Cardiovascular System PDF
Document Details
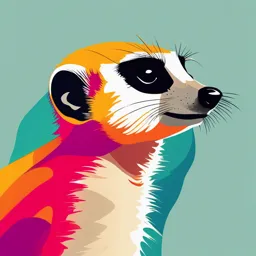
Uploaded by AccomplishedLongBeach
Victoria University
Tags
Related
- Cardiovascular System Anatomy, Physiology & Pathology Notes PDF
- Cardiovascular System Anatomy PDF
- Cardiovascular System Anatomy Physiology & Pathology PDF
- Cardiovascular System Anatomy, Physiology & Pathology Notes PDF
- NATS1010 Human Anatomy and Physiology 2 Cardiovascular System 1 Practical PDF
- Anatomy & Physiology of Cardiovascular System PDF
Summary
These notes cover the Cardiovascular System, specifically focusing on the anatomy and physiology of the human heart. The document details the functions of a circulatory system, the comparison of systemic and pulmonary circuits, and the basic structure and function of the heart.
Full Transcript
Cardiovascular System Learning Objectives: Cardiovascular System 1 (CV1) General functions of the cardiovascular system Describe the functions of a circulatory system and list the major substances transported by the human cardiovascular system. Compare and c...
Cardiovascular System Learning Objectives: Cardiovascular System 1 (CV1) General functions of the cardiovascular system Describe the functions of a circulatory system and list the major substances transported by the human cardiovascular system. Compare and contrast the systemic and pulmonary circuits (circulations) with respect to structure and function Describe the basic structure and function(s) of the heart Trace a drop of blood from the right atrium through the cardiovascular system until it returns to the right atrium, listing all significant anatomical structures the drop of blood encounters along the way. © 2016 Pearson Education, Ltd. 18.1 Heart Anatomy The Pulmonary and Systemic Circuits Heart is a transport system consisting of two side-by-side pumps – Right side receives oxygen-poor blood from tissues Pumps blood to lungs to get rid of CO2, pick up O2, via pulmonary circuit – Left side receives oxygenated blood from lungs Pumps blood to body tissues via systemic circuit © 2016 Pearson Education, Ltd. The Pulmonary and Systemic Circuits (cont.) Receiving chambers of heart – Right atrium Receives blood returning from systemic circuit – Left atrium Receives blood returning from pulmonary circuit Pumping chambers of heart – Right ventricle Pumps blood through pulmonary circuit – Left ventricle Pumps blood through systemic circuit © 2016 Pearson Education, Ltd. Figure 18.1 The systemic and pulmonary circuits. Capillary beds of lungs where gas exchange occurs Pulmonary Circuit Pulmonary Pulmonary arteries veins Aorta and Venae branches cavae Left atrium Left Right ventricle atrium Right Heart ventricle Systemic Circuit Capillary beds Oxygen-rich, of all body CO2-poor blood tissues where gas Oxygen-poor, exchange occurs CO2-rich blood © 2016 Pearson Education, Ltd. Coverings of the Heart Pericardium: double-walled sac that surrounds heart; made up of two layers 1. Superficial fibrous pericardium: functions to protect, anchor heart to surrounding structures, and prevent overfilling 2. Deep two-layered serous pericardium Parietal layer lines internal surface of fibrous pericardium Visceral layer (epicardium) on external surface of heart Two layers separated by fluid-filled pericardial cavity (decreases friction) 3. Epicardium: visceral layer of serous pericardium 4. Myocardium: circular or spiral bundles of contractile cardiac muscle cells Cardiac skeleton: crisscrossing, interlacing layer of connective tissue – Anchors cardiac muscle fibers – Supports great vessels and valves – Limits spread of action potentials to specific paths © 2016 Pearson Education, Ltd. Figure 18.3 The layers of the pericardium and of the heart wall. Pulmonary trunk Fibrous pericardium Parietal layer of serous Pericardium pericardium Myocardium Pericardial cavity Epicardium (visceral layer of serous Heart pericardium) wall Myocardium Endocardium Heart chamber © 2016 Pearson Education, Ltd. Clinical – Homeostatic Imbalance 18.1 Pericarditis – Inflammation of pericardium – Roughens membrane surfaces, causing pericardial friction rub (creaking sound) heard with stethoscope – Cardiac tamponade Excess fluid that leaks into pericardial space Can compress heart’s pumping ability Treatment: fluid is drawn out of cavity (usually with syringe) © 2016 Pearson Education, Ltd. Figure 18.5e Gross anatomy of the heart. Aorta Left pulmonary artery Superior vena cava Left atrium Right pulmonary artery Left pulmonary veins Pulmonary trunk Right atrium Mitral (bicuspid) valve Right pulmonary veins Fossa ovalis Aortic valve Pectinate muscles Pulmonary valve Tricuspid valve Right ventricle Left ventricle Chordae tendineae Papillary muscle Interventricular septum Trabeculae carneae Epicardium Inferior vena cava Myocardium Endocardium Frontal section © 2016 Pearson Education, Ltd. Figure 18.5b Gross anatomy of the heart. Left common carotid Brachiocephalic trunk artery Left subclavian artery Superior vena cava Aortic arch Ligamentum arteriosum Right pulmonary artery Left pulmonary artery Ascending aorta Left pulmonary veins Pulmonary trunk Auricle of left atrium Right pulmonary veins Circumflex artery Right atrium Right coronary artery Left coronary artery (in coronary sulcus) (in coronary sulcus) Anterior cardiac vein Left ventricle Right ventricle Right marginal artery Great cardiac vein Small cardiac vein Anterior interventricular artery (in anterior interventricular sulcus) Inferior vena cava Apex Anterior view © 2016 Pearson Education, Ltd. Chambers and Associated Great Vessels Four chambers of the heart Atria: the receiving chambers – Small, thin-walled chambers; contribute little to propulsion of blood – Auricles: appendages that increase atrial volume – Right atrium: receives deoxygenated blood from body Three veins empty into right atrium: – Superior vena cava: returns blood from body regions above the diaphragm – Inferior vena cava: returns blood from body regions below the diaphragm – Coronary sinus: returns blood from coronary veins – Left atrium: receives oxygenated blood from lungs Pectinate muscles found only in auricles Four pulmonary veins return blood from lungs © 2016 Pearson Education, Ltd. Chambers and Associated Great Vessels (cont.) Ventricles: the discharging chambers – Make up most of the volume of heart – Thicker walls than atria – Actual pumps of heart – Right ventricle Pumps blood into pulmonary trunk – Left ventricle Pumps blood into aorta (largest artery in body) © 2016 Pearson Education, Ltd. 18.2 Heart Valves Ensure unidirectional blood flow through heart Open and close in response to pressure changes Two major types of valves – Atrioventricular valves located between atria and ventricles – Semilunar valves located between ventricles and major arteries © 2016 Pearson Education, Ltd. Atrioventricular (AV) Valves Two atrioventricular (AV) valves prevent backflow into atria when ventricles contract – Tricuspid valve (right AV valve): made up of three cusps and lies between right atria and ventricle – Mitral valve (left AV valve, bicuspid valve): made up of two cusps and lies between left atria and ventricle – Chordae tendineae: anchor cusps of AV valves to papillary muscles that function to: Hold valve flaps in closed position Prevent flaps from everting back into atria 1 Blood returning to the Direction of heart fills atria, pressing blood flow against the AV valves. The increased pressure forces Atrium AV valves open. Cusp of 2 As ventricles fill, AV valve atrioventricular flaps hang limply into ventricles. valve (open) Chordae tendineae 3 Atria contract, forcing additional blood into ventricles. Papillary Ventricle muscle AV valves © 2016 Pearson open; Education, Ltd. atrial pressure greater than ventricular pressure Semilunar (SL) valves Two semilunar (SL) valves prevent backflow from major arteries back into ventricles – Open and close in response to pressure changes – Each valve consists of three cusps that roughly resemble a half moon – Pulmonary semilunar valve: located between right ventricle and pulmonary trunk – Aortic semilunar valve: located between left ventricle and aorta © 2016 Pearson Education, Ltd. Semilunar (SL) valves © 2016 Pearson Education, Ltd. Clinical – Homeostatic Imbalance 18.2 Two conditions severely weaken heart: – Incompetent valve Blood backflows so heart repumps same blood over and over – Valvular stenosis Stiff flaps that constrict opening Heart needs to exert more force to pump blood Defective valve can be replaced with mechanical, animal, or cadaver valve © 2016 Pearson Education, Ltd. 18.3 Pathway of Blood Through Heart Right side of the heart – Superior vena cava (SVC), inferior vena cava (IVC), and coronary sinus → – Right atrium → – Tricuspid valve → – Right ventricle → – Pulmonary semilunar valve → – Pulmonary trunk → – Pulmonary arteries → – Lungs © 2016 Pearson Education, Ltd. 18.3 Pathway of Blood Through Heart Left side of the heart – Four pulmonary veins → – Left atrium → – Mitral valve → – Left ventricle → – Aortic semilunar valve → – Aorta → – Systemic circulation © 2016 Pearson Education, Ltd. Focus Figure 18.1 The heart is a double pump, each side supplying its own circuit. Tricuspid Pulmonary Oxygen-poor blood Superior vena cava (SVC) Right valve Right semilunar valve Pulmonary Oxygen-rich blood Inferior vena cava (IVC) atrium ventricle trunk Coronary sinus Pulmonary arteries SVC Coronary sinus Pulmonary trunk Right Tricuspid atrium Pulmonary valve semilunar valve Right IVC ventricle Oxygen-poor blood is carried Oxygen-poor blood in two pulmonary arteries to To heart returns from the body the lungs (pulmonary circuit) To lungs tissues back to the heart. to be oxygenated. Systemic Pulmonary capillaries capillaries Oxygen-rich blood is Oxygen-rich blood To body delivered to the body returns to the heart via To heart tissues (systemic circuit). the four pulmonary veins. Aorta Pulmonary veins Aortic Left semilunar Mitral valve atrium valve Left ventricle Aortic Mitral semilunar valve Left valve Left Four Aorta ventricle atrium pulmonary veins © 2016 Pearson Education, Ltd. 18.3 Pathway of Blood Through Heart Equal volumes of blood are pumped to pulmonary and systemic circuits Pulmonary circuit is short, low-pressure circulation Systemic circuit is long, high-friction circulation Anatomy of ventricles reflects differences – Left ventricle walls are 3× thicker than right Pumps with greater pressure © 2016 Pearson Education, Ltd. Coronary Circulation Coronary circulation – Functional blood supply to heart muscle itself – Shortest circulation in body – Delivered when heart is relaxed – Left ventricle receives most of coronary blood supply Coronary arteries – Both left and right coronary arteries arise from base of aorta and supply arterial blood to heart – Both encircle heart in coronary sulcus – Branching of coronary arteries varies among individuals – Arteries contain many anastomoses (junctions) Provide additional routes for blood delivery Cannot compensate for coronary artery occlusion – Heart receives 1/20th of body’s blood supply © 2016 Pearson Education, Ltd. Clinical – Homeostatic Imbalance 18.3 Angina pectoris – Thoracic pain caused by fleeting deficiency in blood delivery to myocardium – Cells are weakened Myocardial infarction (heart attack) – Prolonged coronary blockage – Areas of cell death are repaired with noncontractile scar tissue © 2016 Pearson Education, Ltd. Cardiovascular System Learning Objectives: Cardiovascular System Physiology of cardiac muscle contraction List the parts of the electrical conduction system of the heart in sequence, beginning at the sinoatrial (SA) node, and explain how the electrical conduction system functions. List the waveforms and segments of a typical electrocardiogram (ECG or EKG), and explain the electrical events represented by each waveform or segment. Define cardiac cycle, systole, and diastole. Diagram or describe the atrial and ventricular events of the cardiac cycle, beginning with atrial and ventricular diastole. Describe atrioventricular (AV) and semilunar (SL) valve position (open/closed) and the direction of blood flow during ventricular filling, isovolumetric ventricular contraction, ventricular ejection, and isovolumetric ventricular relaxation. Diagram or describe the relationships among the left atrial and ventricular pressure and volume curves, heart sounds, and the electrocardiogram during one cardiac cycle (the Wiggers diagram). Setting the Basic Rhythm: The Intrinsic Conduction System Sequence of excitation – Cardiac pacemaker cells pass impulses, in following order, across heart in ∼0.22 seconds 1. Sinoatrial node → 2. Atrioventricular node → 3. Atrioventricular bundle → 4. Right and left bundle branches → 5. Subendocardial conducting network (Purkinje fibers) © 2016 Pearson Education, Ltd. Figure 18.13 Intrinsic cardiac conduction system and action potential succession during one heartbeat. Slide 6 Superior vena cava Right atrium Pacemaker potential 1 The sinoatrial (SA) node (pacemaker) SA node generates impulses. Internodal pathway 2 The impulses Left atrium pause (0.1 s) at the atrioventricular Atrial muscle (AV) node. 3 The Subendocardial atrioventricular conducting (AV) bundle network AV node connects the atria (Purkinje fibers) to the ventricles. Ventricular 4 The bundle branches Pacemaker muscle conduct the impulses Inter- Plateau through the ventricular potential interventricular septum. septum 5 The subendocardial conducting network depolarizes the contractile 0 200 400 600 cells of both ventricles. Milliseconds Anatomy of the intrinsic conduction system showing the sequence Comparison of action potential shape of electrical excitation at various locations © 2016 Pearson Education, Ltd. Clinical – Homeostatic Imbalance 18.4 Defects in intrinsic conduction system may cause: – Arrhythmias: irregular heart rhythms – Uncoordinated atrial and ventricular contractions – Fibrillation: rapid, irregular contractions Heart becomes useless for pumping blood, causing circulation to cease; may result in brain death Treatment: defibrillation interrupts chaotic twitching, giving heart “clean slate” to start regular, normal depolarizations © 2016 Pearson Education, Ltd. Clinical – Homeostatic Imbalance 18.4 To reach ventricles, impulse must pass through AV node If AV node is defective, may cause a heart block – Few impulses (partial block) or no impulses (total block) reach ventricles – Ventricles beat at their own intrinsic rate Too slow to maintain adequate circulation – Treatment: artificial pacemaker, which recouples atria and ventricles © 2016 Pearson Education, Ltd. Modifying the Basic Rhthym: Extrinsic Innervation of the Heart Heartbeat modified by ANS via cardiac centers in medulla oblongata – Cardioacceleratory center: sends signals through sympathetic trunk to increase both rate and force Stimulates SA and AV nodes, heart muscle, and coronary arteries – Cardioinhibitory center: parasympathetic signals via vagus nerve to decrease rate Inhibits SA and AV nodes via vagus nerves © 2016 Pearson Education, Ltd. Figure 18.14 Autonomic innervation of the heart. The vagus nerve Dorsal motor nucleus (parasympathetic) of vagus decreases heart rate. Cardioinhibitory center Cardioacceleratory center Medulla oblongata Sympathetic trunk ganglion Thoracic spinal cord Sympathetic trunk Sympathetic cardiac nerves increase heart rate and force of contraction. AV node SA node Parasympathetic neurons Sympathetic neurons Interneurons © 2016 Pearson Education, Ltd. Action Potentials of Contractile Cardiac Muscle Cells Contractile muscle fibers make up bulk of heart and are responsible for pumping action – Different from skeletal muscle contraction; cardiac muscle action potentials have plateau Steps involved in AP: 1. Depolarization opens fast voltage-gated Na+ channels; Na+ enters cell Positive feedback influx of Na+ causes rising phase of AP (from −90 mV to +30 mV) 2. Depolarization by Na+ also opens slow Ca2+ channels At +30 mV, Na+ channels close, but slow Ca2+ channels remain open, prolonging depolarization – Seen as a plateau 3. After about 200 ms, slow Ca2+ channels are closed, and voltage-gated K+ channels are open Rapid efflux of K+ repolarizes cell to RMP Ca2+ is pumped both back into SR and out of cell into extracellular space © 2016 Pearson Education, Ltd. Figure 18.15 The action potential of contractile cardiac muscle cells. Slide 4 Action 1 Depolarization is due to Na+ influx potential through fast voltage-gated Na+ channels. A positive feedback cycle rapidly opens 20 Plateau many Na+ channels, reversing the membrane potential. Channel inactivation Membrane potential (mV) 2 ends this phase. 0 Tension development (contraction) 2 Plateau phase is due to Ca2+ influx Tension (g) −20 through slow Ca2+ channels. This keeps 1 3 the cell depolarized because most K+ −40 channels are closed. −60 Absolute 3 Repolarization is due to Ca2+ refractory channels inactivating and K+ channels −80 period opening. This allows K+ efflux, which brings the membrane potential back to its resting voltage. 0 150 300 Time (ms) © 2016 Pearson Education, Ltd. Electrocardiography Electrocardiograph can detect electrical currents generated by heart Electrocardiogram (ECG or EKG) is a graphic recording of electrical activity – Composite of all action potentials at given time; not a tracing of a single AP – Electrodes are placed at various points on body to measure voltage differences 12 lead ECG is most typical Main features: – P wave: depolarization of SA node and atria – QRS complex: ventricular depolarization and atrial repolarization – T wave: ventricular repolarization – P-R interval: beginning of atrial excitation to beginning of ventricular excitation – S-T segment: entire ventricular myocardium depolarized – Q-T interval: beginning of ventricular depolarization through ventricular repolarization © 2016 Pearson Education, Ltd. Figure 18.16 An electrocardiogram (ECG) tracing. QRS complex R Ventricular depolarization Atrial Ventricular depolarization repolarization Sinoatrial P T node Atrioventricular node Q P-R S-T Interval Segment S Q-T Interval 0 0.2 0.4 0.6 0.8 Time (s) © 2016 Pearson Education, Ltd. Figure 18.17 The sequence of depolarization and repolarization of the heart related to the deflection waves of an ECG tracing. Slide 7 SA node R P T Q S 1 Atrial depolarization, initiated by the SA node, causes the P wave. AV node R P T Q S 2 With atrial depolarization complete, the impulse is delayed at the AV node. R P T Q S 3 Ventricular depolarization begins at apex, causing the QRS complex. Atrial repolarization occurs. R P T Q S 4 Ventricular depolarization is complete. R P T Q S 5 Ventricular repolarization begins at apex, causing the T wave. R P T Q S Depolarization 6 Ventricular repolarization is complete. Repolarization © 2016 Pearson Education, Ltd. Clinical – Homeostatic Imbalance 18.5 Changes in patterns or timing of ECG may reveal diseased or damaged heart, or problems with heart’s conduction system Problems that can be detected: – Enlarged R waves may indicate enlarged ventricles – Elevated or depressed S-T segment indicates cardiac ischemia Problems that can be detected: (cont.) – Prolonged Q-T interval reveals a repolarization abnormality that increases risk of ventricular arrhythmias – Junctional blocks, blocks, flutters, and fibrillations are also detected on ECG © 2016 Pearson Education, Ltd. Figure 18.19 Normal and abnormal ECG tracings. Normal sinus rhythm. Junctional rhythm. The SA node is nonfunctional, P waves are absent, and the AV node paces the heart at 40–60 beats/min. Second-degree heart block. Some P waves are not conducted through the AV node; hence more P than QRS waves are seen. In this tracing, the ratio of P waves to QRS waves is mostly 2:1. © 2013 Pearson Ventricular fibrillation. These chaotic, grossly irregular ECG Education, Inc. deflections are seen in acute heart attack and electrical shock. Figure 20–13 Cardiac Arrhythmias. Premature Atrial Contractions (PACs) Premature atrial contractions (PACs) increase the incidence of PACs, presumably often occur in healthy individuals. In a PAC, by increasing the permeabilities of the SA the normal atrial rhythm is momentarily pacemakers. The impulse spreads along the P P P interrupted by a “surprise” atrial contraction. conduction pathway, and a normal ventricu- Stress, caffeine, and various drugs may lar contraction follows the atrial beat. Paroxysmal Atrial Tachycardia (PAT) In paroxysmal (par-ok-SIZ-mal) atrial tachycardia, or PAT, a premature atrial contraction triggers a flurry of atrial activity. P P P P P P The ventricles are still able to keep pace, and the heart rate jumps to about 180 beats per minute. Atrial Fibrillation (AF) During atrial fibrillation (fib-ri-LĀ-shun), are now nonfunctional, their contribution the impulses move over the atrial surface at to ventricular end-diastolic volume (the rates of perhaps 500 beats per minute. The maximum amount of blood the ventricles atrial wall quivers instead of producing an can hold at the end of atrial contraction) is organized contraction. The ventricular rate so small that the condition may go cannot follow the atrial rate and may remain unnoticed in older individuals. within normal limits. Even though the atria Premature Ventricular Contractions (PVCs) Premature ventricular contractions called an ectopic pacemaker. The (PVCs) occur when a Purkinje cell or frequency of PVCs can be increased by ventricular myocardial cell depolarizes to exposure to epinephrine, to other P T P T P T threshold and triggers a premature stimulatory drugs, or to ionic changes contraction. Single PVCs are common and that depolarize cardiac muscle plasma not dangerous. The cell responsible is membranes. Ventricular Tachycardia (VT) Ventricular tachycardia is defined as four or more PVCs without intervening normal beats. It is also known as VT or P V-tach. Multiple PVCs and VT may indicate that serious cardiac problems exist. Ventricular Fibrillation (VF) Ventricular fibrillation (VF) is respon- sible for the condition known as cardiac arrest. VF is rapidly fatal, because the ventricles quiver and stop pumping blood. 15 © 2018 Pearson Education, Ltd. Heart Sounds Two sounds (lub-dup) associated with closing of heart valves – First sound is closing of AV valves at beginning of ventricular systole – Second sound is closing of SL valves at beginning of ventricular diastole – Pause between lub-dups indicates heart relaxation Mitral valve closes slightly before tricuspid, and aortic closes slightly before pulmonary valve – Differences allow auscultation of each valve when stethoscope is placed in four different regions © 2016 Pearson Education, Ltd. Figure 18.20 Areas of the thoracic surface where the sounds of individual valves are heard most clearly. Aortic valve sounds heard in 2nd intercostal space at right sternal margin Pulmonary valve sounds heard in 2nd intercostal space at left sternal margin Mitral valve sounds heard over heart apex (in 5th intercostal space) in line with middle of clavicle Tricuspid valve sounds typically heard in right sternal margin of 5th intercostal space © 2016 Pearson Education, Ltd. Figure 20–18b Heart Sounds. 120 Semilunar Semilunar valves open valves close 90 Pressure (mm Hg) 60 Left ventricle Left AV valves AV valves 30 atrium close open 0 S1 S2 S4 S3 S4 Heart sounds “Lubb” “Dupp” b The relationship between heart sounds and key events in the cardiac cycle 18 © 2018 Pearson Education, Ltd. Clinical – Homeostatic Imbalance 18.6 Heart murmurs: abnormal heart sounds heard when blood hits obstructions Usually indicate valve problems – Incompetent (or insufficient) valve: fails to close completely, allowing backflow of blood Causes swishing sound as blood regurgitates backward from ventricle into atria – Stenotic valve: fails to open completely, restricting blood flow through valve Causes high-pitched sound or clicking as blood is forced through narrow valve © 2016 Pearson Education, Ltd. 18.6 Mechanical Events of Heart Systole: period of heart contraction Diastole: period of heart relaxation Cardiac cycle: blood flow through heart during one complete heartbeat – Atrial systole and diastole are followed by ventricular systole and diastole – Cycle represents series of pressure and blood volume changes – Mechanical events follow electrical events seen on ECG Three phases of the cardiac cycle (following left side, starting with total relaxation) © 2016 Pearson Education, Ltd. 18.6 Mechanical Events of Heart 1. Ventricular filling: mid-to-late diastole Pressure is low; 80% of blood passively flows from atria through open AV valves into ventricles from atria (SL valves closed) Atrial depolarization triggers atrial systole (P wave), atria contract, pushing remaining 20% of blood into ventricle – End diastolic volume (EDV): volume of blood in each ventricle at end of ventricular diastole Depolarization spreads to ventricles (QRS wave) Atria finish contracting and return to diastole while ventricles begin systole © 2016 Pearson Education, Ltd. 18.6 Mechanical Events of Heart 2. Ventricular systole Atria relax; ventricles begin to contract Rising ventricular pressure causes closing of AV valves Two phases 2a: Isovolumetric contraction phase: all valves are closed 2b: Ejection phase: ventricular pressure exceeds pressure in large arteries, forcing SL valves open » Pressure in aorta around 120 mm Hg End systolic volume (ESV): volume of blood remaining in each ventricle after systole © 2016 Pearson Education, Ltd. 18.6 Mechanical Events of Heart 3. Isovolumetric relaxation: early diastole Following ventricular repolarization (T wave), ventricles are relaxed; atria are relaxed and filling Backflow of blood in aorta and pulmonary trunk closes SL valves – Causes dicrotic notch (brief rise in aortic pressure as blood rebounds off closed valve) – Ventricles are totally closed chambers (isovolumetric) When atrial pressure exceeds ventricular pressure, AV valves open; cycle begins again © 2016 Pearson Education, Ltd. Figure 18.19 Summary of events during the cardiac cycle. Left heart QRS P T P Electrocardiogram 1st 2nd Heart sounds Dicrotic notch 120 Pressure (mm Hg) 80 Aorta Left ventricle 40 Atrial systole Left atrium 0 120 volume (ml) Ventricular EDV SV 50 ESV Atrioventricular valves Open Closed Open Aortic and pulmonary valves Closed Open Closed Phase 1 2a 2b 3 1 Left atrium Right atrium Left ventricle Right ventricle Ventricular Atrial Isovolumetric Ventricular Isovolumetric Ventricular filling contraction contraction phase ejection phase relaxation filling 1 2a 2b 3 Ventricular filling Ventricular systole Early diastole (mid-to-late diastole) (atria in diastole) © 2016 Pearson Education, Ltd. Cardiovascular System Learning Objectives: Cardiovascular System Anatomy and functional roles of the different types of blood vessels Name the different types of vessels found in the circulatory system, and briefly relate the structural features to the functions for each major vessel type. Consider the impact of vessel characteristics on flow, pressure and resistance. Define vasoconstriction and vasodilation. Part 1 Blood Vessel Structure and Function Blood vessels: delivery system of dynamic structures that begins and ends at heart – Work with lymphatic system to circulate fluids Arteries: carry blood away from heart; oxygenated except for pulmonary circulation and umbilical vessels of fetus Capillaries: direct contact with tissue cells; directly serve cellular needs Veins: carry blood toward heart; deoxygenated except for pulmonary circulation and umbilical vessels of fetus © 2016 Pearson Education, Ltd. Figure 19.1 The relationship of blood vessels to each other and to lymphatic vessels. Venous system Arterial system Large veins Heart (capacitance vessels) Elastic Large arteries lymphatic (conducting vessels arteries) Lymph node Muscular arteries Lymphatic (distributing system arteries) Small veins (capacitance vessels) Arteriovenous anastomosis Lymphatic capillaries Sinusoid Arterioles (resistance vessels) Terminal Postcapillary arteriole venule Thoroughfare Capillaries Precapillary Metarteriole channel (exchange sphincter vessels) © 2016 Pearson Education, Ltd. Blood Vessel Structure and Function Structure Functions Arteries The walls (outer structure) of arteries Transport blood away from the contain smooth muscle fibre that contract heart and relax under the instructions of the Transport oxygenated blood only sympathetic nervous system. (except in the case of the pulmonary artery). Arterioles Arterioles are tiny branches of arteries Transport blood from arteries to that lead to capillaries. These are also capillaries under the control of the sympathetic Arterioles are the main regulators nervous system, and constrict and dialate, of blood flow and pressure. to regulate blood flow. Capillaries Capillaries are tiny (extremely narrow) Function is to supply the tissues of blood vessels, of approximately 5-20 the body with the components of micro-metres blood, and (carried by the blood), (one micro-metre = 0.000001metre) and also to remove waste from diameter. the surrounding cells... as There are networks of capillaries in most opposed to simply moving the of the organs and tissues of the body. blood around the body (in the These capillaries are supplied with blood case of other blood vessels) by arterioles and drained by venules. Exchange of oxygen, carbon Capillary walls are only one cell thick (see dioxide, water, salts, etc., diagram), which permits exchanges of between the blood and the material between the contents of the surrounding body tissues. capillary and the surrounding tissue. Veins The walls (outer structure) of veins consist Transport blood towards the of three layers of tissues that are thinner heart. and less elastic than the corresponding Transport deoxygenated blood layers of aerteries. only (except in the case of the Veins include valves that aid the return of pulmonary vein). blood to the heart by preventing blood from flowing in the reverse direction. © 2016 Pearson Education, Ltd. Physiology of Circulation Flow, Pressure, and Resistance Definition of Terms Blood flow: volume of blood flowing through vessel, organ, or entire circulation in given period – Measured in ml/min, it is equivalent to cardiac output (CO) for entire vascular system – Overall is relatively constant when at rest, but at any given moment, varies at individual organ level, based on needs Blood pressure (BP): force per unit area exerted on wall of blood vessel by blood – Expressed in mm Hg – Measured as systemic arterial BP in large arteries near heart – Pressure gradient provides driving force that keeps blood moving from higher- to lower-pressure areas © 2016 Pearson Education, Ltd. Definition of Terms (cont.) Resistance (peripheral resistance): opposition to flow – Measurement of amount of friction blood encounters with vessel walls, generally in peripheral (systemic) circulation – Three important sources of resistance Blood viscosity Total blood vessel length Blood vessel diameter © 2016 Pearson Education, Ltd. Definition of Terms (cont.) – Blood viscosity The thickness or “stickiness” of blood due to formed elements and plasma proteins – The greater the viscosity, the less easily molecules are able to slide past each other Increased viscosity equals increased resistance – Total blood vessel length The longer the vessel, the greater the resistance encountered – Blood vessel diameter Has greatest influence on resistance Frequent changes alter peripheral resistance Viscosity and blood vessel length are relatively constant Resistance varies inversely with fourth power of vessel radius – If radius increases, resistance decreases, and vice-versa © 2016 Pearson Education, Ltd. 19.9 Control of Blood Flow Tissue perfusion: blood flow through body tissues; involved in: 1. Delivery of O2 and nutrients to, and removal of wastes from, tissue cells 2. Gas exchange (lungs) 3. Absorption of nutrients (digestive tract) 4. Urine formation (kidneys) Rate of flow is precisely right amount to provide proper function to that tissue or organ © 2016 Pearson Education, Ltd. 19.9 Control of Blood Flow – Example: redistribution of blood during exercise At rest, skeletal muscles receive about 20% of total blood in body, but during exercise, skeletal muscle can receive over 70% of blood Intrinsic controls: skeletal muscle arterioles dilate, increasing blood flow to muscle Extrinsic controls decrease blood flow to other organs such as kidneys and digestive organs – MAP is maintained despite dilation of skeletal muscle arterioles © 2016 Pearson Education, Ltd. Figure 19.13 Distribution of blood flow at rest and during strenuous exercise. 750 750 Brain 750 12,500 Heart 250 Skeletal 1200 muscles Skin 500 Kidneys 1100 Abdomen 1400 1900 Other 600 Total blood 600 flow at rest 600 5800 ml/min 400 Total blood flow during strenuous exercise 17,500 ml/min Cardiovascular System Learning Objectives: Cardiovascular System Regulation of cardiac output (CO), stroke volume (SV), and heart rate (HR) Name the different types of vessels found in the circulatory system, and briefly relate the structural features to the functions for each major vessel type. Consider the impact of vessel characteristics on flow. Define cardiac output (CO) and state its units of measurement. Explain how to calculate cardiac output (CO), given stroke volume (SV) and heart rate (HR). Predict how changes in heart rate (HR) and/or stroke volume (SV) affect cardiac output (CO). Explain how venous return, preload, and afterload each affect end diastolic volume (EDV), end systolic volume (ESV), and stroke volume (SV). Describe the role of the autonomic nervous system in the regulation of cardiac output. 18.7 Regulation of Pumping Cardiac output: amount of blood pumped out by each ventricle in 1 minute CO = heart rate (HR) × stroke volume (SV) HR = number of beats per minute Stroke volume: volume of blood pumped out by one ventricle with each beat – Correlates with force of contraction At rest: CO (ml/min) = HR (75 beats/min) × SV (70 ml/beat) = 5.25 L/min CO is affected by factors leading to: – Regulation of stroke volume – Regulation of heart rates © 2016 Pearson Education, Ltd. Regulation of Stroke Volume Mathematically: SV = EDV − ESV – EDV is affected by length of ventricular diastole and venous pressure (∼120 ml/beat) – ESV is affected by arterial BP and force of ventricular contraction (∼50 ml/beat) – Normal SV = 120 ml − 50 ml = 70 ml/beat Three main factors that affect SV: – 1. Preload – 2. Contractility – 3. Afterload © 2016 Pearson Education, Ltd. Regulation of Stroke Volume (cont.) 1. Preload: degree of stretch of heart muscle – Preload: degree to which cardiac muscle cells are stretched just before they contract Changes in preload cause changes in SV – Affects EDV – Relationship between preload and SV called Frank-Starling law of the heart – Most important factor in preload stretching of cardiac muscle is venous return—amount of blood returning to heart Slow heartbeat and exercise increase venous return Increased venous return distends (stretches) ventricles and increases contraction force Venous → EDV → SV → CO Return Frank-Starling Law © 2016 Pearson Education, Ltd. Regulation of Stroke Volume (cont.) 2. Contractility – Contractile strength at given muscle length Independent of muscle stretch and EDV – Increased contractility lowers ESV; caused by: Sympathetic epinephrine release stimulates increased Ca2+ influx, leading to more cross bridge formations Positive inotropic agents increase contractility – Thyroxine, glucagon, epinephrine, digitalis, high extracellular Ca2+ – Decreased by negative inotropic agents Acidosis (excess H+), increased extracellular K+, calcium channel blockers © 2016 Pearson Education, Ltd. Regulation of Stroke Volume (cont.) 3. Afterload: back pressure exerted by arterial blood – Afterload is pressure that ventricles must overcome to eject blood Back pressure from arterial blood pushing on SL valves is major pressure – Aortic pressure is around 80 mm Hg – Pulmonary trunk pressure is around 10 mm Hg – Hypertension increases afterload, resulting in increased ESV and reduced SV © 2016 Pearson Education, Ltd. Figure 18.21 Factors involved in determining cardiac output. Exercise (by Ventricular Bloodborne CNS output in sympathetic activity, filling time (due epinephrine, response to exercise, skeletal muscle and to heart rate) thyroxine, fright, anxiety, or respiratory pumps; excess Ca2+ blood pressure see Chapter 19) Venous Sympathetic Parasympathetic Contractility return activity activity EDV ESV (preload) Stroke volume (SV) Heart rate (HR) Initial stimulus Physiological response Cardiac output (CO = SV × HR) Result © 2016 Pearson Education, Ltd. Regulation of Heart Rate Heart rate can be regulated by: – Autonomic nervous system – Chemicals Autonomic nervous system regulation of heart rate – Sympathetic nervous system can be activated by emotional or physical stressors – Norepinephrine is released and binds to β1-adrenergic receptors on heart, causing: Pacemaker to fire more rapidly, increasing HR – EDV decreased because of decreased fill time Increased contractility – ESV decreased because of increased volume of ejected blood – Parasympathetic nervous system opposes sympathetic effects Acetylcholine hyperpolarizes pacemaker cells by opening K+ channels, which slows HR © 2016 Pearson Education, Ltd. Regulation of Heart Rate (cont.) Chemical regulation of heart rate – Hormones Epinephrine from adrenal medulla increases heart rate and contractility Thyroxine increases heart rate; enhances effects of norepinephrine and epinephrine – Ions Intra- and extracellular ion concentrations (e.g., Ca2+ and K+) must be maintained for normal heart function – Imbalances are very dangerous to heart © 2016 Pearson Education, Ltd. Clinical – Homeostatic Imbalance 18.7 Hypocalcemia: depresses heart Hypercalcemia: increases HR and contractility Hyperkalemia: alters electrical activity, which can lead to heart block and cardiac arrest Hypokalemia: results in feeble heartbeat; arrhythmias © 2016 Pearson Education, Ltd. Cardiovascular System Learning Objectives: Cardiovascular System Blood pressure and its functional interrelationships with cardiac output (CO), peripheral resistance, and hemodynamics Define total peripheral resistance (TPR), compare the relative contributions of systemic arteries, arterioles, capillaries, and veins to TPR, and identify which vessels are the primary site of variable (controlled) resistance to flow. Write and explain the equation relating mean arterial pressure (MAP) to cardiac output (CO) and total peripheral resistance (TPR). Predict and describe how mean arterial pressure (MAP) is affected by changes in total peripheral resistance (TPR), cardiac output (CO), heart rate (HR) and stroke volume (SV). Diagram or describe the anatomical components and steps of the baroreceptor reflex and explain how this reflex helps maintain blood pressure homeostasis when blood pressure changes. Predict the baroreceptor reflex response to a decrease in arterial blood pressure occurringupon standing (orthostatic hypotension). Describe the short-term and long term regulation of mean arterial pressure Arterial Blood Pressure Systolic pressure: pressure exerted in aorta during ventricular contraction – Left ventricle pumps blood into aorta, imparting kinetic energy that stretches aorta – Averages 120 mm Hg in normal adult Diastolic pressure: lowest level of aortic pressure when heart is at rest Pulse pressure: difference between systolic and diastolic pressure Pulse: throbbing of arteries due to difference in pulse pressures, which can be felt under skin Mean arterial pressure (MAP): pressure that propels blood to tissues – Heart spends more time in diastole, so not just a simple average of diastole and systole MAP is calculated by adding diastolic pressure + 1/3 pulse pressure – Example: BP = 120/80; Pulse Pressure = 120 − 80 = 40; so MAP = 80 + (1/3)x40 = 80 + 13 = 93 mm Hg Pulse pressure and MAP both decline with increasing distance from heart © 2016 Pearson Education, Ltd. Arterial Blood Pressure (cont.) Clinical monitoring of circulatory efficiency – Vital signs: pulse and blood pressure, along with respiratory rate and body temperature – Taking a pulse Radial pulse (taken at the wrist): most routinely used, but there are other clinically important pulse points Pressure points: areas where arteries are close to body surface – Can be compressed to stop blood flow in event of hemorrhaging © 2016 Pearson Education, Ltd. Figure 19.7 Body sites where the pulse is most easily palpated. Superficial temporal artery Facial artery Common carotid artery Brachial artery Radial artery Femoral artery Popliteal artery Posterior tibial artery Dorsalis pedis artery © 2016 Pearson Education, Ltd. Arterial Blood Pressure (cont.) – Measuring blood pressure Systemic arterial BP is measured indirectly by auscultatory methods using a sphygmomanometer 1. Wrap cuff around arm superior to elbow 2. Increase pressure in cuff until it exceeds systolic pressure in brachial artery 3. Pressure is released slowly, and examiner listens for sounds of Korotkoff with a stethoscope Systolic pressure: normally less than 120 mm Hg – Pressure when sounds first occur as blood starts to spurt through artery Diastolic pressure: normally less than 80 mm Hg – Pressure when sounds disappear because artery no longer constricted; blood flowing freely © 2016 Pearson Education, Ltd. 19.8 Regulation of Blood Pressure Maintaining blood pressure requires cooperation of heart, blood vessels, and kidneys – All supervised by brain Three main factors regulating blood pressure – Cardiac output (CO) – Peripheral resistance (PR) – Blood volume Blood pressure varies directly with CO, PR, and blood volume © 2016 Pearson Education, Ltd. 19.8 Regulation of Blood Pressure Recall that CO = SV × HR, so if MAP = CO × PR, then MAP = SV × HR × PR Anything that increases SV, HR, or TPR will also increase MAP – SV is effected by venous return (EDV) – HR is maintained by medullary centers – PR (systemic resistance) is effected mostly by vessel diameter © 2016 Pearson Education, Ltd. Figure 19.9 Major factors determining MAP. Stroke Heart Diameter of Blood Blood volume rate blood vessels viscosity vessel length Cardiac output Peripheral resistance Mean arterial pressure (MAP) © 2016 Pearson Education, Ltd. 19.8 Regulation of Blood Pressure Factors can be affected by: – Short-term regulation: Neural mechanisms Vasomotor center Reflex Control- – Baroreceptor Reflexes – Chemoreceptor Reflexes Hormonal Control Higher order brain centers – Long-term regulation: renal controls © 2016 Pearson Education, Ltd. 21-3 Cardiovascular Regulation Neural mechanisms – Cardiovascular (CV) center of the medulla oblongata Consists of cardiac centers and vasomotor center – Cardiac centers Cardioacceleratory center increases cardiac output Cardioinhibitory center reduces cardiac output Vasomotor center – Control of vasoconstriction Controlled by adrenergic nerves (NE) Stimulate contraction in arteriole walls – Control of vasodilation Controlled by cholinergic nerves (NO) Relax smooth muscle 30 © 2018 Pearson Education, Ltd. Short-Term Regulation Baroreceptor reflexes – Located in carotid sinuses, aortic arch, and walls of large arteries of neck and thorax – If MAP is high: Increased blood pressure stimulates baroreceptors to increase input to vasomotor center Inhibits vasomotor and cardioacceleratory centers Stimulates cardioinhibitory center Decrease cardiac output Cause peripheral vasodilation Results in decreased blood pressure – When blood pressure falls, CV centers Increase cardiac output Cause peripheral vasoconstriction 19.8 Regulation of Blood Pressure What happends when you stand up? → blood pools in veins → ↓ venous return (↓EDV) → ↓ SV → ↓ CO → ↓ MAP → baroreceptors sense the decreased BP → ↑ sympathetic discharge → ↑ HR → ↑ contractility (SV) → ↑ arteriolar resistance (vasoconstriction) → ↑ CO & ↑ TPR → MAP returned to normal Figure 19.10 Baroreceptor reflexes that help maintain blood pressure homeostasis. Slide 6 3 Impulses from baroreceptors stimulate cardioinhibitory center (and inhibit cardioacceleratory center) and inhibit vasomotor center. 4a Sympathetic impulses to heart cause HR, contractility, and CO. 2 Baroreceptors in carotid sinuses and aortic arch are stimulated. 4b Rate of vasomotor impulses allows vasodilation, 5 CO and R causing R. return blood 1 Stimulus: pressure to Blood pressure homeostatic range. (arterial blood Homeostasis: Blood pressure in normal range pressure rises above normal range). 1 Stimulus: Blood pressure (arterial blood pressure falls below 4b Vasomotor normal range). 5 CO and R return blood fibers stimulate pressure to vasoconstriction, homeostatic causing R. range. 2 Baroreceptors in carotid sinuses and aortic arch are inhibited 4a Sympathetic impulses to heart Cause HR, contractility, and 3 Impulses from baroreceptors CO. activate cardioacceleratory center (and inhibit cardioinhibitory center) and stimulate vasomotor center. © 2016 Pearson Education, Ltd. Short-Term Regulation Chemoreceptor reflexes – Aortic arch and large arteries of neck detect increase in CO2, or drop in pH or O2 – Cause increased blood pressure by: Signaling cardioacceleratory center to increase CO Signaling vasomotor center to increase vasoconstriction © 2016 Pearson Education, Ltd. Short-Term Regulation Influence of higher brain centers – Reflexes that regulate BP are found in medulla – Hypothalamus and cerebral cortex can modify arterial pressure via relays to medulla – Hypothalamus increases blood pressure during stress – Hypothalamus mediates redistribution of blood flow during exercise and changes in body temperature © 2016 Pearson Education, Ltd. Short-Term Mechanisms: Hormonal Controls Hormones regulate BP in short term via changes in peripheral resistance or long term via changes in blood volume Adrenal medulla hormones – Epinephrine and norepinephrine from adrenal gland increase CO and vasoconstriction Angiotensin II stimulates vasoconstriction ADH: high levels can cause vasoconstriction Atrial natriuretic peptide decreases BP by antagonizing aldosterone, causing decreased blood volume © 2016 Pearson Education, Ltd. Long-Term Mechanisms: Renal Regulation Long-term mechanisms control BP by altering blood volume via kidneys Kidneys regulate arterial blood pressure by: 1. Direct renal mechanism 2. Indirect renal mechanism (renin-angiotensin- aldosterone) © 2016 Pearson Education, Ltd. Long-Term Mechanisms: Renal Regulation (cont.) Direct renal mechanism – Alters blood volume independently of hormones Increased BP or blood volume causes elimination of more urine, thus reducing BP Decreased BP or blood volume causes kidneys to conserve water, and BP rises © 2016 Pearson Education, Ltd. Figure 19.11 Direct and indirect (hormonal) mechanisms for renal control of blood pressure. Direct renal mechanism Indirect renal mechanism (renin-angiotensin-aldosterone) Initial stimulus Arterial pressure Arterial pressure Physiological response Result Inhibits baroreceptors Sympathetic nervous system activity Filtration by kidneys Angiotensinogen Renin release from kidneys Angiotensin I Angiotensin converting enzyme (ACE) Angiotensin II Urine formation ADH release by Thirst via Vasoconstriction; Adrenal cortex posterior pituitary hypothalamus peripheral resistance Secretes Aldosterone Blood volume Sodium reabsorption Water reabsorption Water intake by kidneys by kidneys Blood volume Mean arterial pressure Mean arterial pressure © 2016 Pearson Education, Ltd. Long-Term Mechanisms: Renal Regulation (cont.) Indirect mechanism – The renin-angiotensin-aldosterone mechanism Decreased arterial blood pressure causes release of renin from kidneys Renin enters blood and catalyzes conversion of angiotensinogen from liver to angiotensin I Angiotensin-converting enzyme, especially from lungs, converts angiotensin I to angiotensin II Angiotensin II acts in four ways to stabilize arterial BP and ECF: Stimulates aldosterone secretion Causes ADH release from posterior pituitary Triggers hypothalamic thirst center to drink more water Acts as a potent vasoconstrictor, directly increasing blood pressure © 2016 Pearson Education, Ltd. © 2016 Pearson Education, Ltd. Cardiovascular System Learning Objectives: Cardiovascular System Application of homeostatic mechanisms Provide specific examples to demonstrate how the cardiovascular system maintains blood pressure homeostasis in the body. Predictions related to disruption of homeostasis Given a factor or situation (e.g., left ventricular failure), predict the changes that could occur in the cardiovascular system and the consequences of those changes (i.e., given a cause, state a possible effect). Summary of Blood Pressure Regulation Goal of blood pressure regulation is to keep blood pressure high enough to provide adequate tissue perfusion, but not so high that blood vessels are damaged – Example: If BP to brain is too low, perfusion is inadequate, and person loses consciousness – If BP to brain is too high, person could have stroke © 2016 Pearson Education, Ltd. Figure 19.12 Factors that increase MAP. Activity of Release Fluid loss from Crisis stressors: Vasomotor tone; Dehydration, Body size muscular of ANPP hemorrhage, exercise, trauma, bloodborne high hematocrit pump and excessive body chemicals respiratory sweating temperature (epinephrine, pump NE, ADH, angiotensin II) Conservation Blood volume Blood pH of Na+ and Blood pressure O2 water by kidneys CO2 Blood Baroreceptors Chemoreceptors volume Venous Activation of vasomotor and cardio- return acceleratory centers in brain stem Diameter of Blood Blood vessel Stroke Heart blood vessels viscosity length volume rate Cardiac output Peripheral resistance Initial stimulus Physiological response Mean arterial pressure (MAP) Result © 2016 Pearson Education, Ltd. Homeostatic Imbalances in Blood Pressure (cont.) Circulatory shock – Condition where blood vessels inadequately fill and cannot circulate blood normally Inadequate blood flow cannot meet tissue needs – Hypovolemic shock results from large-scale blood loss – Vascular shock results from extreme vasodilation and decreased peripheral resistance – Cardiogenic shock results when an inefficient heart cannot sustain adequate circulation © 2016 Pearson Education, Ltd. Figure 19.18 Events and signs of hypovolemic shock. Initial stimulus Acute bleeding (or other events that reduce blood volume) leads to: Physiological response 1. Inadequate tissue perfusion Signs and symptoms resulting in O2 and nutrients to cells Result 2. Anaerobic metabolism by cells, so lactic acid accumulates 3. Movement of interstitial fluid into blood, so tissues dehydrate Chemoreceptors activated Baroreceptor firing reduced Hypothalamus activated Brain (by in blood pH) (by blood volume and pressure) (by blood pressure) Major effect Minor effect Respiratory centers Cardioacceleratory and Sympathetic nervous ADH Neurons activated vasomotor centers activated system activated released depressed by pH Intense vasoconstriction Heart rate (only heart and brain spared) Central nervous system depressed Renal blood flow Kidneys Renin released Adrenal cortex Angiotensin II produced in blood Aldosterone Kidneys retain Water released salt and water retention Rate and Tachycardia; Skin becomes Urine output Thirst Restlessness Coma depth of weak, thready cold, clammy, (early sign) (late sign) breathing pulse and cyanotic Blood pressure maintained; CO2 blown