API RP 577 Welding Processes, Inspection, and Metallurgy PDF 2020
Document Details
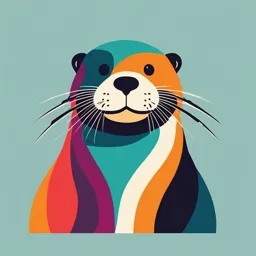
Uploaded by LionheartedMolybdenum
2020
Tags
Related
- API RP 577 Welding Processes, Inspection, and Metallurgy 2020 PDF
- API RP 577 Welding Processes, Inspection, and Metallurgy PDF 2020
- API RP 577 Welding Processes, Inspection, and Metallurgy PDF
- Welding Metallurgy of Steels Module 21 PDF
- AWS B5.16:2006 PDF Specification for the Qualification of Welding Engineers
- Complete Engineering Theory Book PDF
Summary
This document is API Recommended Practice 577, Third Edition, October 2020, covering welding processes, inspection, and metallurgy. It details recommended practices and standards for the industry.
Full Transcript
Welding Processes, Inspection, and Metallurgy API RECOMMENDED PRACTICE 577 THIRD EDITION, OCTOBER 2020 Special Notes API publications necessarily address problems of a general nature. With respect to particular circumstances, local, state, and federal laws and regulations should be reviewed. Nei...
Welding Processes, Inspection, and Metallurgy API RECOMMENDED PRACTICE 577 THIRD EDITION, OCTOBER 2020 Special Notes API publications necessarily address problems of a general nature. With respect to particular circumstances, local, state, and federal laws and regulations should be reviewed. Neither API nor any of API's employees, subcontractors, consultants, committees, or other assignees make any warranty or representation, either express or implied, with respect to the accuracy, completeness, or usefulness of the information contained herein, or assume any liability or responsibility for any use, or the results of such use, of any information or process disclosed in this publication. Neither API nor any of API’s employees, subcontractors, consultants, or other assignees represent that use of this publication would not infringe upon privately owned rights. API publications may be used by anyone desiring to do so. Every effort has been made by the Institute to assure the accuracy and reliability of the data contained in them; however, the Institute makes no representation, warranty, or guarantee in connection with this publication and hereby expressly disclaims any liability or responsibility for loss or damage resulting from its use or for the violation of any authorities having jurisdiction with which this publication may conflict. API publications are published to facilitate the broad availability of proven, sound engineering and operating practices. These publications are not intended to obviate the need for applying sound engineering judgment regarding when and where these publications should be utilized. The formulation and publication of API publications is not intended in any way to inhibit anyone from using any other practices. Any manufacturer marking equipment or materials in conformance with the marking requirements of an API standard is solely responsible for complying with all the applicable requirements of that standard. API does not represent, warrant, or guarantee that such products do in fact conform to the applicable API standard. All rights reserved. No part of this work may be reproduced, translated, stored in a retrieval system, or transmitted by any means, electronic, mechanical, photocopying, recording, or otherwise, without prior written permission from the publisher. Contact the Publisher, API Publishing Services, 200 Massachusetts Avenue, NW, Suite 1100, Washington, DC 20001. Copyright © 2020 American Petroleum Institute Foreword Nothing contained in any API publication is to be construed as granting any right, by implication or otherwise, for the manufacture, sale, or use of any method, apparatus, or product covered by letters patent. Neither should anything contained in the publication be construed as insuring anyone against liability for infringement of letters patent. The verbal forms used to express the provisions in this document are as follows. Shall: As used in a standard, “shall” denotes a minimum requirement in order to conform to the standard. Should: As used in a standard, “should” denotes a recommendation or that which is advised but not required in order to conform to the standard. May: As used in a standard, “may” denotes a course of action permissible within the limits of a standard. Can: As used in a standard, “can” denotes a statement of possibility or capability. This document was produced under API standardization procedures that ensure appropriate notification and participation in the developmental process and is designated as an API standard. Questions concerning the interpretation of the content of this publication or comments and questions concerning the procedures under which this publication was developed should be directed in writing to the Director of Standards, American Petroleum Institute, 200 Massachusetts Avenue, Suite 1100, Washington, DC 20001. Requests for permission to reproduce or translate all or any part of the material published herein should also be addressed to the director. Generally, API standards are reviewed and revised, reaffirmed, or withdrawn at least every five years. A one-time extension of up to two years may be added to this review cycle. Status of the publication can be ascertained from the API Standards Department, telephone (202) 682-8000. A catalog of API publications and materials is published annually by API, 200 Massachusetts Avenue, Suite 1100, Washington, DC 20001. Suggested revisions are invited and should be submitted to the Standards Department, API, 200 Massachusetts Avenue, Suite 1100, Washington, DC 20001, [email protected]. iii Contents Page 1 Scope............................................................................... 1 2 Normative References................................................................. 1 3 Terms, Definitions, and Acronyms....................................................... 3 3.1 Terms and Definitions................................................................. 3 3.2 Acronyms........................................................................... 12 4 Welding Processes................................................................... 12 4.1 General............................................................................. 12 4.2 Shielded Metal Arc Welding (SMAW).................................................... 12 4.3 Gas Tungsten Arc Welding (GTAW)..................................................... 15 4.4 Gas Metal Arc Welding (GMAW)........................................................ 18 4.5 Flux-Cored Arc Welding (FCAW)........................................................ 21 4.6 Submerged Arc Welding (SAW)......................................................... 24 4.7 Stud Arc Welding (SW)................................................................ 26 4.8 Plasma Arc Welding (PAW)............................................................ 26 4.9 Electrogas Welding (EGW)............................................................. 28 5 Welding Materials.................................................................... 30 5.1 General............................................................................. 30 5.2 P-Number Assignment to Base Metals................................................... 30 5.3 F-Number Assignment to Filler Metals................................................... 31 5.4 AWS Classification of Filler Metals...................................................... 31 5.5 A-Number........................................................................... 31 5.6 Filler Metal Selection................................................................. 31 5.7 Consumable Storage and Handling...................................................... 32 6 Welding Procedure................................................................... 32 6.1 General............................................................................. 32 6.2 Welding Procedure Specification (WPS).................................................. 33 6.3 Procedure Qualification Record (PQR)................................................... 45 6.4 Reviewing the WPS and PQR........................................................... 45 6.5 Tube-to-Tubesheet Welding Procedures................................................. 45 7 Welder Qualification.................................................................. 47 7.1 General............................................................................. 47 7.2 Welders and Welding Operators........................................................ 47 7.3 Examination Failure of a Production Weld................................................ 47 7.4 Retest for qualification................................................................ 47 7.5 Expiration, Revocation, and Renewal of Welder or Welding Operator Qualification.............. 47 7.6 Welder Performance Qualification...................................................... 47 7.7 Reviewing a WPQ.................................................................... 48 7.8 Limitations for Welder Qualifications.................................................... 49 8 Nondestructive Examination........................................................... 50 8.1 Discontinuities/imperfections.......................................................... 50 8.2 Materials Identification................................................................ 54 8.3 Visual Examination (VT)............................................................... 55 8.4 Magnetic Particle Examination (MT)..................................................... 62 8.5 Alternating Current Field Measurement.................................................. 66 8.6 Liquid Penetrant Examination (PT)...................................................... 67 v Contents Page 8.7 Eddy Current Examination (ET)......................................................... 69 8.8 Radiographic Examination (RT)......................................................... 69 8.9 Ultrasonic Examination (UT)........................................................... 83 8.10 Hardness Testing.................................................................... 95 8.11 Pressure and Leak Testing/Examination (LT)............................................. 96 9 Welding Inspection................................................................... 97 9.1 General............................................................................. 97 9.2 Tasks Prior to Welding................................................................ 97 9.3 Tasks During Welding Operations...................................................... 101 9.4 Tasks Upon Completion of Welding.................................................... 103 9.5 Nonconformances and Defects........................................................ 105 9.6 NDE Examiner Certification........................................................... 105 9.7 Weld Inspection Data Recording....................................................... 106 10 Metallurgy......................................................................... 109 10.1 General............................................................................ 109 10.2 Structure of Metals and Alloys........................................................ 109 10.3 Physical Properties.................................................................. 111 10.4 Mechanical Properties............................................................... 113 10.5 Preheating......................................................................... 116 10.6 Heat Treatment..................................................................... 116 10.7 Material Test Reports................................................................ 119 10.8 Weldability of Metals................................................................. 120 10.9 Weldability of High Alloys............................................................ 122 11 Refinery and Petrochemical Plant Welding Issues........................................ 124 11.1 General............................................................................ 124 11.2 Hot Tapping and In-Service Welding.................................................... 124 11.3 Lack of Fusion With GMAW-S Welding Process.......................................... 127 11.4 Caustic Service..................................................................... 128 11.5 Controlled Deposition Welding........................................................ 128 12 Safety Precautions.................................................................. 130 Annex A (normative) Technology and Symbols............................................... 131 Annex B (normative) Actions to Address Improperly Made Production Welds..................... 137 Annex C (informative) WPS/PQR Review.................................................... 139 Annex D (normative) Guide to Common Filler Metal Selection................................... 174 Annex E (informative) Example Report of RT Results.......................................... 178 Annex F (informative) Inspection Considerations............................................. 179 Annex G (informative) Welding Safety....................................................... 181 Bibliography............................................................................... 182 Figures 1 SMAW Welding Process............................................................... 13 2 SMAW Welding Electrode Deposition.................................................... 14 3 GTAW Welding Equipment............................................................. 16 vi Contents Page 4 GTAW Welding Process............................................................... 17 5 GMAW Equipment.................................................................... 18 6 GMAW Welding Process............................................................... 19 7 FCAW Equipment.................................................................... 22 8 FCAW Welding Process............................................................... 23 9 FCAW Welding Process, Self-shielded................................................... 23 10 SAW Welding Process................................................................ 25 11 Comparison of the Gas Tungsten Arc and Plasma Arc Welding Processes....................... 27 12 Electrogas Welding With a Solid Electrode.................................................. 29 13 Typical Discontinuities Present in a Single Bevel Groove Weld in a Butt Joint.................... 52 14 Direct Visual Examination Requirements.................................................... 55 15 Inspector’s Kit.......................................................................... 58 16 Bridge Cam Gauge...................................................................... 58 17 Adjustable Fillet Weld Gauge.............................................................. 59 18 Skew-T Fillet Weld Gauge................................................................. 59 19 Weld Fillet Gauge....................................................................... 60 20 Weld Fillet Gauge....................................................................... 60 21 Weld Size Gauge........................................................................ 61 22 Hi-Lo Gauge............................................................................ 61 23 Surface-Breaking Discontinuity............................................................ 62 24 Subsurface Discontinuity................................................................. 62 25 Weld Discontinuity...................................................................... 64 26 Flux Lines.............................................................................. 64 27 Detecting Discontinuities Transverse to Weld................................................ 65 28 Detecting Discontinuities Parallel to the Weld................................................ 65 29 Pie Gauge.............................................................................. 66 30 Fluorescent Penetrant Technique.......................................................... 68 31 IQI Common Hole Diameters.............................................................. 71 32 IQI................................................................................. 72 33 Single-Wall Techniques............................................................... 74 34 Double-Wall Techniques............................................................... 74 35 Incomplete or Lack of Penetration (LOP).................................................... 77 36 Interpass Slag Inclusions................................................................ 77 37 Cluster Porosity..................................................................... 78 38 Lack of Side Wall Fusion.............................................................. 78 39 Elongated Slag (Wagon Tracks)......................................................... 79 40 Burn-Through....................................................................... 79 41 Offset or Mismatch With Lack of Penetration (LOP)........................................... 80 42 Excessive Penetration (Icicles, Drop-Through)............................................... 80 43 Internal (Root) Undercut............................................................... 81 44 Transverse Crack.................................................................... 81 45 Tungsten Inclusions.................................................................. 82 46 Root Pass Aligned Porosity............................................................ 82 47 A-Scan............................................................................. 84 48 B-Scan............................................................................. 84 49 C-Scan............................................................................. 85 50 D-Scan............................................................................. 86 51 S-Scan............................................................................. 87 52 TOFD D-Scan Display................................................................. 88 Contents Page 53 TOFD B-Scan........................................................................ 89 54 TOFD Transducer Arrangement and Ultrasonic Energy Beam Propagation..................... 89 55 DAC Curve for a Specified Reference Reflector............................................ 92 56 DAC Curve for an Unknown Reflector.................................................... 92 57 Location of Hardness Measurements.................................................... 96 A.1 Joint Types and Applicable Welds...................................................... 132 A.2 Symbols for Various Weld Joints...................................................... 133 A.3 Supplementary Symbols for Welds..................................................... 133 A.4 Standard Weld Symbols.............................................................. 134 A.5 Groove Weld Nomenclature........................................................... 135 A.6 SMAW Welding Electrode Identification System.......................................... 135 A.7 GMAW/GTAW/PAW Welding Electrode Identification System................................ 135 A.8 FCAW Welding Electrode Identification System.......................................... 136 A.9 SAW Welding Electrode Identification System............................................ 136 A.10 EGW Welding Electrode Identification System........................................... 136 B.1 Suggested Actions for Welds Made by an Incorrect Welder................................. 137 B.2 Steps to Address Production Welds Made by an Improper Welding Procedure................ 138 Tables 1 P-Number Assignments.................................................................. 31 2 Common Types of Discontinuities......................................................... 51 3 Commonly Used NDE Methods............................................................ 52 4 Capability of the Applicable Examination Method for Weld-Type Joints.......................... 53 5 Capability of the Applicable Method vs. Discontinuity......................................... 53 6 Discontinuities Commonly Encountered with Welding Processes............................... 54 7 ASTM E94 IQIs.......................................................................... 71 8 Conditions That May Exist in a Material or Product....................................... 108 9 Results of Nondestructive Examination................................................. 108 10 Results of Application of Acceptance/Rejection Criteria................................... 108 11 Brinell Hardness Limits for Steels in Refining Services.....................................118 12 Weld Crack Tests.................................................................... 122 13 Hot Tapping/In-Service Welding Hazards Associated With Some Particular Substances......... 126 D.1 Common Welding Consumables for SMAW of Carbon and Low-Alloy Steel................... 174 D.2 Common Welding Consumables for SMAW of Carbon and Low-Alloy Steel................... 175 D.3 Copper-Nickel and Nickel-Based Alloys................................................. 176 D.4 Classification Changes in Low-Alloy Filler Metal Designations.............................. 177 Welding Processes, Inspection, and Metallurgy 1 Scope This recommended practice (RP) provides guidance to the API authorized inspector on welding inspection as encountered with fabrication and repair of refinery and chemical plant equipment and piping, pipelines, and other related industries. This RP includes descriptions of common welding processes, welding procedures, welder qualifications, metallurgical effects from welding, and inspection techniques to aid the inspector in fulfilling their role implementing API 510, API 570, API 653, and API 582. The level of learning and training obtained from this document is not a replacement for the training and experience required to be a certified welding inspector under one of the established welding certification programs, such as the American Welding Society (AWS) Certified Welding Inspector (CWI), or Canadian and European equivalent schemes such as CWB, CSWIP, PCN, or EFW. This RP does not require all welds to be inspected, nor does it require welds to be inspected to specific techniques and extent. Welds selected for inspection, and the appropriate inspection techniques, should be determined by the welding inspectors, engineers, or other responsible personnel using the applicable code or standard. The importance, difficulty, and problems that could be encountered during welding should be considered by all involved. A welding engineer should be consulted on any critical, specialized, or complex welding issues. 2 Normative References The following referenced documents are indispensable for the application of this document. For dated references, only the edition cited applies. For undated references, the latest edition of the referenced document (including any amendments) applies. API 510, Pressure Vessel Inspection Code: Maintenance, Inspection, Rating, Repair, and Alteration API 570, Piping Inspection Code: Inspection, Repair, Alteration, and Rerating of In-Service Piping Systems API Recommended Practice 571, Damage Mechanisms Affecting Fixed Equipment in the Refining Industry API Recommended Practice 574, Inspection Practices for Piping System Components API Recommended Practice 578, Material Verification Program for New and Existing Alloy Piping Systems API Recommended Practice 582, Recommended Practice and Supplementary Welding Guidelines for the Chemical, Oil, and Gas Industries API Recommended Practice 2201, Procedures for Welding or Hot Tapping on Equipment in Service ASME Boiler and Pressure Vessel Code 1 Section VIII, Rules for Construction of Pressure Vessels 1 ASME International, 3 Park Avenue, New York, New York 10016-5990, www.asme.org. 2 API RECOMMENDED PRACTICE 577 Section IX, Qualification Standard for Welding and Brazing Procedures, Welders, Brazers, and Welding and Brazing Operators Section XI, Rules for Inservice Inspection of Nuclear Power Plant Components ASME B31, Code for Pressure Piping B31.1, Power Piping B31.3, Process Piping B31.4, Liquid Transportation Systems for Hydrocarbons, Liquid Petroleum Gas, Anhydrous Ammonia and Alcohols B31.8, Gas Transmission and Distribution Piping Systems ASME PCC-2, Repair of Pressure Equipment and Piping ASNT Central Certification Program CP-189 2, Standard for Qualification and Certification of Nondestructive Testing Personnel ASNT Central Certification Program SNT-TC-1A, Personnel Qualification and Certification in Nondestructive Testing ASTM A833 3, Standard Test Method for Indentation Hardness of Metallic Materials by Comparison Hardness Testers ASTM A956, Standard Test Method for Leeb Hardness Testing of Steel Products ASTM A1038, Standard Practice for Portable Hardness Testing by the Ultrasonic Contact Impedance Method ASTM E94, Standard Guide for Radiographic Examination ASTM E1316, Standard Terminology for Nondestructive Examinations AWS A3.0M/A3.0:2010 4, Standard Welding Terms and Definitions AWS A5.XX, Series of Filler Metal Specifications ISO 9712 5, Non-destructive testing—Qualification and certification of NDT personnel NACE SP0472 6, Methods and Controls to Prevent In-Service Environmental Cracking of Carbon Steel Weldments in Corrosive Petroleum Refining Environments WRC Bulletin 342 7, Stainless Steel Weld Metal: Prediction of Ferrite Content 2 American Society for Nondestructive Testing, 1711 Arlingate Lane, P.O. Box 28518, Columbus, Ohio 43228, www.asnt.org. 3 ASTM International, 100 Barr Harbor Drive, West Conshohocken, Pennsylvania 19428, www.astm.org. 4 American Welding Society, 550 NW LeJeune Road, Miami, Florida 33126, www.aws.org. 5 International Organization for Standardization, 1, ch. de la Voie-Creuse, Case postale 56, CH-1211, Geneva 20, Switzerland, www.iso.org. 6 NACE International (formerly the National Association of Corrosion Engineers), 1440 South Creek Drive, Houston, Texas 77218-8340, www.nace.org. 7 The Welding Research Council, 3 Park Avenue, 27th Floor, New York, New York 10016-5902, www.forengineers.org. WELDING PROCESSES, INSPECTION, AND METALLURGY 3 3 Terms, Definitions, and Acronyms 3.1 Terms and Definitions In an effort to minimize confusion, definitions in this document have been harmonized with AWS standard welding terms and definitions. Any variances from those definitions are identified with footnotes. The reader should consult the applicable code of construction for definitions of terms as they apply to that specific code. For the purposes of this document, the following terms and definitions apply. alternating current field measurement ACFM Electromagnetic inspection technique that can be used to detect and size surface-breaking (or in some cases near-surface) defects in both magnetic and non-magnetic materials. actual throat Shortest distance between the weld root and the face of a fillet weld. air carbon arc cutting CAC-A Carbon arc cutting process variation that removes molten metal with a jet of air. arc blow Deflection of an arc from its normal path due to by magnetic forces. arc length Distance from the tip of the welding electrode to the adjacent surface of the weld pool. arc strike arc burn Discontinuity resulting from an arc, consisting of any localized remelted metal, heat-affected metal, or change in the surface profile of any metal object. arc welding AW Group of welding processes producing coalescence of workpieces by melting them with an arc, with or without the application of pressure and with or without filler metal. artifact radiographic film artifact Indications on radiographic film that may be caused by damage to the film before, during, or after processing. NOTE It is extremely important to identify these false indications as such and note them on the interpreter’s report. autogenous weld Fusion weld made without filler metal. 4 API RECOMMENDED PRACTICE 577 back-gouging Removal of weld metal and base metal from the weld root side of a welded joint to facilitate complete fusion and complete joint penetration upon subsequent welding from that side. backing Material or device placed against the back side of the joint adjacent to the joint root, or at both sides of a joint in electroslag or electrogas welding, to support and shield molten weld metal. The material may be partially fused or remain unfused during the welding process. base metal base material substrate parent metal (nonstandard) Metal or alloy being welded, brazed, soldered, or cut. base metal zone BMZ Portion of base metal adjacent to a weld, braze, or solder joint or thermal cut and unaffected by welding, brazing, soldering, or thermal cutting. NOTE See also heat-affected zone and weld metal zone. bevel angle Angle between the bevel of a joint member and a plane perpendicular to the surface of the member. burn-through Hole or depression in the root bead of a single-groove weld due to excess penetration. buttering Surfacing variation depositing surfacing metal on one or more surfaces to provide metallurgically compatible weld metal for the subsequent completion of the weld. cold crack Crack occurring in a metal at or near ambient temperatures. NOTE Cold cracks can occur in base metal, heat-affected, and weld metal zones. See also hot crack. complete fusion Fusion over the entire fusion faces and between all adjoining weld beads. NOTE See also incomplete fusion. constant-current power source CC Arc welding power source with a volt–ampere relationship yielding a small welding current change from a large arc voltage change. WELDING PROCESSES, INSPECTION, AND METALLURGY 5 constant-voltage power source CV Arc welding power source with a volt–ampere relationship yielding a large welding current change from a small arc voltage change. controlled deposition welding 8 CDW half-bead technique temper bead technique Welding technique used to obtain controlled grain refinement and tempering of the underlying heat-affected zone in the base metal. NOTE Various controlled-deposition techniques, such as temper bead (tempering of the layer below the current bead being deposited) and half bead (requiring removal of one-half of the first layer), are included. corrosion specialist Person, acceptable to the owner–user, who has knowledge and experience in corrosion damage mechanisms, metallurgy, materials selection, and corrosion monitoring techniques. crack Fracture-type discontinuity characterized by a sharp tip and high ratio of length and width to opening displacement. defect Discontinuity or discontinuities that, by nature or accumulated effect, render a part or product unable to meet minimum applicable acceptance standards or specifications (e.g. total crack length). NOTE The term designates rejectability. direct current electrode negative DCEN straight polarity Arrangement of direct current arc welding leads in which the electrode is the negative pole and the workpiece is the positive pole of the welding arc. direct current electrode positive DCEP reverse polarity Arrangement of direct current arc welding leads in which the electrode is the positive pole and the workpiece is the negative pole of the welding arc. discontinuity Interruption of the typical structure of a material, such as a lack of homogeneity in its mechanical, metallurgical, or physical characteristics. 8 Termnot defined in AWS A3.0M/A3.0:2010; definition is based on the definition in the International Association of Drilling Contractors (IADC) Lexicon. 6 API RECOMMENDED PRACTICE 577 NOTE A discontinuity is not necessarily a defect. See also defect and flaw. distortion Change in shape or dimensions, whether temporary or permanent, of a part as a result of heating or welding. eddy current examination technique ET Inspection method that applies primarily to nonferromagnetic materials. examiner Person who assists the inspector by performing specific NDE on components but does not evaluate the results of those examinations in accordance with the appropriate inspection code, unless specifically trained and authorized to do so by the owner or user. flaw Undesirable discontinuity. NOTE See also defect. filler metal Metal or alloy to be added in making a brazed, soldered, or welded joint. fillet weld size For equal leg fillet welds, the leg lengths of the largest isosceles right triangle that can be inscribed within the fillet weld cross section. For unequal leg fillet welds, the leg lengths of the largest right triangle that can be inscribed within the fillet weld cross section. groove angle Included angle between faces of a weld or groove between work pieces. heat-affected zone HAZ Portion of base metal whose mechanical properties or microstructure have been altered by the heat of welding, brazing, soldering, or thermal cutting. NOTE See also base metal zone and weld metal zone. heat input Energy applied to the workpiece during welding. hot crack Crack occurring in a metal during solidification or at elevated temperatures. Hot cracks can occur in both heat- affected zones and weld metal zones. NOTE See also cold crack. WELDING PROCESSES, INSPECTION, AND METALLURGY 7 image quality indicator9 IQI penetrameter (archaic) Device used to determine the sensitivity and ability of a radiograph to discern an image, or confirm the adequacy of the radiographic technique to produce radiographs with the required image quality level or radiographic sensitivity. Imperfection 10 Discontinuity or irregularity in a weld or deviation from the intended geometry that is detectable by methods outlined in this standard. inclusion Entrapped foreign solid material, such as slag, flux, tungsten, or oxide. incomplete fusion IF lack of fusion Weld discontinuity in which fusion did not occur between the weld metal and the fusion faces or adjoining weld beads. NOTE See also complete fusion. incomplete joint penetration Joint root condition in a groove weld in which weld metal does not extend through the joint thickness. NOTE See also joint penetration. inert gas Gas that does not react chemically with materials. indication Signal of discontinuity in the material under NDE. inspector Person who is qualified and certified to perform inspections under the appropriate inspection code or standard. interpass temperature, welding 11 Highest temperature in the weld joint immediately prior to welding, or in the case of multiple pass welds, the highest temperature in the section of the previously deposited weld metal, immediately before the next pass is started. 9 Term not defined in AWS A3.0M/A3.0:2010; definition is based on ASME V (2015), Article 1, I-121.1. 10 Term not defined in AWS A3.0M/A3.0:2010; definition is loosely based on ASME V (2017), Article 1, I-121. 11 Definition from ASME IX used as it is more descriptive than that in AWS. 8 API RECOMMENDED PRACTICE 577 joint penetration Distance that the weld metal extends from the weld face into a joint, exclusive of weld reinforcement. joint type Weld joint classification based on the relative orientation of the member being joined. NOTE The five basic joint types are butt, corner, edge, lap, and T-joints. lack of fusion LOF See incomplete fusion. lamellar tear Subsurface terrace and step-like crack in the base metal with a basic orientation parallel to the wrought surface caused by tensile stresses in the through-thickness direction of the base metals weakened by the presence of small, dispersed, planar-shaped, nonmetallic inclusions parallel to the metal surface. lamination Type of discontinuity with separation or weakness generally aligned parallel to the worked surface of a metal. longitudinal crack Crack with its major axis orientation approximately parallel to the weld axis. nondestructive examination NDE Act of determining the suitability of a material or component for its intended purpose using methods and techniques that do not affect its serviceability. nonrelevant indication12 NDE indication caused by a condition or type of discontinuity that is not rejectable. NOTE False indications are nonrelevant. Not all nonrelevant indications are recordable. overlap Protrusion of weld metal beyond the weld toe or weld root. NOTE The term also refers to the portion of the preceding weld nugget remelted by the succeeding weld in resistance seam welding. oxyacetylene cutting OFC-A Oxygen-fuel gas cutting process variation employing acetylene as the fuel gas. 12 Term not defined in AWS A3.0M/A3.0:2010; definition is from ASME V (2017), Article 1, I-121. WELDING PROCESSES, INSPECTION, AND METALLURGY 9 NOTE This is considered a thermal process and is an essential variable for some processes. It is not listed in ASME Section IX. peening Mechanical working of metals using impact blows. porosity Cavity-type discontinuities formed by gas entrapment during solidification or in a thermal spray deposit. positive material identification PMI Physical evaluation or test of a material to confirm that the material that has been or will be placed into service is consistent with the selected or specified alloy material designated by the owner–user. preheat Metal temperature value achieved in a base metal or substrate prior to initiating thermal operations. NOTE Also equal to the minimum interpass temperature. procedure qualification record PQR Record of welding variables used to produce an acceptable test weldment, and the results of tests conducted on the weldment to qualify a welding procedure specification. recordable indication Recording on a datasheet of an indication or condition that does not necessarily exceed the rejection criteria in terms of code or contract, but is documented. reportable indication Recording on a datasheet of an indication that exceeds the reject flaw size criteria and requires not only documentation, but also notification to the appropriate authority for correction. NOTE All reportable indications are recordable indications but not vice versa. relevant indication13 NDE indication caused by a condition or type of discontinuity that requires evaluation. NOTE All relevant indications are recordable. reverse polarity See direct current electrode positive. 13 Term not defined in AWS A3.0M/A3.0:2010; definition is based on ASME V (2017), Article 1, I-121. 10 API RECOMMENDED PRACTICE 577 root face Portion of the groove face within the joint root. root opening root spacing Separation at the joint root between the workpieces. shielding gas backing gas Gases used to produce a protective atmosphere. slag Nonmetallic product resulting from the mutual dissolution of flux and nonmetallic impurities in some welding and brazing processes. slag inclusion Discontinuity consisting of slag entrapped in weld metal or at the weld interface. spatter Metal particles expelled during fusion welding that do not form part of the weld. NOTE This is a deleterious surface condition that can interfere with subsequent operations such as inspection or coating. tack weld Weld made to hold the parts of a weldment in proper alignment until the final welds are made. temper bead welding See controlled deposition welding. throat, theoretical Distance from the beginning of the joint root perpendicular to the hypotenuse of the largest right triangle that can be inscribed within the cross-section of a fillet weld. NOTE This dimension is based on the assumption that the root opening is equal to zero. transverse crack Crack with its major axis oriented approximately perpendicular to the weld axis. travel angle drag angle push angle work angle Angle that is less than 90° between the electrode axis and a line perpendicular to the weld axis, in a plane determined by the electrode axis and the weld axis. NOTE This angle can also be used to partially define the position of guns, torches, rods, and beams. WELDING PROCESSES, INSPECTION, AND METALLURGY 11 tungsten inclusion Discontinuity consisting of tungsten entrapped in weld metal. undercut Groove melted into the base metal adjacent to the weld toe or weld root and left unfilled by weld metal. underfill Groove weld condition in which the weld face or root surface is below the adjacent surface of the base metal. welder Person who performs manual or semiautomatic welding. welder certification Written verification that a welder has produced welds meeting a prescribed standard of welder performance. welder performance qualification WPQ Demonstration of a welder’s or welding operator’s ability to produce welds meeting prescribed standards. welding Joining process that produces coalescence of materials by heating them to the welding temperature, with or without the application of pressure or by the application of pressure alone, and with or without the use of filler metal. welding engineer Person who holds an engineering degree and is knowledgeable and experienced in the engineering disciplines associated with welding. welding operator Person who operates adaptive control, automatic, mechanized, or robotic welding equipment. welding procedure specification WPS Document that provides the required welding variables for a specific application to ensure repeatability by properly trained welders and welding operators. weldment Assembly joined by welding. weld interface fusion line (nonstandard) Boundary between weld metal and base metal in a fusion weld, between base metals in a solid-state weld without filler metal, or between filler metal and base metal in a solid-state weld with filler metal. 12 API RECOMMENDED PRACTICE 577 weld joint Junction of members or edges of members that are to be joined or have been joined by welding. weld metal zone WMZ Portion of the weld area consisting of weld metal. NOTE See also base metal zone and heat-affected zone. weld reinforcement convexity face reinforcement root reinforcement Weld metal in excess of the quantity required to fill a weld groove. weld toe Junction of the weld face and the base metal. wet fluorescent magnetic-particle examination technique WFMT Inspection method suitable only for ferromagnetic materials. 3.2 Acronyms LT leak testing/examination method MT magnetic particle examination method NDE nondestructive examination PT dye penetrant examination method RT radiographic examination method VT visual examination method 4 Welding Processes 4.1 General The inspector should understand the basic arc welding processes most frequently used in the fabrication and repair of refinery and chemical process equipment. These processes include shielded metal arc welding (SMAW), gas tungsten arc welding (GTAW), gas metal arc welding (GMAW), flux-cored arc welding (FCAW), submerged arc welding (SAW), stud welding (SW), plasma arc welding (PAW), and electrogas welding (EGW). Descriptions of less frequently used welding process are available in the bibliography. Each process has advantages and limitations depending upon the application and can be prone to specific types of discontinuities. 4.2 Shielded Metal Arc Welding (SMAW) General SMAW is the most widely used of the various arc welding processes. SMAW creates an arc between a covered electrode and the weld pool. It employs the heat of the arc, originating from the tip of a consumable covered electrode, to melt the base metal. Shielding is provided by the decomposition of the electrode coating, without WELDING PROCESSES, INSPECTION, AND METALLURGY 13 the application of pressure, and with filler metal from the electrode. Either alternating current (AC) or direct current (DC) may be employed, depending on the required mechanical properties, welding power supply, and specific electrode selected. A constant-current (CC) power supply is preferred. SMAW is a manual welding process. See Figure 1 and Figure 2 for schematics of the SMAW welding process and electrode deposition, respectively. Figure 1—SMAW Welding Process 14 API RECOMMENDED PRACTICE 577 Figure 2—SMAW Welding Electrode Deposition Electrode Covering Depending on the type of electrode being used, the covering performs one or more of the following functions: provides a gas to shield the arc and prevent excessive atmospheric contamination of the molten filler metal; provides scavengers, deoxidizers, and fluxing agents to cleanse the weld and prevent excessive grain growth in the weld metal; establishes the electrical characteristics of the electrode, stabilizes the welding arc, and influences operability in various welding positions; provides a slag blanket to protect the molten weld metal from the atmosphere and enhances the mechanical properties, bead shape, and surface cleanliness of the weld metal; provides a means of adding alloying elements to produce appropriate weld metal chemistry and mechanical properties and to increase deposition efficiency. Advantages of SMAW Following are some commonly accepted advantages of the SMAW process. a) The equipment is relatively simple, inexpensive, and portable. b) The process can be used in areas of limited access. WELDING PROCESSES, INSPECTION, AND METALLURGY 15 c) The process is less sensitive to wind and draft than other welding processes. d) The process is suitable for most of the commonly used metals and alloys. e) The level of training and experience required is lower than for other welding processes. Limitations of SMAW Limitations associated with SMAW include the following. a) Deposition rates are lower than for other processes such as GMAW. b) Slag must be removed from every deposited weld pass, at stops and starts, and before depositing a weld bead adjacent to or onto a previously deposited weld bead. 4.3 Gas Tungsten Arc Welding (GTAW) General GTAW is an arc welding process that generates an arc between a nonconsumable tungsten electrode and the weld pool. The process is commonly, and somewhat incorrectly, referred to as tungsten inert gas (TIG) or heliarc welding, and is used with a shielding gas and without the application of pressure. GTAW can be used with or without the addition of filler metal. The CC-type power supply can be either DC or AC, and depends largely on the metal to be welded. Direct current welding is typically performed with the electrode negative (DCEN) polarity. DCEN welding offers the advantages of deeper penetration and faster welding speeds. Alternating current provides a cathodic cleaning (sputtering) that removes refractory oxides from the surfaces of the weld joint, which is necessary for the welding of nonferrous materials such as aluminum and magnesium. The cleaning action occurs during the portion of the AC wave, when the electrode is positive with respect to the work piece. See Figure 3 and Figure 4 for schematics of the GTAW equipment and welding process. 16 API RECOMMENDED PRACTICE 577 Figure 3—GTAW Welding Equipment WELDING PROCESSES, INSPECTION, AND METALLURGY 17 Figure 4—GTAW Welding Process Advantages of GTAW Some commonly accepted advantages of the GTAW process include the following: a) produces high purity welds, generally free from defects; b) requires little postweld cleaning; c) allows for excellent control of root pass weld penetration; d) can be used with or without filler metal, dependent on the application; e) produces a relatively defect- and contaminant-free root pass for those process services that may be more aggressive toward contaminated root passes. Limitations of GTAW Limitations associated with GTAW process are: a) lower deposition rates than the rates possible with consumable electrode arc welding processes, b) low tolerance for contaminants on filler or base metals, c) difficulty in shielding the weld zone properly in drafty environments, and 18 API RECOMMENDED PRACTICE 577 d) greater operator skill is required than that for other methods such as SMAW. 4.4 Gas Metal Arc Welding (GMAW) General GMAW is an arc welding process that produces an arc between a continuously fed filler metal electrode and the weld pool. The process is used with shielding from an externally supplied gas without the application of pressure. GMAW may be operated in semiautomatic, machine, or automated modes. It employs a constant- voltage (CV) power supply, and uses either the short-circuiting, globular, spray, or pulsed transfer modes to transfer filler metal from the electrode to the work. The transfer mode is determined by several factors. The most influential are: a) magnitude and type of welding current; b) electrode diameter; c) electrode composition; d) electrode extension or contact tube-to-work distance (often referred to as “stick out”); e) shielding gas. See Figure 5 and Figure 6 for schematics of the GMAW equipment and welding process. Figure 5—GMAW Equipment WELDING PROCESSES, INSPECTION, AND METALLURGY 19 Figure 6—GMAW Welding Process Short Circuiting Transfer Mode (GMAW-S) 4.4.2.1 General GMAW-S encompasses the lowest range of both welding current and electrode diameters associated with the GMAW process. This process produces a fast freezing weld pool that is generally suited for joining thin sections, out-of-position welding, or root passes. Due to the fast-freezing nature of this process, there is high potential for lack of sidewall and interpass fusion when welding thick-wall equipment or a nozzle attachment. The shielding gas for this transfer mode is typically 75 % argon with 25 % CO2. 4.4.2.2 GMAW-MSC Transfer Mode (Modified Short Circuit) The modified short-circuit GMAW mode, designated the GMAW-MSC process, has several proprietary derivatives of the short-circuiting transfer mode that use a modified waveform to reduce some problems associated with short-circuiting—mainly, spatter and a turbulent weld pool. These systems typically sense the progression of the short circuit as it occurs and modulate the current to limit the amount of force behind spatter and turbulence-producing events. GMAW-MSC power sources are software-driven to maintain optimum arc characteristics by closely monitoring and controlling the electrode current during all phases of the short circuit. There are a limited number of companies that manufacture welding power supplies that employ this technology. The GMAW-MSC transfer mode minimizes the disadvantages of GMAW-S while maintaining comparable weld metal deposition rates and achieving X-ray quality welds. The welding process has the capability to complete open root welds more rapidly than GTAW, with low heat input and no resulting lack of fusion (LOF). The lower heat input results in smaller heat-affected zones (HAZ) as well as reduced distortion and lesser chance of burn-through. The process appears to be more tolerant of inexperienced welders because GMAW-MSC is tolerant of gaps and capable of automatically maintaining the optimum wire feed speed and contact tip-to-work distance, while allowing the use of larger diameter GMAW consumables. 20 API RECOMMENDED PRACTICE 577 Globular Transfer Mode The advantages of this transfer method are its relative low cost when carbon dioxide is used as a shielding gas, along with a high deposition rate. The maximum deposition rate for the globular arc transfer mode is about 6350 mm/min (250 in./min). The globular arc transfer mode is often considered the least desirable of the GMAW transfer modes due to its tendency to produce high heat, a poor weld surface, and weld spatter, as well as cold lap. This transfer mode uses relatively low current (less than 250 A). During welding, a ball of molten metal from the electrode tends to build up on the end of the electrode, often in irregular shapes, with a diameter up to twice that of the electrode. When the droplet detaches (i.e. by gravity or short circuiting) and is forced onto the work piece, it produces an uneven surface as well as excessive weld spatter. This transfer mode produces a high amount of heat and forces the welder to use a larger electrode wire to maintain a stable arc, which increases the size of the weld pool and causes greater residual stresses and distortion in the weld area. This welding process uses 100 % carbon dioxide as the shielding gas, and is limited to the flat and horizontal positions. Spray Transfer Mode The spray transfer mode results in a highly directed stream of discrete drops that are accelerated by arc forces. Since these drops are smaller than the arc length, short circuits do not occur and the amount of spatter generated is negligible. The inert gas shield allows the spray arc transfer mode to weld most metals. However, using this process on materials thinner than 0.250 in. (6 mm) may be difficult because of the high currents needed to produce the spray arc. The spray transfer mode allows for high weld metal deposition rates. At high deposition rates, the welding process may produce a weld metal pool that is too weak to be supported by surface tension alone (primarily dependent upon electrode diameter), which limits the use of the welding process in the vertical and overhead positions. Specialized power supplies have been developed to address the work thickness and welding position limitations. The maximum deposition rate for the spray arc transfer mode is about 150 in./min (3810 mm/min). This transfer mode is typically 98 % argon, 2 % oxygen. Depending upon the wire diameter and amperage, the shielding gas may have composition 80 % argon, 20 % oxygen. Pulsed Transfer Mode Pulsed arc GMAW was developed to overcome the thickness and welding position limitations of the Globular, Spray, and Short Circuit modes. This transfer mode provides: a) a low background/constant current to sustain the arc without providing enough energy to produce drops at the tip of the wire; and b) a superimposed/pulsing current with an amplitude greater than the transition current necessary for spray transfer. During the pulsing portion of the current cycle, one or more drops are formed and transferred to the workpiece. The frequency and amplitude of the pulses control the rate at which the wire melts. Pulsing provides the desirable features of spray arc transfer for joining sheet metals and welding in all positions. The maximum deposition rate for the pulsed arc transfer mode is about 5080 mm/min (200 in./min). The pulsed arc GMAW method requires a power source capable of providing current pulses with a frequency between 30 pulses/s and 400 pulses/s, and requires that the shielding gas be primarily argon with a low carbon dioxide concentration. The same shielding gas used for the spray transfer mode is typically used for the pulsed transfer mode as well. Advantages of GMAW Some commonly accepted advantages of the GMAW process include the following. a) It is the only consumable electrode process that can be used to weld most commercial ferrous and nonferrous metals and alloys. b) Its deposition rates are significantly higher than those obtained with SMAW and GTAW. WELDING PROCESSES, INSPECTION, AND METALLURGY 21 c) Minimal postweld cleaning is required due to the absence of slag. Limitations of GMAW Limitations associated with GMAW include the following. a) The welding equipment is more complex, more costly, and less portable than that for SMAW. b) The welding arc must be protected from air drafts that can disperse the shielding gas. c) When using the GMAW-S process, the weld is more susceptible to lack of adequate fusion (see Section 11.3). As a result, ultrasonic examination (UT) is generally employed as the inspection method because the probability of detecting LOF is rather low using radiographic examination (RT) (see Sections 8.8 and 8.9). 4.5 Flux-Cored Arc Welding (FCAW) General FCAW is an arc welding process that produces an arc between a continuously fed tubular electrode and the weld pool. The process is used with shielding gas evolved from a flux contained within the tubular electrode itself, with or without additional shielding from an externally supplied gas, and without the application of pressure. Normally a semiautomatic process, the use of FCAW depends on the type of electrodes available, the mechanical property requirements of the welded joints, and the joint designs and fit-up. The recommended power source is the DC CV type, similar to power sources used for GMAW. Figure 7 shows a schematic of FCAW equipment, and Figure 8 shows the welding process with additional gas shielding. Figure 9 shows a schematic of the self-shielded FCAW process where no additional gas is used. 22 API RECOMMENDED PRACTICE 577 Figure 7—FCAW Equipment WELDING PROCESSES, INSPECTION, AND METALLURGY 23 Figure 8—FCAW Welding Process Figure 9—FCAW Welding Process, Self-shielded 24 API RECOMMENDED PRACTICE 577 Advantages of FCAW Some commonly accepted advantages of the FCAW process include: a) metallurgical benefits that can be derived from alloying elements contained within the flux; b) slag that supports and shapes the weld bead, allowing for a slower cooling rate; c) higher deposition and productivity rates compared to other processes such as SMAW and GTAW; d) shielding produced at the surface of the weld that makes it more tolerant of stronger air currents than GMAW. Limitations of FCAW The following limitations are associated with the FCAW process. a) The equipment is more complex, more costly, and less portable than that for SMAW. b) Self-shielding FCAW generates large volumes of welding fumes and requires suitable exhaust equipment. c) Slag must be removed between weld passes and removed from surfaces planned for inspection. If a weld is being placed in corrosive service, failure to remove slag from the weld cap or root can create sites for corrosion to initiate. d) Backing material is required for root pass welding. e) Self-shielded FCAW is typically not recommended for pressure-containing welds. 4.6 Submerged Arc Welding (SAW) General SAW is an arc welding process that uses an arc or arcs between a flux-covered bare metal electrode(s) and the weld pool. The arc and molten metal are shielded by a blanket of granular flux, supplied through the welding nozzle from a hopper. The process is used without pressure, and with filler metal from the electrode itself and sometimes from a supplemental source (welding electrode, flux, or metal granules). SAW can be applied in three different modes: semiautomatic, automatic, and machine. It can utilize either a CV or CC power supply. SAW is used extensively in shop pressure vessel fabrication and pipe manufacturing. Figure 10 shows a schematic of the SAW process. WELDING PROCESSES, INSPECTION, AND METALLURGY 25 Figure 10—SAW Welding Process Advantages of SAW Some commonly accepted advantages of the SAW process are the following. a) It provides very high metal deposition rates. b) It produces repeatable high-quality welds for large weldments and repetitive short welds. Limitations of SAW The following limitations are associated with SAW. a) A power supply capable of providing high amperage at 100 % duty cycle is recommended. b) The weld is not visible during the welding process. c) Equipment required is more costly and extensive and less portable; d) The process is generally limited to shop applications and flat position. 26 API RECOMMENDED PRACTICE 577 4.7 Stud Arc Welding (SW) General SW is an arc welding process that generates an arc between a metal stud or similar part and the work piece. A stud gun holds the tip of the stud against the work. Once the surfaces of the parts are properly heated (i.e. when the end of the stud is molten and the work has an equal area of molten pool), they are brought into contact by the application of pressure. Shielding gas or flux may or may not be employed. The process may be fully automatic or semiautomatic. Direct current is typically used for SW with the stud gun connected to the negative terminal (DCEN). The power source is a CC type. SW is a specialized process predominantly limited to welding insulation and refractory support pins to tanks, pressure vessels and heater casings. Advantages of SW Some commonly accepted advantages of the SW process include: a) high productivity rates compared to manually welding studs to base metal; b) it is considered to be an all-position process. Limitations of SW Limitations of SW are the following: a) the process is primarily suitable for only carbon steel and low-alloy steels; b) the process is specialized to a few applications. 4.8 Plasma Arc Welding (PAW) General PAW is a variation of the GTAW process except that the tungsten electrode is positioned within the body of the torch. This process is rarely used in the fabrication and repair of pressure equipment. There are two types of plasma arc welding, specifically the transferred arc process and nontransferred arc process. Plasma Transferred Arc (PTA) In the PTA process, similar to GTAW, the workpiece is part of the electrical circuit, and the arc is struck between the tungsten electrode and the workpiece. By constricting the arc, the plasma or ionized gas is forced through a fine-bore copper nozzle which constricts the arc, and exits the orifice at high velocities (approaching the speed of sound). The process produces a collimated arc which focuses the arc on a relatively small area of the workpiece with an arc temperature in excess of 20,000 °F (11,100 °C). Nontransferred Arc or Plasma Spray (PS) In the PS process, the arc is struck between the tungsten electrode and the constricting nozzle rather than with the workpiece. The plasma spray process is solely used for the deposition of surface coatings. It is not used for making strength welds. A comparison of the GTAW and PAW circuits and welding processes is shown in Figure 11. WELDING PROCESSES, INSPECTION, AND METALLURGY 27 Figure 11—Comparison of the Gas Tungsten Arc and Plasma Arc Welding Processes Advantages of PAW Some commonly accepted advantages of the PAW process include: a) high tolerance for misalignment in the arc; b) high welding rate; c) high penetrating capability (keyhole effect); d) less distortion resulting from lower total heat input due to focused arc; e) the weld itself and the HAZ are narrower than in traditional GTAW due to the constricted arc. Limitations of PAW The limitations of PAW include: a) expensive equipment; b) larger torch than GTAW making access more difficult in narrow weld joints; c) focused arc requiring better control by the welder. 28 API RECOMMENDED PRACTICE 577 4.9 Electrogas Welding (EGW) General EGW is similar to the GMAW process in that a solid electrode can be fed into the joint. Alternately, it is similar to the FCAW process in that a flux cored tubular electrode can be used. The weld is deposited in the flat position with the molten metal continually being deposited at the bottom of the moving cavity. In EGW, fixed or moving metal (usually copper) or ceramic shoes (or dams), water cooled if needed, are set up before starting, to bridge the gap between abutting plates and contain the molten metal until solidification is completed. The welding process utilizes either a solid wire or flux-cored electrode. The weld area is protected from atmospheric contamination by an externally supplied shielding gas, or by the gas produced by the disintegration of the flux- cored electrode wire. EGW is used to make square-groove welds for butt and T-joints in the construction of storage tanks, ship hulls, and pressure vessels with plate thicknesses from 3/8 in. to 4 in. (9 mm to 100 mm). The workpiece should be at least 0.4 in. (10 mm) thick, while the maximum thickness for one electrode is approximately 0.8 in. (20 mm). Additional electrodes make it possible to weld thicker workpieces. The height of the weld is limited only by the mechanism used to lift the welding head. In general, the height ranges from 4 in. to 50 ft (100 mm to 15 m). Low and medium carbon steels, low-alloy high-strength steels, and some stainless steels can be welded using the electrogas process. Quenched and tempered steels may also be welded by the process, provided adequate heat is applied. Figure 12 shows an EGW setup using a solid electrode. WELDING PROCESSES, INSPECTION, AND METALLURGY 29 Figure 12—Electrogas Welding With a Solid Electrode 30 API RECOMMENDED PRACTICE 577 Advantages of EGW Some commonly accepted advantages of the EGW process include the following: a) welding usually done in one pass; b) very high deposition rates; c) minimum distortion; d) ability to add beneficial alloying elements to the weldment. Limitations of EGW Some limitations of EGW include: a) low toughness; b) massive, expensive welding equipment and guidance systems required; c) lengthy set-up times needed; d) can only be used with vertically positioned joints; e) can require external source of shielding gas. 5 Welding Materials 5.1 General Welding materials refers to the many materials involved in welding, including the base metal, filler metal, fluxes, and gases, if any, as each of these has an impact on the welding procedure specification (WPS) and the weldment mechanical properties. An understanding of the conventions used by the ASME Section IX is necessary to adequately review a qualified welding procedure. 5.2 P-Number Assignment to Base Metals Base metals are assigned P-numbers in ASME Section IX to reduce the number of welding procedure qualifications required. For ferrous base metals having specified impact test requirements, group numbers within P-numbers are assigned, which may become essential variables under certain circumstances. These assignments are based on comparable base metal characteristics such as composition, weldability, and mechanical properties. Table 1 lists the assignments of base metals to P-numbers. A complete listing of P-number, S-number, and group number assignments are provided in QW/QB-422 of ASME Section IX. This list is an ascending sort based on specification numbers. Specification numbers grouped by P-number and group number are also listed in ASME Section IX nonmandatory Annex D. Within each list of the same P-number and group number, the specifications are listed in an ascending sort. WELDING PROCESSES, INSPECTION, AND METALLURGY 31 Table 1—P-Number Assignments Base Metal Welding Brazing P-No.1 through P-No. 11, including Steel and alloys P-No. 101 through P-No. 103 P-No. 5A, 5B, 5C, and 15E Aluminum and aluminum-base alloys P-No. 21 through P-No. 25 P-No. 104 and P-No. 105 Copper and copper-base alloys P-No. 31 through P-No. 35 P-No. 107 and P-No. 108 Nickel and nickel-base alloys P-No. 41 through P-No. 47 P-No. 110 through P-No. 112 Titanium and titanium-base alloys P-No. 51 through P-No. 53 P-No. 115 Zirconium and zirconium-base alloys P-No. 61 through P-No. 62 P-No. 117 Reprinted Courtesy of ASME. 5.3 F-Number Assignment to Filler Metals Electrodes and welding rods are assigned F-numbers to reduce the number of welding procedure and performance qualifications. The F-number groupings are based primarily upon their usability characteristics, which fundamentally determines the ability of welders to make satisfactory welds for a given process and filler metal. Welders who qualify with one filler metal are qualified to weld with all filler metals having the same F-number, and in the case of carbon steel SMAW electrodes, may additionally qualify to weld with electrodes having lower F-numbers. For example, a welder who qualified with an E7018 (an F-4 electrode) is qualified to weld with all F-4 electrodes, plus all F-1, F-2, and F-3 electrodes (with backing limitations). However, other F-numbers, such as F-6, qualify for that F-number alone. The grouping does not imply that base metals or filler metals within a group may be indiscriminately substituted for a metal that was used in the qualification test. Consideration should be given to the compatibility of the base and filler metals from the standpoint of metallurgical properties, postweld heat treatment, design and service requirements, and mechanical properties. A complete list of F-numbers for electrodes and welding rods is addressed in ASME Section IX, Table QW- 432. 5.4 AWS Classification of Filler Metals An AWS classification number identifies welding electrodes and rods. The AWS classification numbers are specified in ASME Section IIC under their appropriate SFA specification number. ASME Section IX, Table QW- 432, lists the AWS classification numbers and SFA specification numbers included under each of the F- numbers. Note that the Xs in the AWS classification numbers represent numerals, i.e. the AWS classifications E6010, E7010, E8010, E9010, and E10010 are incorporated under F-number 3 (EXX10). Annex A contains additional details on the conventions used in identification of filler metals for welding processes. 5.5 A-Number To minimize the number of welding procedure qualifications, steel and steel-alloy filler metals are also grouped according to their A-number. The A-number grouping in ASME Section IX, Table QW-442, is based on the chemical composition of the deposited weld metal. This grouping does not imply that filler metals may be indiscriminately substituted without consideration for the compatibility with the base metal and the service requirements. 5.6 Filler Metal Selection Inspectors should verify the filler metal selection is appropriate for the base metal being welded. Some considerations in selection include: 32 API RECOMMENDED PRACTICE 577 a) chemical composition of filler metal; b) tensile strength of filler metal and base metal; c) dilution of alloying elements from base metal; d) hardenability of filler metal; e) susceptibility to hot cracking; f) corrosion resistance of filler metal; g) toughness. Annex D provides a guide of common filler metals for base metals most often used in petrochemical plants. In addition, Table D.4 compares the current AWS filler metal classifications to the previous ones for low-alloy steels. AWS has modified the classifications for several common low-alloy filler metals. 5.7 Consumable Storage and Handling Welding consumable storage and handling guidelines should be in accordance with the consumable manufacturer’s instructions and guidelines and as given in the AWS A5.XX series of filler metal specifications. Covered electrodes exposed to moisture can become unstable due to moisture pickup by the coating. Particularly susceptible to moisture pickup are coatings on low-hydrogen electrodes and stainless-steel electrodes. Moisture can be a source of hydrogen, which can cause weldment cracking. To reduce exposure to moisture, certain welding consumables should be stored in heated holding ovens after they have been removed from the manufacturer’s packaging. Low-hydrogen SMAW electrodes are characterized by a final identification digit of 5, 6, or 8 (e.g. E7016, E8018) and are supplied in non-hermetically sealed containers. Some manufacturers recommend that these electrodes be warmed according to specific temperature requirements prior to use. The electrodes should be stored separately from other types of electrodes with higher hydrogen content, such as cellulose-based electrodes (E6010, E8010), as these can be a primary source of hydrogen pickup. Some welding consumables that are slightly damp can be reconditioned by baking in separate special ovens; however, this is not a universally accepted practice. Electrode ovens should be heated by electrical means and have automatic heat controls and visible temperature indicators. These ovens should only be used for electrode storage, as using them for food storage or cooking could cause the electrode coatings to absorb moisture. Any electrodes or fluxes that have become wet should be discarded. 6 Welding Procedure 6.1 General Qualified welding procedures are required for welding fabrication and repair of pressure vessels, piping, tanks, and other items. These procedures detail the steps necessary to make a specific weld and generally consist of a written description, details of the weld joint and welding process variables, and test data to demonstrate that the procedure produces weldments meeting design requirements. While various codes and standards exist for the development of welding procedures, this section reflects criteria described in ASME BPVC Section IX. Welding procedures qualified to ASME Section IX are required by API inspection codes for both fabrication and repair welding. However, construction codes and proprietary company specifications may have additional requirements or allow specific additions or exceptions, so they should be reviewed for each weld application. Welding procedures required by ASME Section IX include a written WPS and an attendant document called a procedure qualification record (PQR). The purpose of the WPS is to provide specific direction to the person applying the material during the welding process. As well as defining parameters for the welder or welding WELDING PROCESSES, INSPECTION, AND METALLURGY 33 operator, the WPS also provides information to the welding inspector to measure a weld against the relevant WPS. The PQR is a record of the welding data and variables used to weld a test coupon and the test results used to qualify the WPS. The purpose of PQR is to demonstrate that the joining process proposed for construction is capable of producing joints having the required mechanical properties for the intended application. It is important to differentiate the PQR from the welder performance qualification (WPQ), detailed in Section 7. The purpose of the WPQ is to provide a record of the welding variables and results of tests conducted on a weldment to establish that the welder is capable of making an acceptable quality weld using an appropriate WPS. 6.2 Welding Procedure Specification (WPS) General ASME Section IX requires each organization to develop welding procedures to be used in the fabrication or repair of components. While this requirement may appear repetitious, qualified WPSs are an important aspect of fabrication quality control. They help each organization recognize the significance of changes in welding variables that may be required on the job and the effects of the changes on weldment properties. The WPS is only one step for welding fabrication quality assurance. Some codes and standards allow welding procedure qualification by others, provided the qualification is acceptable to the inspector and meets other conditions imposed by the referencing code or standard. The complete WPS for a welding process addresses all essential, supplementary essential, and nonessential variables when impact testing is required, or when specified by the end user (see ASME Section IX QG-101). Essential variables affect the mechanical properties of the weld. If they are changed beyond what the reference code paragraph allows for the process, the WPS shall be requalified. Nonessential variables do not affect the mechanical properties of the weld. They may be changed on the WPS without requalifying the welding procedure. When supplementary essential variables apply, or when specified by the end user, they are treated as essential variables. 34 API RECOMMENDED PRACTICE 577 Types of Variables (Refer to C.4.1) 6.2.2.1 Essential, Supplementary Essential, and Nonessential Variables for Shielded Metal Arc Welding (SMAW) Supplementary Essential Nonessential Article IV Paragraph Process Variable Essential WPS PQR WPS PQR WPS PQR.1 Change in groove design X.5 The deletion of backing X Joints QW-402.10 Change in root spacing X.11 The addition or deletion of X retainers.5 Change in the group number X X.6 Base metal thickness (T) limits Base Metals X X toughness QW-403.8 Base metal thickness (T) qualified X X.11 The change in P-number qualified X X.3 A change in the size of filler metal X.4 A change in the F-number X X.5 A change in the A-number X X.12 A change in the filler metal X X classification.14 The addition or deletion of filler X X Filler Metals metal QW-404.22 The addition or deletion of a X consumable insert.23 A change in the filler metal X X product form.30 Change in the deposited weld X X thickness (t).33 A change in the classification X.1 The addition of a position X Positions.2 A change of position X X QW-405.3 A change from vertical up to vertical down progression X.1 Decrease > 100 °F preheat X X Preheat temperature QW-406.3 Increase > 100 °F interpass X X temperature.1 A change in PWHT X X PWHT QW-407.2 A change in PWHT (time and X X temperature range).1 The addition or deletion of trail gas and/or change in composition X.2 A change from a single gas, X X mixture, or percentage.3 A change in the shielding gas flow X Gas rate or mixture QW-408.5 The addition or deletion of X backing gas or flow.9 The deletion of backing gas or a X X change in composition.10 The deletion of trail gas or a X X change in composition WELDING PROCESSES, INSPECTION, AND METALLURGY 35 Supplementary Essential Nonessential Article IV Paragraph Process Variable Essential WPS PQR WPS PQR WPS PQR.1 Heat input increase X X.3 The addition or deletion of a X pulsing current Electrical Characteristics.4 A change in current type (AC, DC) X X X and polarity QW-409.8 A change in the amperage range X.12 A change in tungsten electrode X type and size.1 A change in string or weave bead X.3 A change in orifice, cup, or nozzle X size.5 A change in the method of X cleaning.6 A change in the method of back X gouging.7 A change in oscillation technique X.9 A change from multiple to single X X X Technique pass welding per side QW-410.10 A change from single to multiple X X X electrodes.11 A change from closed to out of X X chamber.15 A change in electrode spacing X.25 A change from manual to X automatic.26 The addition or deletion of X peening.64 The use of a thermal process X X NOTE 1 WPS Contents: See QW-200.1(b). NOTE 2 PQR Contents: See QW-200.2(b). NOTE 3 ASME Section IX must be utilized in conjunction with this table; see Article IV, Weld Data. NOTE 4 Nonessential variables may be included on the PQR but are not required. Reference: ASME Section IX, Table QW-256, Welding Variables—Gas Tungsten—Arc Welding (GTAW). 6.2.2.2 Essential, Supplementary Essential, and Nonessential Variables for Gas Tungsten Arc Welding (GTAW) Supplementary Essential Nonessential Article IV Paragraph Process Variables Essential WPS PQR WPS PQR WPS PQR.1 Change in groove design X.5 The deletion of backing X Joints QW-402.10 Change in root spacing X.11 The addition or deletion of X retainers 36 API RECOMMENDED PRACTICE 577 Supplementary Essential Nonessential Article IV Paragraph Process Variables Essential WPS PQR WPS PQR WPS PQR.5 Change in the group number X X.6 Base metal thickness (T) limits Base Metals X X toughness QW-403.8 Base metal thickness (T) qualified X X.11 The change in P-number qualified X X.3 A change in the size of filler metal X.4 A change in the F-number X X.5 A change in the A-number X X.12 A change in the filler metal X X classification.14 The addition or deletion of filler X X Filler Metals metal QW-404.22 The addition or deletion of a X consumable insert.23 A change in the filler metal X X product form.30 Change in the deposited weld X X thickness (t).33 A change in the classification X.1 The addition of a position X Positions.2 A change of position X X QW-405.3 A change from vertical up to X vertical down progression.1 Decrease > 100 °F preheat X X Preheat temperature QW-406.3 Increase > 100 °F Interpass X X temperature.1 A change in the PWHT X X PWHT QW-407.2 A change in PWHT (time and X X temperature range).1 The addition or deletion of trail X gas and/or change in composition.2 A change from a single gas, X X mixture, or percentage.3 A change in the shielding gas X Gas flow rate or mixture QW-408.5 The addition or deletion of X backing gas or flow.9 The deletion of backing gas or a X X change in composition.10 The deletion of trail gas or a X X change in composition.1 Heat input increase X X.3 The addition or deletion of a X pulsing current Electrical Characteristics.4 A change in current type (AC, X X X DC) and polarity QW-409.8 A change in the amperage range X.12 A change in tungsten electrode X type and size WELDING PROCESSES, INSPECTION, AND METALLURGY 37 Supplementary Essential Nonessential Article IV Paragraph Process Variables Essential WPS PQR WPS PQR WPS PQR.1 A change in string or weave bead X.3 A change in orifice, cup, or nozzle X size.5 A change in the method of X cleaning.6 A change in the method of back X gouging.7 A change in oscillation technique X.9 A change from multiple to single X X X Technique pass welding per side QW-410.10 A change from single to multiple X X X electrodes.11 A change from closed to out of X X chamber.15 A change in electrode spacing X.25 A change from manual to X automatic.26 The addition or deletion of X peening.64 The use of a thermal process X X NOTE 1 WPS Contents: See QW-200.1(b). NOTE 2 PQR Contents: See QW-200.2(b). NOTE 3 ASME Section IX must be utilized in conjunction with this table; see Article IV, Weld Data. NOTE 4 Nonessential variables may be included on the PQR but are not required. Reference: ASME Section IX, Table QW-256, Welding Variables—Gas Tungsten—Arc Welding (GTAW). 6.2.2.3 Essential, Supplementary Essential, and Nonessential Variables for Gas Metal Arc Welding (GMAW) and Flux-Cored Arc Welding (FCAW) Supplementary Essential Nonessential Article IV Paragraph Process Variables Essential WPS PQR WPS PQR WPS PQR.1 Change in groove design X.5 The deletion of backing X Joints QW-402.10 Change in root spacing X.11 The addition or deletion of X retainers.5 Change in the group number X X.6 Base metal thickness (T) limits X X toughness Base Metals.8 Base metal thickness (T) X X QW-4