronic equipment has benefited from solid state components and particularly from the development of integrated circuits. Electronic Fundamentals Semiconductor Disadvantages Diode (Semiconductor) Characteristics And Properties Although solid-state components have many advantages over the thermionic valves that were once widely used, they also have several inherent disadvantages. Silicon And Germanium Atoms Two types of semiconductive materials are silicon and germanium. Both the silicon and germanium atoms have four valence electrons, a trait that makes these materials excellent for semiconductive use. First, solid-state components are highly susceptible to changes in temperature and can be damaged if they are operated at extremely high temperatures. These atoms differ from each other, in that, silicon has 14 protons in its nucleus and germanium has 32. Additional components are often required simply for the purpose of stabilizing solid-state circuits so that they will operate over a wide temperature range. Solid-state components may be easily damaged by exceeding their power dissipation limits and they may also be occasionally damaged when their normal operating voltages are reversed. In comparison, vacuum tube components are not nearly as sensitive to temperature changes or improper operating voltages. Fig 1 shows a representation of the atomic structure for both materials. The valence electrons in germanium are in the fourth shell while the ones in silicon are in the third shell, closer to the nucleus. This means that the germanium valence electrons are at higher energy levels than those in silicon and, therefore, require a smaller amount of additional energy to escape from the atom. There are still a few areas where semiconductor devices cannot replace tubes. This is particularly true in high power, ultra-high radio frequency applications. However, as semiconductor technology develops, these limitations are gradually being overcome. Despite the several disadvantages just mentioned, solid-state components are still the most efficient and reliable devices to be found. They are used in all new equipment designs and new applications are constantly being found for these devices in the military, industrial, and consumer fields. Fig 1 – Silicon and Germanium Atoms Module 4B1 ETBN 0480 May 2022 Edition 5 Electronic Fundamentals This property makes germanium more unstable at high temperatures and this is a basic reason why silicon is the most widely used semiconductive material. Both silicon and germanium are tetravalent in atomic structure, meaning they have four valency electrons in their outer shell. Most semiconductor materials are in Group 4(a) of the Periodic table, an extract of which is shown below in Table 1 Table 1 - Elements (groups indicate the number of valence electrons); In a good Insulator, surface charge stays put for a very long time, however, semiconductor spot surface charges very slowly move away. The slow movement is due to thermal diffusion (random motion due to thermal energy) of electrons. Doping Electrons are always in some random motion, which we perceive as motion (kinetic) energy of “heat”, somewhat like a “neat” pile of leaves eventually spreading out over the whole lawn due to random motions due to changing wind directions, etc. The operation of “active” semi-conductor devices depends on producing and controlling layers of electric charge. These usually occur at the interface between two kinds of semi-conductor materials, or between a semi-conductor and a metal conductor. To produce a controlled result, semiconductors are first highly purified – typically only one “impure” atom in 100,000,000 silicon atoms. A thick silicon rod is “zone refined” – melted and then cooled slowly, starting from one end, to form a very pure solid. When a Group 5 material from Table 1 is added, the average atomic number is higher. This is called N (negative) type material. The average nuclear positive charge per unit volume is greater than “intrinsic” (pure) silicon, and there are also more electrons as well. Of course, a piece of material as a whole is electrically neutral. When Group 3 material is added, the average atomic number is lower. This is called P (positive) type material. The average nuclear positive charge per unit volume is less than pure silicon. This “zone refining” process is similar to freezing pure water ice out of salty ocean water – Icebergs near the earth’s poles consist of pure water (no salt) – “silicon ice” (solid) which is slowly frozen from melted silicon is very pure. The impurities are mostly trapped in the end of the rod which solidifies last. That end is cut off and used for other purposes where the silicon does not need to be so pure Module 4B1 ETBN 0480 May 2022 Edition Doping is achieved by using Group 3a or 5a material in Table 1 to change the balance. A pure or intrinsic semiconductor (Tetravalent – Group 4) can be doped with a pentavalent (Group 5) or trivalent (Group 3) material to obtain two basic types of semiconductor materials. 6 Electronic Fundamentals The PN Junction When two different semi-conductor materials are brought together, there is a natural migration of electrons from the “n” type material and holes in the “p” type material, as shown in Fig 2 As this process progresses there is an area at the PN junction, which is formed called the “depletion layer”, which in turn creates a “Barrier Potential, which can be seen in Fig 2 and 3. Unbiased P-N Junction The charges in the barrier region of the junction set up a barrier potential difference which prevents further drift of carriers. Electrons and holes combine because heat energy causes the atoms in material to vibrate. If you pick up a warm object, the warmth that you feel is caused by the vibrating atoms. The higher the ambient temperature, the stronger the mechanical vibration will be. These vibrations can occasionally dislodge an electron from the valence orbit and provide enough energy to combine with holes. This continues until all of the atoms near the junction in the p-type material have their holes filled. This is called the depletion layer since it is depleted of current carriers. Fig 2 PN Junction with small Depletion Region The barrier potential, VB, is the amount of energy required to move electrons through the electric field. At 25ºC, it is approximately 0.7 V for silicon and 0.3 V for germanium. As the junction temperature increases, the barrier potential decreases and vice versa. Fig 3 – Unbiased PN Junction with Depletion Region Module 4B1 ETBN 0480 May 2022 Edition 7 Electronic Fundamentals Forward Bias As electrons leave the N region, more flow in from the negative terminal of the battery. Thus, current through the N region is formed by the movement of conduction electrons (majority carriers) toward the junction (Fig 14). The primary usefulness of the P-N junction is its ability to allow current in only one direction and to prevent current in the other direction as determined by the bias. There are two practical bias conditions for a P-N junction: forward and reverse. Either of these conditions is created by application of an external voltage of the proper polarity and magnitude. The effect of the barrier potential in the depletion region is to oppose forward bias. This is because the negative ions near the junction in the P region tend to prevent electrons from crossing the junction into the P region. You can think of the barrier potential effect (VB) as simulating a small battery connected in a direction to oppose the forward bias voltage, as shown in Fig 15 and Fig 16. When the semiconductor goes into forward bias, the negative terminal of the battery pushes the conduction band electrons in the N region toward the junction, while the positive terminal pushes the holes in the P region also toward the junction. The resistances Rp and Rn represent the dynamic resistance of the P and N materials. The external bias voltage must overcome the effect of the barrier potential before the P-N junction conducts. Once the diode is conducting, a voltage will be dropped across the device. This occurs because the diodes semiconductor material has a low but finite resistance value and the current flowing through it must produce a corresponding voltage drop. As it turns out, this forward bias voltage drop is approximately equal to the barrier potential. This is 0.3V for a germanium diode and 0.7V for a silicon diode. The amount of forward bias current, IF, is a function of the applied DC bias, V, the forward voltage drop, VF, and the external resistance R. The relationship simply involves Ohm's law as: Fig 4 – Forward Bias in a P-N Junction When it overcomes the barrier potential, the external voltage source provides the N region electrons with enough energy to penetrate the depletion region and cross the junction, where they combine the P region holes. Module 4B1 ETBN 0480 May 2022 Edition 8 𝑰𝑭 = 𝑽 − 𝑽𝑭 𝑹 Electronic Fundamentals For example, the forward current in a silicon diode with a bias voltage of 10 volts and an external resistor of 100 ohm is: 𝑰𝑭 = Reverse Bias 𝟏𝟎 − 𝟎. 𝟕 𝟗. 𝟑 = = 𝟗𝟑𝒎𝑨 𝟏𝟎𝟎 𝟏𝟎𝟎 When the voltage on the anode is greater than the voltage on the cathode, current will flow through the diode. Reverse bias is the condition that prevents current across the P-N junction from flowing. Fig 6 shows a DC voltage source connected to reverse bias the diode. Notice that the negative terminal of the battery is connected to the P region and the positive terminal is connected to the N Region The negative terminal of the battery attracts holes in the P region away from the P-N junction, while the positive terminal also attracts electrons away from the junction. As electrons and holes move away from the junction, the depletion region widens; more positive ions are created in the N region and more negative ions are created in the P region, as shown below. The initial flow of majority carriers away from the junction is called transient current and lasts only for a very short time upon application of reverse bias. The depletion region widens until the potential difference across it equals the external bias voltage. Fig 5 – Typical Forward Bias Voltages in a Germanium P-N Junction Fig 6 – Reverse Bias PN Junction Module 4B1 ETBN 0480 May 2022 Edition 9 Electronic Fundamentals At this point, the holes and electrons stop moving away from the junction and majority current ceases, as indicated below in Fig 7. Reverse Bias Breakdown When an ordinary P-N junction diode is reverse biased, normally only very small reverse saturation current flows. This current is due to movement of minority carriers and is almost independent of the voltage applied. However, if the reverse bias is increased, a point is reached when the junction breaks down and the reverse current increases abruptly. This current could be large enough to destroy the junction, but if the reverse current is limited by means of a suitable series resistor, the power dissipation at the junction will not be excessive, and the device may be operated continuously in its breakdown region to its normal (reverse saturation) level. It is found that for a suitably designed diode, the breakdown voltage is very stable over a wide range of reverse currents. This quality gives the breakdown diode many useful applications as a voltage reference source. The critical value of the voltage, at which the breakdown of a P-N junction diode occurs is called the breakdown voltage. Fig 7 Maximum Reverse Bias Conditions (VB = VS) When the diode is reverse biased, the depletion region effectively acts as an insulator between the layers of oppositely charged ions, forming an effective capacitance. Since the depletion region widens with increased reverse biased voltage, the capacitance decreases and vice versa. This internal capacitance is called the depletion region capacitance. The breakdown voltage depends on the width of the depletion region, which, in turn, depends on the doping level. The junction offers almost zero resistance at the breakdown point. The minority carriers flowing through the junction under reverse biased conditions, acquire a kinetic energy which increases with the increase in reverse voltage. At a sufficiently high reverse voltage (say 5V or more), the kinetic energy of minority carriers becomes so large that they knock out electrons from the covalent bonds of the semiconductor material. As a result of collision, the liberated electrons in turn liberate more electrons and the current becomes very large leading to the breakdown of the crystal structure itself. This phenomenon is called the avalanche breakdown. Module 4B1 ETBN 0480 May 2022 Edition 10 Electronic Fundamentals The P-N Junction Diode Invariably, it is the cathode that is marked on the device. Some typical diode markings are shown below in Fig 9. Construction The electrodes of the diode are called the Anode (A) (from the Greek meaning up) and Cathode (K) (from the Greek meaning down). Hodos means route, hence the term diode (di+hodos - meaning 2 routes). Conventionally, the current flows from A to K. Thus, since conventional current is from positive to negative, the P side of the diode forms the Anode and N side the Cathode, see Fig 8. Fig 9 – PN Diode Common Component Markings Fig 8 – PN Diode Symbol And Associated Polarities In common with all other electronic device symbols, the arrow on the symbol points in the direction of conventional current flow. The most common use of a diode is as a rectifier. It converts AC to DC. Module 4B1 ETBN 0480 May 2022 Edition 11 Electronic Fundamentals Diode Characteristic Curves Fig 10 on the following page shows a graph of diode current versus voltage. The upper right quadrant of the graph represents the forward biased condition. As you can see, there is very little forward current (IF) for forward voltages (VF) below the barrier potential. As the forward voltage approaches the value of the barrier potential (0.7V for silicon and 0.3V for germanium), the current begins to increase. Once the forward voltage reaches the barrier potential, the current increases drastically and must be limited by a series resistor. The voltage across the forward biased diode remains approximately equal to the barrier potential. The lower left quadrant of the graph represents the reverse biased condition. As the reverse voltage (VR) increases to the left, the current remains near zero until the breakdown voltage is reached. When breakdown occurs, there is a large reverse current which, if not limited, can destroy the diode. Typically, the breakdown voltage is greater than 50V for most rectifier diodes. Remember, most diodes should not be operated in reverse breakdown. Module 4B1 ETBN 0480 May 2022 Edition 12 Electronic Fundamentals Fig 10 – Characteristics of a P-N Junction Diode Module 4B1 ETBN 0480 May 2022 Edition 13 Electronic Fundamentals Diode Characteristic Curves - Germanium Diode Reverse Characteristics The V-I characteristic curve in Fig 11 on the following page is for a germanium diode. Let’s consider its operation in detail. The V-I curve also shows that when the diode is reverse-biased, the reverse current that flows is extremely small. Notice that the reverse current increases slightly as the reverse voltage increases but remains less than 0.1mA until the reverse voltage approaches a value of 20 volts. Then the reverse current suddenly increases to a much higher value. Forward Characteristics The diagram shows that the forward current through a germanium diode is extremely small and almost insignificant until the forward bias voltage across the diode increases beyond a value of approximately 0.2 volts. The forward current increases as the forward bias voltage is increased still further. The increase in forward current really starts to occur as the external bias voltage overcomes the diode’s internal barrier voltage. Once the bias voltage exceeds the barrier voltage (0.3V), the forward current increases very rapidly and at a linear rate because the diode then acts as a low resistance. If this forward current continued to rise, the diode would eventually be damaged by an excessive flow of current. Throughout the linear portion of the curve, the voltage across the diode is only several tenths of a volt as shown. While the forward voltage drop is not constant, it changes very little over a wide current range. A tremendous change in forward current occurs while the voltage across the diode changes only a small amount. The point at which the bias voltage equals the barrier voltage is indicated opposite. Notice that this point occurs when the bias voltage is equal to 0.3V. Also notice that the diode’s forward current is equal to 1mA at this time and that this current can increase above 5mA while the corresponding voltage across the diode remains below 0.4 volts. This sudden increase in reverse current results because the reverse bias voltage becomes strong enough to tear many valence electrons from their parent atoms and therefore increase the number of electron-hole pairs in the N and P materials. This causes an increase in minority carriers which in turn support a higher reverse current. In other words the junction simply breaks down when the reverse bias voltage approaches a value of 20 volts. The voltage at which the sudden change occurs is commonly referred to as the breakdown voltage. This breakdown voltage will vary from one diode to the next since it is determined by the exact manner in which the diode is constructed. In certain cases, ordinary germanium diodes can be damaged when breakdown occurs, however, there are special diodes which are designed to operate in this region. These special devices, known as Zener diodes, will be described in detail later. When breakdown occurs, the diode no longer offers a high resistance to the flow of reverse current and therefore cannot effectively block current in the reverse direction. For these reasons, operation in the breakdown region is avoided when an ordinary P-N junction diode is being used. The diagram therefore shows that the diode’s internal barrier voltage is approximately 0.3V. However, it is important to realize that this voltage will vary slightly from one germanium diode to the next. Module 4B1 ETBN 0480 May 2022 Edition 14 Electronic Fundamentals Fig 11 – Characteristic Curve for a Germanium Diode Module 4B1 ETBN 0480 May 2022 Edition 15 Electronic Fundamentals Diode Characteristic Curves - Silicon Diode While a silicon diode operates the same as the germanium diode, there are some important differences in their characteristic curves. Forward Characteristics The V-I curve in Fig 12, opposite, shows the characteristics of a typical silicon diode. Notice that the forward characteristics of this diode are basically similar to those of the germanium diode previously described, however, there is an important exception. The internal barrier voltage of the silicon diode is not overcome until the forward bias voltage is equal to approximately 0.7 volts as shown. Beyond this point the forward current increases rapidly and at a linear rate. The corresponding forward voltage across the diode increases only slightly. The exact amount of forward voltage required to overcome the barrier voltage will vary from one silicon diode to the next but will usually be close to the 0.7 volts indicated. Reverse Characteristics The reverse characteristics of the silicon diode are also similar to those of the typical germanium diode previously described. However, the silicon diode has a much lower reverse current than the germanium type as indicated in the diagram. Notice that the reverse current remains well below 0.1mA until the breakdown voltage of the device is reached. Then, as with the germanium diode, a relatively high reverse current is allowed to flow. A breakdown voltage of 45 volts is indicated, however, this voltage will vary from one silicon diode to the next. Also, the reverse currents in many silicon diodes may be in the extremely low nano-ampere range and therefore insignificant for most practical applications. Module 4B1 ETBN 0480 May 2022 Edition 16 Fig 12 – Characteristic Curve for a Silicon Diode Electronic Fundamentals The Breakdown Effect If the reverse bias voltage applied to a diode is increased, the reverse leakage current will remain approximately constant, until a point is reached when a sudden and large increase in current takes place due to avalanche breakdown. The large increase in diode current will dissipate power in the diode and may lead to damage to the device. It is necessary with rectifier type diodes to ensure that the diode is not driven into breakdown. The manufacturers of diodes usually quote a safe maximum inverse voltage, the Peak Inverse Voltage (PIV). When a diode breaks down, due to excessive inverse voltage (i.e. excessive reverse bias), whilst the diode current increases rapidly, the inverse voltage remains approximately constant. Note: Only Zener diodes are designed to operate in the breakdown region, however even these devices require a series resistor, to limit the current flow and avoid exceeding the diodes power rating. Temperature Considerations In some critical applications it is also necessary to consider the effect that temperature has on diode operation. In general, the diode characteristic that is most adversely affected by changes in temperature is the diode’s reverse current. This current is caused by the minority carriers that are present in the N and P sections of the diode as explained previously. At extremely low temperatures the reverse current through a typical diode will be practically zero, but at room temperature this current will be somewhat higher although still quite small. At extremely high temperatures an even higher reverse current will flow which in some cases might interfere with normal diode operation (Fig 13 opposite). Module 4B1 ETBN 0480 May 2022 Edition 17 Fig 13 – Effects of Temperature on a Diode Electronic Fundamentals Silicon v Germanium The Ideal Diode Model Early semiconductor developments used germanium as the commercial, semiconductor material. Due to its ease of processing and more stable temperature characteristics, silicon became the semiconductor of choice. The simplest way to visualise diode operation is to think of it as a switch. Figures 14 and 15 Show Forward and Reverse Bias Models As a consequence of that, most early germanium semiconductors were replaced with silicon. These were primarily transistors and diodes. However, germanium diodes have the advantage of an intrinsically low forward voltage drop, typically 0.3V; this low forward voltage drop results in a low power loss and more efficient diode, making it superior in many ways to the silicon diode. A silicon diode forward voltage drop, by comparison, is typically 0.7V. This lower voltage drop with germanium becomes important in very low signal environments and in low level logic circuits. Fig 14 – Ideal Model Switch And Diode Comparison As a result germanium diodes are finding increasing application in low level digital circuits. With this increased interest, certain general germanium characteristics should be understood. When forward biased, the diode ideally acts as a closed (on) switch and when reverse biased, it acts an open (off) switch as shown in the diagram. First and most important is that of an increased leakage current for germanium at a reverse voltage. This is mitigated to some degree by the fact that in low level circuits the reverse voltage applied to a germanium diode is also usually very low, resulting in a low reverse leakage current (leakage current is directly proportional to reverse voltage). However the leakage current is still larger than with silicon . Fig 15 – Ideal Model Switch And Diode Comparison Module 4B1 ETBN 0480 May 2022 Edition 18 Electronic Fundamentals The ideal characteristic curve for this Reverse Bias model is shown in Fig 16 below. The Practical Diode Model The next higher level of accuracy includes the barrier potential in the diode model. Fig 17 – Ideal Forward Bias Model with Barrier Potential In this approximation, the forward biased diode, shown in Fig 17 above is represented as a closed switch in series with a small "battery" equal to the barrier potential VB (0.7V for Si and 0.3V for Ge), as shown in the diagram. Fig 16 – Ideal Reverse Bias Model Curve The positive end of the battery is toward the anode. Note that the forward voltage and the reverse current are always zero in the ideal case. This ideal model, of course, neglects the effect of the barrier potential, the internal resistances and other parameters. Keep in mind that the barrier potential is not a voltage source and cannot be measured with a voltmeter; rather it only has the effect of a battery when forward bias is applied because the forward bias voltage, VBIAS, must overcome the barrier potential before the diode begins to conduct current. You may want to use the ideal model when you are troubleshooting or trying to figure out the operation of a circuit and are not concerned with more exact values of voltage or current. Module 4B1 ETBN 0480 May 2022 Edition 19 Electronic Fundamentals The reverse biased diode, as shown in Fig 18 is represented by an open switch, as in the ideal case, because the barrier potential does not affect reverse bias, as shown in the diagram opposite. The Complex Diode Model One more level of accuracy will be considered at this point. Fig 20 below, shows the forward biased diode model with both the barrier potential and the low forward dynamic resistance. Fig 18 – Ideal Reverse Bias Model with Barrier Potential The characteristic curve for this model is shown in the diagram below. In this book, the practical model is used in analysis unless otherwise stated. The Fig 21 also shows how the high internal reverse resistance affects the reverse biased model. Fig 21 – Ideal Reverse Bias Model with Barrier Potential Fig 19 – Ideal Reverse Bias Model with Barrier Potential Module 4B1 ETBN 0480 May 2022 Edition Fig 20 – Ideal Reverse Bias Model with Barrier Potential 20 Electronic Fundamentals The characteristic curve is shown below in Fig 22. Fig 22 – Ideal Reverse Bias Model with Barrier Potential Other parameters such as junction capacitance and breakdown voltage become important only under certain operating conditions and will be considered only where appropriate. Module 4B1 ETBN 0480 May 2022 Edition 21 Electronic Fundamentals The DMM Diode Test Position When the Diode is Defective A Multimeter can be used as a fast and simple way to check a diode. As you know, a good diode will show an extremely high resistance (or open) with reverse bias and a very low resistance with forward bias. When a diode has failed open, you get an open circuit voltage reading (2.6V is typical) for both the forward bias and the reverse bias condition, as shown in Fig 24. A defective open circuit diode will show an extremely high resistance (or open) for both. A defective short circuit or resistive diode will show zero or a low resistance for both forward and reverse bias. An open circuit diode is the mode common type of failure. If a diode is short circuit, the meter reads 0V in both forward bias and reverse bias tests. Sometimes, a failed diode may exhibit a small resistance for both bias conditions rather than a pure short. In this case the meter will show a small voltage much less than the correct open voltage. Many Digital Multimeters (DMMs) have a diode test position which provides a convenient way to test a diode. A typical DMM, as shown in Fig 10, has a small diode symbol to mark the position of the function switch. When set to diode test, the meter provides an internal voltage sufficient to forward bias and reverse bias diode. For example, a resistive diode may result in a reading of 1.1 V in both directions rather than the correct readings of 0.7 V forward and 2.6 V reverse. This internal voltage may vary among different makes of DMM, but 2.5 V to 3.5 V is a typical range of values. The meter provides a voltage reading or other indication to show the condition of the diode under test. When the Diode is Working In the first diagram in Fig 23, on the next page, the red (positive) lead of the meter is connected to the anode and the black (negative) lead is connected to the cathode to forward bias the diode. If the diode is good, you will get a reading of between 0.5 V and 0.9 V, with 0.7 V being typical for forward bias. In the second diagram, the diode is turned around to reverse bias the diode as shown. If the diode is working properly, you will get a voltage reading based on the meter's internal voltage source. The 2.6 V shown in the diagram represents a typical value and indicates that the diode has an extremely high reverse resistance with essentially all of the internal voltage appearing across it. Module 4B1 ETBN 0480 May 2022 Edition 22 Electronic Fundamentals Fig 23 – Diode Testing – Working Diode Module 4B1 ETBN 0480 May 2022 Edition 23 Electronic Fundamentals Fig 24 – Diode Testing- Defective Diode Module 4B1 ETBN 0480 May 2022 Edition 24 Electronic Fundamentals