Amino Acids and Proteins PDF
Document Details
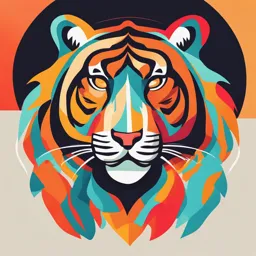
Uploaded by BrightPulsar
Tags
Summary
This document provides a comprehensive overview of amino acids and proteins. It covers their structures, functions, and classifications, including details on primary, secondary, tertiary, and quaternary structures. It also explores the properties of different amino acids and how these properties influence protein functions.
Full Transcript
AMINO ACIDS AND PROTEINS PROTEINS Involved in most activities of the organism; 50% dry weight of cells; most structurally sophisticated molecules known STRUCTURE linear, unbranched polymers constructed from 20 different alpha amino acids, encoded in the DNA of...
AMINO ACIDS AND PROTEINS PROTEINS Involved in most activities of the organism; 50% dry weight of cells; most structurally sophisticated molecules known STRUCTURE linear, unbranched polymers constructed from 20 different alpha amino acids, encoded in the DNA of the genome Vary in sizes mass single chain of proteins - typically 10-50 kdal; proteins as small as 350 dal and greater than 1000 kdal are known to exist Multichain protein complexes of greater than 200 kdal are frequently encountered FUNCTIONS OF PROTEINS 1. enzymatic catalysis – most proteins are enzymes 2. transport and storage of small molecules and ions; *HEMOGLOBIN, LIPOPROTEINS – carry lipids from the liver to the organs 3. structural elements of the cytoskeleton which: – provides strength and structure to cells – Forms the fundamental mechanistic components of intracellular and extracellular movement 4. structure of skin and bone- e.g. collagen, keratin 5. immunity – proteins like antibodies that mediate protective response to pathogens 6. hormonal regulation – some hormones are proteins (e.g. somatotropin or pituitary growth hormone and insulin) – cellular receptors that recognize hormones and neurotransmitters are proteins 7. control of genetic expression. Activators, repressors, and many other regulators of gene expression in prokaryotes and eukaryotes are proteins PROTEINS HAVE UNIQUE CONFORMATION SPECIFICITY of biologic function – consequence of uniqueness of the three-dimensional structural shape of conformation -physiological activity is linked to their three- dimensional structure -denaturation (change or alteration of its three dimensional structure due to extreme temp., changes in pH, variation in salt concentration) leads to reduction or loss of biological activity PROTEINS HAVE UNIQUE CONFORMATION Disease states –often related to altered function of a protein -related to anomaly in the protein structure *hemoglobinopathies (blood disorders and diseases that affect RBC) Sickle cell anemia Thalassemia – hemolytic anemia; insufficient production of α or β subunits of hemoglobin AMINO ACIDS- fundamental units of proteins COMPOSITION carboxyl (-COOH) group, amino group (NH2), H atom, variable group or side chain (R) all bonded to a carbon atom (α- carbon) Variable group characteristics: polar (hydrophilic), nonpolar (hydrophobic), acid or base with the exception of glycine all amino acids contain at least one asymmetric carbon atom and are therefore optically active Asymmetric carbon – carbon bonded to four different atoms or group of atoms AMINO ACIDS -exist as stereoisomeric pairs called enantiomers – L (levorotatory) or D (dextrorotatory) amino acid depending on the direction they rotate plane-polarized light Levorotatory (-) – leftwards; describes a chemical compound that rotates the plane of polarization of a transmitted beam of plane-polarized light or other electromagnetic radiation to the left, i.e. in an anticlockwise direction as viewed by the observer looking towards the light source. Dextrorotatory (+) – a chemical compound that rotates the plane of polarization of a transmitted beam of plane- polarized light to the right, i.e., in a clockwise direction as viewed by an observer looking toward the light source. AMINO ACIDS L amino acids – the only optically active amino acids that are incorporated into proteins. D-amino acids – found in bacterial products and in many peptide antibiotics but they are not incorporated into proteins via the ribosomal synthesizing system. D- amino acids would disturb the enzymatic reactions of L-amino acids and they are therefore broken down in the liver by the enzyme D-amino acid oxidase Stereoisomer – any of two or more isomers that have the same molecular constitution or formulas but differ in the arrangement of their atoms in space or in the 3-d arrangement of their atomic groupings in space L and D isomers of amino acids are mirror-images The four different substituents of an asymmetric carbon atom are assigned a priority according to atomic number. -lowest-priority substituent, often hydrogen, is pointed away from the viewer. -The configuration about the carbon is called S, from the Latin sinis-ter for “left,” if the progression from the highest to the lowest priority is counterclockwise -The configuration is called R, from the Latin rectus for “right,” if the progression is clockwise AMINO ACIDS amphoteric molecules – they have both basic and acidic groups Amino acids in solution at neutral pH exist predominantly as dipolar ions (also called zwitterions), have both positive and negative charges In the dipolar form, the α-amino group is protonated (-NH3+) and the α-carboxyl group is deprotonated (-COO-), thus the overall molecule is electrically neutral The ionization state of an amino acid varies with pH acid solution (e.g., pH 1) - amino group is protonated (-NH3+) and the carboxyl group is not dissociated (-COOH); overall charge of the molecule is positive As the pH is raised, the carboxylic acid is the first group to give up a proton, inasmuch as its pKa (dissociation constant) is near 2. The dipolar form persists until the pH approaches 9, when the protonated amino group loses a proton; overall charge is negative. AMINO ACID THREE-LETTER ONE-LETTER ABBREVIATION ABBREVIATION Alanine Ala A Arginine Arg R Asparagine Asn N Aspartic Acid Asp D Cysteine Cys C Glutamine Gln Q Glutamic Acid Glu E Glycine Gly G Histidine His H Isoleucine Ile I Leucine Leu L Lysine Lys K Methionine Met M Phenylalanine Phe F Proline Pro P Serine Ser S Threonine Thr T Tryptophan Trp W Tyrosine Tyr Y Valine Val V CLASSIFICATION OF AMINO ACIDS 1. non-polar – critically important for the processes that drive proteins to “fold”, to form their natural structures. -alkyl chain (R) groups Nonpolar side chain – does not gain or lose protons or participate in hydrogen or ionic bonds -side chains can be thought of as “oily” or lipid-like, a property that promotes hydrophobic Hydrophobic interactions between interactions amino acids with nonpolar side chains. LOCATION OF NONPOLAR AMINO ACIDS IN PROTEINS: -proteins found in aqueous solutions (polar environment) – side chains tend to cluster together in the interior of the protein known as hydrophobic effect w/c is the result of the hydrophobicity of the nonpolar R- groups which act much like droplets of oils that coalesce in an aqueous environment; nonpolar R groups thus fill up the interior of the folded protein and help give it its 3-d shape proteins found in hydrophobic environment (membrane) – nonpolar R-groups are found on the outside surface of the protein interacting with the lipid environment 2. Polar uncharged – contain R groups that can (1) form hydrogen bonds with water, and (2) play a variety of nucleophilic roles in enzyme reactions; have zero net charge at neutral pH, although the side chains of cysteine and tyrosine can lose a proton at an alkaline pH -more soluble in water than the nonpolar amino acids. Glycine - simplest amino acid, has only a single hydrogen for an R group; hydrogen is not a good hydrogen bond former -side chains of asparagine and glutamine each contain a carbonyl group and an amide group, both of which can also participate in hydrogen bonds --solubility properties are mainly influenced by its polar amino and carboxyl groups, and thus glycine is best considered a member of the polar, uncharged group -serine, threonine and tyrosine each contain a polar hydroxyl group that can participate in H bond formation 3. Acidic – contain carboxyl group; side chain carboxyl groups are weaker acids than the α-COOH group but are sufficiently acidic to exist ; -COO- at neutral pH. 4. Basic – have side chains with net positive charges at neutral pH -histidine – contains an imidazole group -arginine – contains a guanidino group -lysine – protonated alkyl amino group PROLINE – is an imino (-NH-) acid, not an α-amino acid -has an aliphatic side chain, side chain is bonded to both the nitrogen and the α- carbon atoms; -markedly influences protein architecture - its ring structure makes it more conformationally restricted than the other amino acids POSTRANSLATIONAL MODIFICATIONS -give rise to more than 100 different kinds of amino acids 1. Addition of hydroxyl (-OH) to some prolines and lysines in collagen 2. Addition of methyl (-CH3) groups to some lysines and histidines in muscle myosin 3. Addition of carboxyl groups (-COOH) to glutamates in blood clotting and bone proteins 4. Addition of phosphate (-PO3) groups to some serine, threonine and tyrosine molecules PEPTIDES AND POLYPEPTIDES PEPTIDE CHAINS – linking together of amino acids POLYPEPTIDES – many amino acids are linked Amino acids when in polypeptide chains, are customarily referred to as residues. PEPTIDE BOND (amide bond)- bond formed between the α–carboxyl group of one amino acid and the α –amino group of another; water is removed in the process PEPTIDE BOND FORMATION HIGHLY ENDERGONIC (energy-requiring) – requires hydrolysis of high-energy phosphate bonds PEPTIDE BOND FORMATION PLANAR – has two adjacent α–carbons, a carbonyl oxygen, α- amino nitrogen, and its associated hydrogen atom, and the carbonyl carbon all lying in the same plane Because of the resonance nature of the peptide bond, the C and N of the peptide bonds form a series of rigid planes -rotation within allowed torsion angles can occur around the bounds attached to the α-carbon; rotation is prevented around the bond axis (partial double-bond character of the C-N) peptide bonds are not broken by conditions that denature proteins such as heating or high concentrations of urea --can be hydrolyzed by prolonged exposure to a strong acid or base at elevated temperatures CHARACTERISTICS OF THE PEPTIDE BOND CONFORMATION OF PROTEINS CONFORMATION OF PROTEINS Protein in its native state has a unique three- dimensional structure (conformation) 1. Primary structure – linear sequence of amino acids joined together by peptide bond; covalent “backbone” of the polypeptide formed by the specific amino acid sequence -peptide sequences are written from left to right, starting with the amino acid residue that has a free α-amino group (N-terminal amino acid) and ending with the residue that has a free α-carboxyl group (C-terminal amino acid) -sequence is coded for by DNA and determines the final three-dimensional form adopted by the protein in its native state Example: 1. Pancreatic hormone insulin has two polypeptide chains, A (21 aa long) and B (30 aa long) linked together by disulfide bonds A Gly-Ile-Val-Glu-Gln-Cys-*Cys-Ala-Ser-Val-Cys-Ser- Leu-Tyr-Gln-Leu-Glu-Asn-Tyr-Cys-Asn B Phe-Val-Asn-Gln-His-Leu-*Cys-Gly-Ser-His-Leu-Val- Glu-Ala-Leu-Tyr-Leu-Val-Cys-Gly-Glu-Arg-Gly-Phe- Phe-Tyr-Thr-Pro-Lys-Ala 2. Sickle cell anemia – the beta chain of hemoglobin is 147 residues in length, yet a single amino acid substitution leads to the disease. In normal hemoglobin, the aa at position 6 is glutamate. In sickle cell hemoglobin, this glutamate is replaced by a valine 2. Secondary structure dictated by the primary structure arises from the interaction of neighboring amino acids IMPORTANT CHARACTERISTIC OF SECONDARY STRUCTURE -formation of hydrogen bonds (H bonds) between the –CO group of one peptide bond and the –NH group of another nearby peptide bond IMPORTANT CHARACTERISTIC OF SECONDARY STRUCTURE α –helix - H bonds form between peptide bonds in the same chain α–helix - rod-like structure with the peptide bonds coiled tightly inside the side chains of the residues protruding outward α–helix -Each –CO is hydrogen bonded to the –NH of a peptide bond that is four residues away from it along the same chain AMINO ACIDS THAT DISRUPT THE α–helix 1. charged amino acids - glutamate, aspartate, histidine, lysine, or arginine - disrupt the helix by forming ionic bonds, or by electrostatically repelling each other 2. amino acids with bulky side chains - tryptophan 3. amino acids that branch at the β-carbon (the first carbon in the R-group, next to the α-carbon) can interfere with formation of the α-helix if they are present in large numbers - valine or isoleucine 4. Proline disrupts an α-helix because its secondary amino group is not geometrically compatible with the right-handed spiral of the α-helix. Instead, it inserts a kink in the chain, which interferes with the smooth, helical structure. There are 3.6 amino acid residues per turn of the helix, and the helix is right-handed (i.e., the coils turn in a clockwise fashion around the axis) β –pleated sheet – H bonds form between peptide bonds in different chains; globular proteins, soluble proteins, fibrous proteins (e.g. silk fibroin) -pleated because the C-C bonds are tetrahedral and cannot exist in straight lines β –pleated sheet – chains lie side by side with the H bonds forming between the –CO group of one peptide bond and the –NH group of another peptide bond in the neighboring chain β –pleated sheet Parallel β pleated sheet – chains run in the same direction Antiparallel β pleated sheet – chains run in opposite direction (globular protein) β turn – tightest turn a polypeptide chain can make; results in a complete reversal in the direction of a polypeptide chain in just four amino acid residues. 3. Tertiary structure - spatial relations of more distant residues; attractions between α helices and pleated sheets Folding - secondarily ordered polypeptide chains of soluble proteins tend to fold into globular structures with the hydrophobic side chains in the interior of the structure away from the water and the hydrophilic side chains on the outside in contact with water 3. Tertiary structure Folding - folding is due to associations between segments of α–helix, extended β-chains, or other secondary structures and represents a state of lowest energy (greatest stability ) of protein in question CONFORMATION RESULTS FROM: 1. Hydrogen-bonding within a chain or between chains 2. The flexibility of the chain at points of instability, allowing water to obtain maximum entropy and thus govern the structure to some extent 3. The formation of other noncovalent bonds between side-chain groups, such as salt linkages, or π-electon interactions of aromatic rings 4. The sites and numbers of disulfide bridges between Cys residues within the chain (Cys residues linked by disulfide bonds are termed cystine residues) 4. Quaternary structure -refers to the spatial relations between individual polypeptide chains in a multichain protein; that is, the characteristic noncovalent interactions between the chains that form the native conformation of the protein as well as occasional disulfide bonds between the chains 1. Many proteins larger than 50 kdal have more than one chain and are said to contain multiple subunits, with individual chains known as protomers. 2. Many multisubunit proteins are composed of different kinds of functional subunits (e.g., regulatory and catalytic subunits of regulatory proteins) PROTEIN STRUCTURE-FUNCTION RELATIONSHIPS OXYGEN TRANSPORT PROTEINS 1. MYOGLOBIN –mediates the transport of oxygen in mitochondria; found in muscle cells; stores oxygen -to perform its function, myoglobin must be able to bind oxygen at the relatively low level oxygen tension in the tissues where hemoglobin releases oxygen. Myoglobin consists mainly of α-helices; heme group is shown in black, iron purple sphere STRUCTURE OF MYOGLOBIN Heme is formed when protoporphyrin IX binds with Fe2+ -hasa heme (prosthetic group – nonprotein group combined to protein) that enables the binding of oxygen STRUCTURE OF MYOGLOBIN Heme is formed when protoporphyrin IX binds with Fe2+ -functional heme is made of ferrous iron (Fe2+) and a protoporphyrin group called protoporphyrin IX composed of four linked pyrrole groups. -The iron is bound to the center of protoporphyrin IX by four nitrogen groups. -Heme fits into a hydrophobic pocket of the myoglobin molecule -without the heme (apoprotein form) myoglobin will not bind oxygen Folded tertiary structure of myoglobin, an oxygen- storage protein Each dot represents an amino acid The heme group, a cofactor that facilitates the binding of oxygen, is shown in blue OXYGEN BINDING SITE LIGAND BONDS – ferrous iron in heme can form six ligand bonds: (four bind the iron to the protoporphyrin ring, fifth bond binds the His residue to the F α- helix (His-F8) on the proximal side of the protoporphyrin ring, the sixth bond forms between an oxygen molecule interposed between the iron and a His residue in the E α-helix (His –E7) on the distal side of the protoporphyrin ring. OXYGEN BINDING -iron interacts with six ligands, four of which share a common plane; fifth and sixth ligands lie above and below the plane -four of the ligands are provided by the nitrogen atoms of the four pyrroles Fifth ligand – donated by the imidazole side chain of amino acid residue His F8 -Oxygen binds to the Mb Heme group OXYGEN BINDING Oxymyoglobin – oxygen molecule adds to the heme iron ion as the 6th ligand -oxygen adds end on to the heme iron but it is not oriented perpendicular to the plane of the heme – tilted 60⁰ with respect to the perpendicular OXYGEN-BINDING SITE HYDROPHOBIC POCKET – protects the ferrous iron group in heme from oxidizing to the ferric form (Fe 3+) -heme with a ferric iron is called metmyoglobin and it cannot carry oxygen; a water group replaces the oxygen in metmyoglobin -since ferric iron is an oxidized iron with extra electron, it cannot form coordinates needed to bind oxygen CARBON MONOXIDE Toxic gas because it binds with a higher affinity to free heme (20,000X better) than does oxygen, and 200X better than oxygen when heme is bound to myoglobin oxygen cannot displace it, acts like a potent competitive inhibitor CARBON MONOXIDE There might be plenty of oxygen available but the hemoglobin or myoglobin bound to CO will not carry it, oxygen will not be made available into the tissues CO effectively binds irreversible to the heme in myoglobin and hemoglobin 2. HEMOGLOBIN – found in RBCs, carries oxygen from the lungs to the tissues and carries CO2 from the tissues to the lungs. -humans – approximately 5B RBCs per ml of blood; each RBC contains 280 hemoglobin molecules. Arterial blood passing from the lungs, heart to the peripheral tissues = 96% saturated with oxygen Venous blood returning to the heart = 64% saturated -each 100 ml of blood passing through a tissue releases about ⅓ of the oxygen it carries or 6.5 ml of oxygen gas at atmospheric pressure and body temperature. STRUCTURE -spherical with a diameter of nearly 5.5 nm -tetrameric protein – w/ four heme prosthetic groups, one associated with each polypeptide chain Adults – two types of globins – two α chains (141 residues each) and two β chains (146 residues each) STRUCTURE OF HEMOGLOBIN TETRAMER – of four covalently bonded linked subunits each of which is structurally similar to myoglobin Each subunit has a hydrophobic pocket that contains heme and serves as the site of oxygen binding Heme prosthetic groups are identical to myoglobin STRUCTURE OF HEMOGLOBIN -strong interactions between unlike subunits – α1β1 interface (and its α2β2 counterpart) involves more than 30 residues -α1β2 (and α2β1) interface involves 19 residues Interactions: hydrophobic interactions (predominant), many H bonds, few ion pairs/salt bridges α = gray, β = blue FUNCTION OF HEMOGLOBIN OXYGEN-CARRIER IN THE BLOOD – must be able to bind oxygen at the relatively high PO2 in the lungs and release oxygen at the relatively low PO2 in the tissues Also carry CO2 from the tissues and release it in the lungs Hemoglobin Undergoes a Structural Change on Binding Oxygen Two Major conformations of Hemoglobin: 1. R (relaxed) state – oxygen has a higher affinity at this state -oxygen binding stabilizes R state; 2. T (tense) state – more stable in the absence of oxygen; predominant conformation of deoxyhemoglobin; low affinity state T state – stabilized by a greater number of ion pairs many of which lie at the α1β2 (and α2β1) interface (Blue = positively charged side chains involved in ion pairs; Red = negatively charged) Binding of oxygen to a hemoglobin subunit in the T state triggers a change in conformation to the R state. When the entire protein undergoes this transition, the structures of the individual subunits change little, but the αβ subunit pairs slide past each other and rotate narrowing the pocket between the β subunit. -some of the ion pairs that stabilize the T state are broken and some new ones are formed. T to R transition is triggered by changes in the positions of key amino acid side chains surrounding the heme. T state - porphyrin ring is slightly wrinkled causing the heme iron to protrude on the proximal His (His F8) side. Binding of oxygen causes the heme to assume a more planar conformation, shifting the position of the proximal His and the attached F Helix. These changes lead to adjustments in the ion pairs at the α1β2 interface. FACTORS THAT FAVOR THE T FORM 1. Low pH 2. High CO2 releases oxygen 3. High 2,3 BPG low oxygen affinity *low PO2 – respiring tissues FACTORS THAT FAVOR THE R FORM 1. High pH 2. Low CO2 binds oxygen 3. Low 2,3 BPG *high PO2 - alveoli The oxygen saturation curve for hemoglobin is sigmoidal -Each heme binds one O2 molecule = 4 O2 molecules per HbA molecule -HbA changes from T to R form when O2 binds Cooperative binding/positive cooperativity –binding of O2 to one heme group in Hb increases the affinity for O2 to the other hemes, produces a the sigmoidal oxygen saturation curve At a PO2 of 26 mmHg, Hb would have delivered 50% of its O2, while Mb would have delivered