Amino Acids and Proteins PDF
Document Details
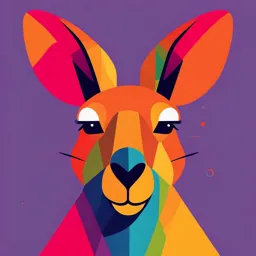
Uploaded by UnconditionalDrama5808
Kenyatta University
Tags
Summary
This document provides an overview of amino acids and proteins, including their structure, properties, and classification. It details the various types of amino acids and their roles in biological processes. It's a great resource for students learning about biochemistry.
Full Transcript
# PROTEINS Proteins are the most abundant organic molecules in animals, playing important roles in all aspects of cell structure and function. Proteins are biopolymers of acids, so named because the amino group is bonded to the carbon atom, next to the carbonyl group. The physical and chemical prop...
# PROTEINS Proteins are the most abundant organic molecules in animals, playing important roles in all aspects of cell structure and function. Proteins are biopolymers of acids, so named because the amino group is bonded to the carbon atom, next to the carbonyl group. The physical and chemical properties of a protein are determined by its constituent amino acids. The individual amino acid subunits are joined by amide linkages called peptide bonds. - **Structure of a general protein and its constituent amino acids.** The amino acids are joined by amide linkages called peptide bonds. - **Proteins have an amazing range of structural and catalytic properties** as a result of their varying amino acid composition. Because of this versatility, proteins serve an astonishing variety of functions in living organisms. ## Structure and Stereochemistry of the Acids Amino acids are the building blocks of proteins, and proteins are essential molecules that play a wide range of critical roles in the body. The simplest acid is amino acid, called glycine. Other common amino acids have side chains (symbolized by R) substituted on the carbon atom. For example, alanine is the amino acid with a methyl side chain. Here’s an overview of the structure of amino acids and proteins: 1. **Amino Group (NH2):** Amino acids contain an amino group (NH2) at one end of the molecule. This group is basic and can accept a proton (H+) to become positively charged. 2. **Carboxyl Group (COOH):** At the other end of the amino acid is a carboxyl group (COOH). This group is acidic and can lose a proton to become negatively charged. 3. **Central Carbon (α-Carbon):** A central carbon atom (α-carbon) links the amino group, carboxyl group, a hydrogen atom, and an R-group (side chain). The R-group varies among different amino acids and determines the unique properties of each amino acid. 4. **Hydrogen Atom (H):** A single hydrogen atom is attached to central carbon. 5. **R-Group (Side Chain):** The R-group is the variable part of the amino acid structure. It distinguishes one amino acid from another. Different R-groups give amino acids their unique properties, including size, charge, and hydrophobic or hydrophilic characteristics. Almost all the naturally occurring amino acids have the (S) configuration. They are called L-amino acids because their stereochemistry resembles that of L-glyceraldehyde. Amino acids combine many of the properties and reactions of both amines and carboxylic acids. The combination of a basic amino group and an acidic carboxyl group in the same molecule also results in some unique properties and reactions. The side chains of some amino acids have additional functional groups that lend interesting properties and undergo reactions of their own. Amino acids are often denoted by abbreviations of three letters (in most cases the first three letters of the name) or one letter. These are useful in writing out the long sequences of amino acids that occur in peptides and proteins. The standard amino acids are 20 common amino acids that are found in nearly all proteins. The standard amino acids differ from each other in the structure of the side chains bonded to their carbon atoms. All the standard amino acids are L-amino acids. ## Classification of amino acids Amino acids are classified according to the side chain, R; the most useful way to do this is according to their hydrophobicity, which determines their function in proteins. ## Nonpolar (Hydrophobic) Amino Acids: - These amino acids have nonpolar R-groups that do not interact well with water. - They are typically found in the interior of proteins. - Examples include glycine, alanine, valine, leucine, isoleucine, proline, methionine, and phenylalanine. ## Polar (Hydrophilic) Amino Acids: - These amino acids have polar R-groups that interact favorably with water. - They are often found on the surface of proteins and can form hydrogen bonds with water and other polar molecules. - Examples include serine, threonine, tyrosine, asparagine, glutamine, and cysteine. ## Aromatic Amino Acids: - Aromatic amino acids have a characteristic aromatic ring in their R-groups. - They can participate in pi-pi stacking interactions and are involved in the structural stability of proteins. - The two aromatic amino acids are phenylalanine and tyrosine. ## Acidic (Negatively Charged) Amino Acids: - Acidic amino acids have carboxyl groups (COOH) in their R-groups. - They can donate protons (H+) and carry a net negative charge at physiological pH. - Examples include aspartic acid (aspartate) and glutamic acid (glutamate). ## Basic (Positively Charged) Amino Acids: - Basic amino acids have amino groups (NH2) in their R-groups. - They can accept protons (H+) and carry a net positive charge at physiological pH. - Examples include lysine, arginine, and histidine. ## Sulfur-Containing Amino Acids: - A subset of amino acids contains sulfur in their R-groups. - Cysteine is notable for its thiol group (SH), which can from disulfide bonds with other cysteine residues, contributing to protein structure and stability. ## Amino Acids with Special Functions: - **Proline** is unique due to its cyclic structure, which introduces rigidity into the protein chain. - **Glycine** is the smallest amino acid, and its R-group is a single hydrogen atom. It imparts flexibility to protein structures. ## Amino Acids Involved in Phosphorylation: - Serine, threonine, and tyrosine are involved in protein phosphorylation, a crucial post-translational modification that regulates protein function and signaling pathways. The classification of amino acids based on their properties is essential for understanding the role of specific amino acids in protein structure and function. These properties influence how amino acids interact with each other within a protein and with their surrounding environment, ultimately determining the protein’s behavior and function in biological processes. ## Unusual Amino Acids Most proteins contain only the 20 amino acids listed above, but a few contain unusual amino acids such as 4-hydroxyproline and 3-hydroxylysine, which occur in collagen; γ-trimethyllysine, found in several Ca2+-binding proteins; γ-carboxyglutamic acid, 3-methyl histidine, pyroglutamic acid. They are formed by modification of the parent amino acid after incorporation into protein. ## IONIZATION OF AMINO ACIDS Amino acids can exist in different ionization states depending on the pH of the environment. This property is crucial for their function in biological systems. 1. **Amino Group (NH2):** The amino group of an amino acid can act as a weak base because it can accept a proton (H+). In its ionized form, the amino group becomes NH3+ (ammonium ion) after accepting a proton from water (H2O). The reaction is: $NH_2 + H_2O = NH_3^+ + OH^-$ 2. **Carboxyl Group (COOH):** The carboxyl group of an amino acid can act as a weak acid because it can donate a proton (H+). In its ionized form, the carboxyl group becomes COO- (carboxylate ion) after donating a proton to water. The reaction is: $COOH = COO^- + H^+$ 3. **Zwitterions (Ampholytes):** Amino acids in their natural state exist as zwitterions, which are molecules that have both a positively charged ammonium group and a negatively charged carboxylate group. The zwitterion has an overall neutral charge and represents the predominant form of amino acids at a specific pH known as the isoelectric point (pI). 4. **pH Dependence:** The ionization state of an amino acid is pH-dependent. The pKa values (acid dissociation constants) of the amino and carboxyl groups determine the pH at which an amino acid exists primarily in its ionized or non-ionized form. - At a pH below its pI, an amino acid exists primarily in its cationic (positively charged) form, with the ammonium group protonated. - At a pH above its pI, an amino acid exists primarily in its anionic (negatively charged) form, with the carboxyl group deprotonated. - At the pI, the amino acid is in its zwitterionic form, with equal numbers of positively and negatively charged groups. 5. **Buffering Capacity:** Amino acids can act as buffers, helping to maintain a stable pH in biological systems. They can accept or donate protons as needed to resist significant changes in pH. - **Ionization States** - At low pH, the amino acid exists primarily in its cationic form: $NH_3^+-R-COOH$ - **Zwitterionic Form (pKa1 < pH < pKa2):** As the pH rises, the carboxyl group loses a proton, leading to the zwitterionic form: $NH_3^+-R-COO^-$ This is typically the dominant form around the isoelectric point (pI). - **Fully Deprotonated Form (pH > pKa2):** At high pH, the amino group can lose a proton, resulting in a negatively charged species: $NH_2-R-COO^-$ - **pKa Values** - Each amino acid has specific pKa values: - $pK_a1$: Dissociation of the carboxyl group (typically around 2). - $pK_a2$: Dissociation of the amino group (typically around 9). - $pK_a3$: For amino acids with ionizable side chains, this represents the side chain’s dissociation (e.g., for aspartic acid, around 4.0). - **Titration Curves** The titration of an amino acid showshow the charge changes with pH. - **Buffer Region:** The pH range where the amino acid resists changes in pH due to the presence of both protonated and deprotonated forms. - **Inflection Points:** Correspond to pKa values, indicating where significant changes in charge occur. - **Importance of Ionization** - **Biological Activity:** The charge state of amino acids affects protein folding, stability, and interaction with other biomolecules. - **Enzyme Function:** Many enzymes rely on specific ionization states for their catalytic activity. - **Separation Techniques:** Ionization properties are utilized in techniques like electrophoresis and chromatography. ## The Isoelectric Point **Definition:** The isoelectric point (pI) is the pH at which an amino acid has no net charge. At this point, the positive and negative charges are balanced, and the molecule exists predominantly in its zwitterionic form. - **Key Concepts:** - **Zwitterion Formation:** At physiological pH (around 7.4), amino acids typically exist as zwitterions, where the amino group (-NH3+) is positively charged, and the carboxyl group (-COO-) is negatively charged. - **Calculating pI:** For amino acids with two functional groups (like the standard amino acids): $pI = \frac{pK_a1 + pK_a2}{2}$ Where: - $pK_a1$ is the dissociation constant of the carboxyl group. - $pK_a2$ is the dissociation constant of the amino group. - For amino acids with ionizable side chains (like glutamic acid or lysine), the pI calculation will involve the pKa values of the side chain as well. - **Acidic and Basic Amino Acids:** - **Acidic Amino Acids:** (e.g., glutamic acid, aspartic acid) - They have a side chain that can donate a proton, influencing the pI. - **Basic Amino Acids:** (e.g., lysine, arginine) - They have a side chain that can accept a proton, also affecting the pI. - **pI and Solubility:** At the pI, amino acids are less soluble in water, which is important for processes like protein precipitation. - **Applications:** Understanding pI is crucial in protein purification techniques (like isoelectric focusing) and can influence protein stability and function. - **Example Calculation:** For an amino acid like glycine: - $pK_a1$ (carboxyl group) = 2.34 - $pK_a2$ (amino group) = 9.60 - Calculating pI: $pI = \frac{2.34 + 9.60}{2} = 5.97$ - For an amino acid like aspartic acid (with an additional acidic side chain): - $pK_a1$ (carboxyl group) = 2.10 - $pK_a2$ (side chain carboxyl group) = 3.86 - $pK_a3$ (amino group) = 9.60 - Calculating pI: $pI = \frac{3.86 + 9.60}{2} = 6.73$ ## Stereoisomers All of the amino acids, except for glycine (Gly or G), have four different groups arranged tetrahedrally around the central C atom, and thus can exist in one of two stereoisomers. These two stereoisomers (or enantiomers) are nonsuperimposable, mirror images and are termed the D and L forms. Stereoisomers are physically and chemically indistinguishable by most techniques, but can be distinguished on the basis of their different optical rotation of plane-polarized light. Molecules are classified as dextrorotatory (D; Greek ‘dextro’ = right) or levorotatory (L; Greek ‘levo’ left depending on whether they rotate the plane of plane-polarized light clockwise or anticlockwise. Only the L-amino acids are found in proteins. D-Amino acids rarely occur in nature, but are found in bacterial cell walls and certain antibiotics. ## PROTEINS Proteins are complex macromolecules that play a wide range of essential roles in living organisms. Their structure can be described at multiple levels, each level providing insight into their function and properties. The primary, secondary, tertiary, and quaternary structures of proteins are the key levels of protein structure. 1. **Primary Structure:** The primary structure of a protein refers to the linear sequence of amino acids in the polypeptide chain. - The sequence is determined by the genetic code and is unique to each protein. - Alterations or mutations in the primary structure can profoundly affect a protein’s function. 2. **Secondary Structure:** Secondary structure refers to the local three-dimensional arrangements of amino acids in a protein chain. - The two most common secondary structures are alpha helices and beta sheets. - Alpha helices are coiled structures held together by hydrogen bonds between the amino and carboxyl groups of nearby amino acids. - Beta sheets are extended, sheet-like structures stabilized by hydrogen bonds between adjacent strands. - Secondary structures are important for the overall folding of a protein. 3. **Tertiary Structure:** Tertiary structure describes the overall three-dimensional shape of a protein. - It results from the interactions between amino acid side chains (R-groups) and includes various types of bonds and forces: - Hydrogen bonds: Stabilize the protein’s folding. - Disulfide bonds: Covalent bonds formed between cysteine residues. - Hydrophobic interactions: Nonpolar R-groups cluster together to minimize exposure to water. - Ionic interactions: Attraction or repulsion between charged R-groups. - Tertiary structure is essential for a protein’s function and determines its active sites and binding sites. 4. **Quaternary Structure:** Some proteins are composed of multiple polypeptide chains, or subunits, which come together to form the complete, functional protein. - The arrangement and interactions of these subunits in the quaternary structure are critical for the protein’s function. - Hemoglobin, for example, is atetrameric protein with four subunits. - Not all proteins have quaternary structures; some exist as single polypeptide chains. Protein structure is fundamental to their function. The precise folding and arrangement of amino acids enable proteins to carryout their specific roles in biological processes. The native, functional conformation of a protein is typically maintained through a delicate balance of noncovalent interactions, covalent bonds, and forces. Any disruption in a protein’s structure, such as denaturation (unfolding), can lead to a loss of function. Understanding protein structure is crucial in fields like biochemistry and molecular biology, as it allows scientists to comprehend the mechanisms of protein function, interactions, and potential targets for drug development.