Biology Chapter 11.2 Endocrine System PDF
Document Details
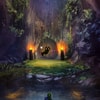
Uploaded by iiScholar
Arizona State University
Tags
Related
- The Endocrine System and Hormones PDF
- General Physiology Lecture - Endocrine System PDF
- Anatomy & Physiology for the Health Sciences II Endocrine System I PDF
- Vander's Human Physiology - The Endocrine System PDF
- Physiology Endocrine System Self-Assessment PDF
- Behavioral Biology Lecture 10: The Endocrine System (Part 2) PDF
Summary
This chapter from a biology textbook details the endocrine system and explains the different types of hormones, their effects, and the methods of regulation. It examines the complex interactions between the nervous and endocrine systems.
Full Transcript
Automatic ZoomActual SizePage Width100%50%75%100%125%150%200%300%400% Chapter 11: Endocrine System 383 Lesson 11.2 **Hormones** Introduction The endocrine system is comprised of various endocrine glands and organs throughout the body, which, in response to specific signals, produce and release...
Automatic ZoomActual SizePage Width100%50%75%100%125%150%200%300%400% Chapter 11: Endocrine System 383 Lesson 11.2 **Hormones** Introduction The endocrine system is comprised of various endocrine glands and organs throughout the body, which, in response to specific signals, produce and release hormones to maintain [homeostasis](javascript:void(0)). This lesson explores the production and release of important hormones in the body as well as the function and regulation of these hormones. Together with the nervous system (see Chapter 12), the endocrine system is responsible for signaling and maintaining homeostasis of other body systems. The endocrine system presents a unique way to synthesize and review information about other body systems. Therefore, some of the information in this lesson is a review or deeper exploration of information presented in other chapters, which are referenced throughout. Due to its role in integrating the functions of multiple body systems, the endocrine system is a particularly high-yield topic on the exam. Gaining a thorough understanding of the endocrine system facilitates an understanding of the required knowledge of many other systems as well. 11.2.01 Hypothalamic Hormones The **hypothalamus**, a forebrain structure located inferior to the [thalamus](javascript:void(0)), regulates the synthesis and secretion of multiple hormone classes. The hypothalamus both processes inputs from the brain\'s cerebral cortex and senses the plasma concentration of numerous hormones; therefore, the hypothalamus serves as the critical interface between the nervous and endocrine systems. In response to various inputs, the hypothalamus controls body-wide endocrine function by influencing activity within the [pituitary gland](javascript:void(0)) (also known as the hypophysis), an endocrine organ located inferior to the hypothalamus. The interface of the nervous and endocrine systems creates what are known as neuroendocrine pathways in the body. Neuroendocrine pathways are involved in the release of either hormones (from endocrine cells) or neurotransmitters and neurohormones (from neurons and neuroendocrine cells). An example of a neuroendocrine pathway is the stress response, depicted in Figure 11.12: 1.When stress is perceived, the hypothalamus sends electrical signals (ie, neural signals) via sympathetic neurons to the adrenal medulla. 2.The adrenal medulla releases epinephrine and norepinephrine (ie, neurohormones of the endocrine system) from neuroendocrine cells. 3.Epinephrine and norepinephrine are transported in the blood and bind receptors in specific body cells to cause responses to stress; for example, epinephrine binding specific receptors in the smooth muscle of blood vessels causes vasoconstriction and a subsequent increase in blood pressure. Chapter 11: Endocrine System 384 **Figure 11.12** The stress response is an example of a neuroendocrine pathway. The hypothalamus is able to exert control over the endocrine system through [peptide hormones](javascript:void(0)) known as releasing and inhibiting factors. These factors are examples of tropic hormones, or hormones that act to influence an endocrine response (ie, alter secretion of other hormones, see Concept 11.1.01). Hypothalamic tropic hormones act on the anterior pituitary as part of the hypothalamic-pituitary (hypothalamic-hypophyseal) control axis. The interaction of the hypothalamus and anterior pituitary is an example of a **portal system** in the body. In a portal system, blood circulates through [two capillary beds](javascript:void(0)) connected in series by small portal veins, rather than through only one capillary bed (see Concept 13.1.10). The hypothalamic-pituitary portal system allows hypothalamic hormones to remain concentrated as they travel a short distance to anterior pituitary target cells, rather than becoming diluted in the entire bloodstream. In this way, the hypothalamus and nearby anterior pituitary have a direct line of chemical communication. An example of communication via the hypothalamic-pituitary portal system is seen in the regulation of growth hormone (GH) release from the anterior pituitary. Growth hormone--releasing hormone (GHRH), a tropic hormone produced in the hypothalamus, travels via portal veins to the anterior pituitary, causing the anterior pituitary to release growth hormone, as shown in Figure 11.13. Chapter 11: Endocrine System 385 **Figure 11.13** The hypothalamic-pituitary portal system. Through the endocrine actions of releasing and inhibiting factors on the anterior pituitary, the hypothalamus promotes homeostasis in the body. As the serum level of a given hormone rises, that hormone inhibits the signaling pathway that promotes the synthesis and secretion of the hormone itself in a control process known as a [negative feedback loop](javascript:void(0)). Many negative feedback loops exist to ensure a constant appropriate level of hormones in the body. Thermoregulation, appetite and satiety, the stress response, body water content and blood pressure, metabolism, growth, and reproduction are all examples of processes governed by the hypothalamus. In addition to secreting tropic hormones to regulate the anterior pituitary (discussed in more detail in Concept 11.2.03), the hypothalamus produces hormones for storage in, and release from, the posterior pituitary (discussed further in Concept 11.2.02). 11.2.02 Posterior Pituitary Hormones The **pituitary gland** consists of the **anterior** and **posterior lobes**, both of which are derived from [embryonic ectodermal tissue](javascript:void(0)). The anterior pituitary is derived from epithelial cells of the developing roof of the mouth and contains typical glandular endocrine cells, whereas the posterior pituitary (neurohypophysis) is derived from ectodermal neural tissue in the developing brain and does *not* contain typical glandular endocrine cells. Therefore, hormones are not *produced* in the posterior pituitary; instead, the lobe is a storage site for hormones released from hypothalamic neurons (ie, **neurohormones**) rather than from typical glandular cells.  Chapter 11: Endocrine System 386 Stimulation of these hypothalamic neurons results in [exocytosis](javascript:void(0)) of neurosecretory vesicles containing the stored neurohormones from axon terminals located in the posterior pituitary. The exocytosed hormones are secreted into small vessels that carry blood away from the posterior pituitary, as illustrated in Figure 11.14. The two neurohormones stored and released from the posterior pituitary are the peptide hormones **antidiuretic hormone (ADH, vasopressin)** and **oxytocin**. **Figure 11.14** The posterior pituitary stores and releases hormones synthesized in the hypothalamus. ADH is involved in the regulation of body water content (see Lesson 16.2). Special stretch receptors monitor the degree of atrial stretching in the [heart](javascript:void(0)) (ie, volume of blood entering the atria) and transmit this information to the hypothalamus. In addition, the hypothalamus monitors changes in blood [osmolarity](javascript:void(0)). In response to changes in atrial stretching and blood osmolarity, the hypothalamus signals the posterior pituitary to adjust ADH secretion accordingly. When blood volume is reduced or blood osmolarity is increased, [ADH causes](javascript:void(0)) the insertion of aquaporins (ie, water channels) into [kidney nephron tubules](javascript:void(0)). Aquaporin insertion increases the permeability of the nephrons to water, thereby increasing the reabsorption of water into the bloodstream, which increases blood volume and decreases blood osmolarity to homeostatic levels. When blood volume increases or Chapter 11: Endocrine System 387 blood osmolarity decreases, less ADH is released to maintain homeostasis. The effect of ADH on body water content is summarized in Figure 11.15. **Figure 11.15** Actions of antidiuretic hormone. Oxytocin is released by the posterior pituitary in response to pressure against the cervix (ie, from the head of the fetus) during childbirth. Oxytocin release increases uterine contractions, further stimulating the release of oxytocin until parturition (Figure 11.16). This situation is one of the rare examples of [positive feedback](javascript:void(0)) in the body, in which a stimulus causes a response that further *increases* (rather than *decreases*, as in negative feedback) the initial stimulus. Oxytocin also targets mammary glands to stimulate milk ejection during breast feeding.  Chapter 11: Endocrine System 388 **Figure 11.16** Oxytocin works in a positive feedback loop just prior to parturition. 11.2.03 Anterior Pituitary Hormones The pituitary gland is a small structure located inside a bony cavity inferior to the hypothalamus at the brain\'s base. Two separate lobes of the pituitary gland exist: the anterior and posterior pituitary. The **anterior pituitary** (also known as the adenohypophysis) is made up of glandular endocrine tissue containing different cell types that synthesize and secrete several tropic peptide hormones (ie, hormones that produce endocrine effects). These tropic peptide hormones include adrenocorticotropic hormone (ACTH), follicle-stimulating hormone (FSH), luteinizing hormone (LH), and thyroid-stimulating hormone (TSH). The anterior pituitary also secretes several direct peptide hormones: β-endorphins, growth hormone (GH), and prolactin. More information about the posterior pituitary, which does not contain typical endocrine glandular cells, can be found in Concept 11.2.02. The synthesis and secretion of many anterior pituitary hormones are controlled by tropic neurohormones released by hypothalamic neurons (Figure 11.17). These neurohormones are secreted into the hypophyseal portal system, which allows direct chemical communication between the hypothalamus and the anterior pituitary (see Concept 11.2.01). Chapter 11: Endocrine System 389 **Figure 11.17** Anterior pituitary hormones. **ACTH** acts on the adrenal cortex to promote synthesis and secretion of glucocorticoids, steroid hormones that function to increase blood glucose and mediate the stress response (see Concept 11.2.04). Secretion of **corticotropin-releasing hormone (CRH)** by the hypothalamus promotes ACTH release as part of the hypothalamic-pituitary-adrenal pathway (HPA axis). Glucocorticoids inhibit secretion of CRH and ACTH. **FSH** and **LH** regulate reproductive function (see Concept 11.2.09) by serving as gonadotropic hormones (ie, hormones that regulate the endocrine activity of gonads \[ovaries, testes\]). FSH stimulates follicle maturation in ovaries and spermatogenesis in testes. LH stimulates estrogen production and triggers ovulation in ovaries and promotes spermatogenesis and testosterone synthesis in testes. **Gonadotropin-releasing hormone (GnRH)** produced by the hypothalamus promotes synthesis and secretion of FSH and LH by the anterior pituitary. Estrogens and testosterone suppress the release of GnRH, FSH, and LH.  Chapter 11: Endocrine System 390 **TSH** acts on the thyroid gland to stimulate production of the thyroid hormones triiodothyronine (T3) and thyroxine (T4). Thyroid hormones affect most body cells and function to increase the rate of cellular metabolism (see Concept 11.2.07) and other processes. The secretion of TSH is controlled by hypothalamic **thyrotropin-releasing hormone (TRH)** as part of the hypothalamic-pituitary-thyroid pathway (HPT axis), and thyroid hormones suppress the release of TRH and TSH. Table 11.1 summarizes the tropic hormones released by the anterior pituitary gland and the regulation of these hormones. **Table 11.1** Tropic hormones of the anterior pituitary. **Hormone** **Secretion stimulated by** **Secretion inhibited by** **Action** Adrenocorticotropic hormone (ACTH) Corticotropin-releasing hormone (CRH) Glucocorticoids Promotes glucocorticoid release by adrenal glands Follicle-stimulating hormone (FSH) Stimulates ovarian follicle maturation, testosterone synthesis, and spermatogenesis Luteinizing hormone (LH) Gonadotropin-releasing hormone (GnRH) Estrogens (low to moderate levels), testosterone Promotes estrogen production, ovulation, and testosterone synthesis Thyroid-stimulating hormone (TSH) Thyrotropin-releasing hormone (TRH) Thyroid hormone Stimulates thyroid gland to release thyroid hormones (T3, T4) **T3** = triiodothyronine; **T4** = thyroxine. **β-endorphins** act as [neurotransmitters](javascript:void(0)), binding opioid receptors in the brain to decrease pain perception and act as natural opiates (in contrast to exogenous opiate drugs \[eg, morphine, heroin\], which also bind opioid receptors). In addition, β-endorphins can cause feelings of euphoria; for example, these chemicals are thought to be partially responsible for the so-called runner\'s high experienced by athletes. β-endorphins may have additional roles, including functions in memory, learning, and thermoregulation. β-endorphins are co-secreted with ACTH and as such, are regulated in the same manner. **GH** regulates growth by promoting protein synthesis, fat utilization, and glucose uptake in many tissues. Secretion of GH is highest in childhood and remains elevated until mature height is reached in early adulthood. Even so, GH remains an important hormone in adults, with effects on processes other than growth (eg, metabolism, cell turnover). Hypothalamic **growth hormone--releasing hormone (GHRH)** stimulates GH release, whereas hypothalamic **somatostatin** inhibits GH release. Proper GH levels are particularly important in childhood: a GH deficit can lead to dwarfism, and excessive GH can lead to gigantism. **Prolactin** is released after parturition in response to nipple stimulation (ie, infant suckling) and promotes the mammary glands to produce and secrete milk (see Concept 10.4.04). However, milk ejection requires the posterior pituitary hormone oxytocin. Prior to parturition (ie, during pregnancy), high levels of estrogen and progesterone inhibit prolactin release. Hypothalamic **prolactin-inhibiting factor (PIF, dopamine)** decreases anterior pituitary prolactin secretion. Note that hypothalamic prolactin regulation is the opposite of other anterior pituitary hormones, whose secretion requires *release* of stimulatory hypothalamic hormones. In contrast, the hypothalamus Chapter 11: Endocrine System 391 must *not* release PIF (ie, an inhibitory hormone) for prolactin secretion to occur. Table 11.2 summarizes the direct hormones produced by the anterior pituitary and their regulation. **Table 11.2** Direct hormones produced by the anterior pituitary. **Hormone** **Secretion stimulated by** **Secretion inhibited by** **Action** β-endorphins Corticotropin-releasing hormone (CRH) Glucocorticoids Decrease pain perception, cause feelings of euphoria Growth hormone (GH) Growth hormone--releasing hormone (GHRH) Somatostatin Promotes growth in children and adults Prolactin Infant suckling Prolactin-inhibiting factor (PIF, dopamine), high estrogen and progesterone levels Stimulates lactation 11.2.04 Adrenal Hormones The capsule-covered **adrenal glands** are located atop each kidney. Each adrenal gland can be subdivided into two portions: an outer portion called the **adrenal cortex** and a central inner portion called the **adrenal medulla**. In addition to being separated anatomically, the two portions of the adrenal glands secrete different hormones (Figure 11.18). Adrenal hormones mediate the **stress response**, the physiological changes that allow the body to cope with and react to physical, social, and emotional stressors. Specifically, adrenal hormones promote the fight-or-flight response, influencing both cardiovascular function and energy metabolism (ie, cellular processes by which energy is stored or utilized), the latter by promoting energy utilization and inhibiting energy storage. **Figure 11.18** Adrenal gland anatomy and hormones. Chapter 11: Endocrine System 392 **Adrenal Cortex** The adrenal cortex is responsible for producing three classes of **corticosteroids** (ie, [steroid hormones](javascript:void(0)) produced by the adrenal cortex). The first corticosteroid class consists of **glucocorticoids**, including **cortisol** and **cortisone**. Glucocorticoids function to increase blood glucose by acting on the liver to increase the synthesis of glucose from other molecules (ie, [gluconeogenesis](javascript:void(0))) and promoting the breakdown of fats into fatty acids (ie, lipolysis) to [provide energy](javascript:void(0)) for gluconeogenesis. Both glucose and free fatty acids are forms of cellular energy that can be readily utilized. In addition to increasing blood glucose levels, glucocorticoids increase the breakdown of proteins to amino acids, which can be used to create new proteins or for ATP production. Glucocorticoids also have anti-inflammatory effects. Glucocorticoid secretion is regulated by the hypothalamic-pituitary-adrenal pathway (HPA axis), as discussed in Concept 11.2.03. The second corticosteroid class consists of **mineralocorticoids**, primarily **aldosterone**. Mineralocorticoids act to regulate blood pressure and blood volume. Blood filtration by kidney nephrons yields a fluid (ie, filtrate) that undergoes significant changes in volume and composition prior to excretion as urine (see Lesson 16.2). Most filtered sodium ions (Na+) and water are reabsorbed; however, Na+ and water reabsorption is subject to some degree of adjustment based on physiological conditions such as blood pressure and blood volume. Aldosterone secretion is regulated by the **renin-angiotensin system (RAS)**. Low blood pressure promotes the renal release of the enzyme renin, which catalyzes cleavage of the plasma protein angiotensinogen to form angiotensin I. The lungs release another enzyme, angiotensin-converting enzyme, which catalyzes the cleavage of angiotensin I to form angiotensin II. Angiotensin II increases blood pressure by inducing vasoconstriction and by promoting aldosterone release, as shown in Figure 11.19. Chapter 11: Endocrine System 393 **Figure 11.19** The renin-angiotensin system controls aldosterone release. [Aldosterone acts](javascript:void(0)) on nephron distal convoluted tubules and collecting ducts to promote Na+ reabsorption and potassium (K+) secretion. However, more Na+ is reabsorbed than K+ is secreted, generating an ion gradient between the filtrate and the interstitial fluid (ie, increases interstitial fluid osmolarity), causing water to be reabsorbed into the interstitial fluid (and eventually the bloodstream) via [osmosis](javascript:void(0)). As a result, blood volume and blood pressure increase, restoring homeostasis. The third corticosteroid class consists of **cortical sex hormones** known as **androgens** (sex steroids promoting male characteristics) and **estrogens** (sex steroids promoting female characteristics). Androgens stimulate sperm cell differentiation and development of masculine sexual traits, whereas estrogens stimulate endometrial growth and development of feminine sexual traits. Note that both androgens and estrogens are secreted by sex organs as well (see Concept 11.2.09). **Adrenal Medulla** The adrenal medulla releases the [catecholamines](javascript:void(0)) **epinephrine** and **norepinephrine**, amino acid--derived hormones that enable increased body mobility and promote rapid information processing under extreme stress. Catecholamine release is controlled by the [autonomic nervous system (ANS)](javascript:void(0)), which is composed of two branches with broadly [antagonistic effects](javascript:void(0)): the sympathetic nervous system (SNS) and the parasympathetic nervous system (PNS). Many tissues are innervated by both ANS branches; however, the adrenal medulla is innervated only by the SNS. Acetylcholine release by sympathetic neurons stimulates catecholamine release (Figure 11.20).  Chapter 11: Endocrine System 394 **Figure 11.20** Innervation of the adrenal medulla by the sympathetic nervous system. Catecholamines modulate the metabolism of glycogen, the polymer storage form of glucose. Low blood glucose levels (ie, hypoglycemia) promote catecholamine release. Along with [pancreatic glucagon](javascript:void(0)), these hormones promote [glycogenolysis](javascript:void(0)) (ie, breakdown of glycogen into glucose monomers). At the same time, catecholamines inhibit enzymes that mediate glycogen synthesis from glucose (ie, [glycogenesis](javascript:void(0))), thereby maintaining higher levels of free glucose in the blood. The response to hypoglycemia is depicted in Figure 11.21. Chapter 11: Endocrine System 395 **Figure 11.21** Low blood glucose leads to catecholamine release. Catecholamine release redirects blood flow via vasoconstriction (ie, narrowing of blood vessels) and vasodilation (ie, widening of blood vessels). During times of stress, vasoconstriction reduces blood supply to organs carrying out nonessential functions (eg, stomach, intestines, kidneys) to conserve oxygen and nutrients for organs necessary for immediate survival (eg, heart, skeletal muscle). Vasodilation increases blood flow to these organs necessary for immediate survival. Catecholamines can have opposing effects (ie, vasoconstriction or vasodilation) on blood vessels in different locations due to catecholamine ability to bind different receptor types. Binding of catecholamines to α-adrenergic receptors during times of stress leads to reduced blood flow to nonessential organs, and catecholamine binding to β-adrenergic receptors results in increased blood flow to essential organs, as shown in Figure 11.22.  Chapter 11: Endocrine System 396 **Figure 11.22** Catecholamine effects on blood vessels. In addition to effects on blood vessel diameter, catecholamines increase heart rate and cardiac muscle contractility, promoting increased blood flow to the brain, lungs, and skeletal muscles, and thereby allowing the processing and execution of responses to stressful stimuli. At the same time, catecholamines dilate airways (bronchioles) to increase respiratory function and oxygen delivery to necessary tissues. The complete stress response involves both catecholamines released by the adrenal medulla and glucocorticoids released by the adrenal cortex (Figure 11.23). Catecholamines mediate very quick responses to immediate stressors, whereas glucocorticoids mediate slower responses to long-term stressors. The presence of high glucocorticoid levels for extended periods of time can lead to negative effects (eg, immune system suppression). Chapter 11: Endocrine System 397 **Figure 11.23** The stress response. 11.2.05 Renal Hormones In addition to its involvement in several other important bodily functions, the [kidney](javascript:void(0)) possesses endocrine function via the production of the peptide hormone **erythropoietin (EPO)**. Decreased oxygen levels in the blood delivered to the kidneys stimulate EPO production. Via the bloodstream, EPO reaches target cells in the red bone marrow, signaling an increase in red blood cell (ie, erythrocyte) production. More erythrocytes in circulation increases the oxygen-carrying capacity of the blood, thereby raising blood oxygen content back to homeostatic levels, as shown in Figure 11.24.  Chapter 11: Endocrine System 398 **Figure 11.24** Erythropoietin maintains blood oxygen homeostasis. In addition to EPO production, the kidney is involved in the activation of vitamin D to vitamin D3, also known as **calcitriol**. Calcitriol acts as a steroid hormone that promotes calcium absorption from ingested food in the small intestine. Calcitriol also acts on the kidney itself to promote [reabsorption](javascript:void(0)) of calcium in the nephron tubules. The overall effect of calcitriol activation is a net increase in serum calcium. 11.2.06 Pancreatic Hormones The **pancreas** is an organ with both [exocrine](javascript:void(0)) and endocrine functions. The exocrine pancreas is composed of glands known as pancreatic acini and secretes products (eg, pancreatic lipase, pancreatic amylase) as a solution known as pancreatic juice into the [duodenum](javascript:void(0)) of the digestive system. The role of the exocrine pancreas in facilitating digestion is discussed in more detail in Concept 15.2.03. Chapter 11: Endocrine System 399 The endocrine pancreas consists of functional units known as islets of Langerhans, which are made up of three types of cells, as shown in Figure 11.25: **Alpha cells** produce the peptide hormone **glucagon**. Alpha cells can also exhibit [paracrine](javascript:void(0)) function through the inhibition of beta cell function in certain settings. **Beta cells** produce the peptide hormone **insulin**, which inhibits neighboring alpha cell function; therefore, beta cells also exhibit paracrine function. **Delta cells** produce **somatostatin**, a peptide hormone that has a generalized inhibitory effect on digestive function and has been shown to suppress insulin and glucagon release. The [products](javascript:void(0)) of alpha and beta cells (ie, glucagon and insulin) are critical in maintaining [glucose](javascript:void(0)) [homeostasis](javascript:void(0)). Glucose is an essential molecule in the body, serving as a source of necessary metabolic intermediates (eg, oxaloacetate for the oxidation of macronutrient metabolites in the [Krebs cycle](javascript:void(0))) and as an [energy substrate](javascript:void(0)) for ATP generation. **Figure 11.25** The pancreas has exocrine and endocrine functions. In the [fasted state](javascript:void(0)), circulating blood glucose levels are maintained due to the breakdown of glycogen stores in the liver (ie, glycogenolysis) and through [gluconeogenesis](javascript:void(0)) (ie, the synthesis of glucose from other molecules). Conversely, in the fed state (ie, after consumption of a carbohydrate-containing meal), blood glucose levels initially increase because glucose is absorbed from the digestive system lumen into the bloodstream, from which glucose can be taken up by tissues and stored as glycogen (ie, glycogenesis).  Chapter 11: Endocrine System 400 When blood glucose levels are low (ie, hypoglycemia), glucagon acts on target cells (eg, in the liver) by binding a [G protein--coupled receptor](javascript:void(0)) on the plasma membrane and inducing the [adenylate](javascript:void(0)) [cyclase/cAMP](javascript:void(0)) second messenger cascade (see Concept 5.2.02). In response, gluconeogenesis and glycogenolysis are promoted in target cells, raising blood glucose levels. In addition, higher glucagon levels are often accompanied by higher availability of amino acids and glycerol for gluconeogenesis. Glucagon limits glucose use in insulin-sensitive tissues and decreases glucose uptake by peripheral tissues. Catecholamines from the adrenal medulla stimulate glucagon release and directly promote glycogenolysis (see Concept 11.2.04). Figure 11.26 summarizes the responses to hypoglycemia. **Figure 11.26** Cellular responses to low blood glucose. When blood glucose levels are high (ie, hyperglycemia), insulin binds insulin receptors in target tissues, causing translocation of glucose transporters to the plasma membrane of these cells. Therefore, binding of insulin allows markedly increased rates of glucose uptake, promoting glycogenesis in the liver and muscle. Insulin also stimulates fat synthesis (via glucose transport into adipocytes) and protein synthesis (via entry of plasma amino acids into tissues), increasing fat and protein stores for use during the fasted state. Insulin inhibits glucagon release, decreases liver gluconeogenesis, and decreases liver and muscle glycogenolysis. Figure 11.27 summarizes the responses to hyperglycemia. Chapter 11: Endocrine System 401 **Figure 11.27** Cellular responses to high blood glucose. **Diabetes mellitus** is a condition characterized by hyperglycemia and is divided into two subtypes. In type 1 (insulin-dependent) diabetes mellitus, insulin production is low or absent due to autoimmune destruction of pancreatic beta cells. Individuals with type 1 diabetes mellitus benefit from supplemental insulin. Type 2 (non-insulin-dependent) diabetes mellitus is characterized by insulin resistance and increased glucose production by the liver. Due to insulin resistance, supplemental insulin is not as helpful in individuals with type 2 diabetes mellitus. The final hormone produced by the pancreas, somatostatin, is also produced in the hypothalamus, where it inhibits growth hormone secretion by the anterior pituitary (see Concept 11.2.03). Somatostatin is secreted by pancreatic delta cells in response to high glucose and amino acid concentrations in the blood and has a general inhibitory effect on digestive processes