Renal Corpuscle Filtrate Production PDF
Document Details
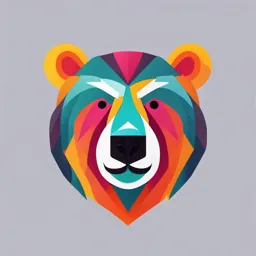
Uploaded by IndividualizedCactus
Tags
Summary
This document details the production of filtrate within the renal corpuscle, a vital part of urine formation. It explains the process of filtration, reabsorption, and secretion, while also describing the structure of the filtration membrane and the pressures associated with glomerular filtration. It is specifically aimed at undergraduate-level learning.
Full Transcript
24.5 Production of Filtrate Within the Renal Corpuscle Filtrate production occurs within the renal corpuscle. Water and solutes cross the filtration membrane and enter the capsular space. We first present a brief overview of the entire process of urine production and then consider details of filtrat...
24.5 Production of Filtrate Within the Renal Corpuscle Filtrate production occurs within the renal corpuscle. Water and solutes cross the filtration membrane and enter the capsular space. We first present a brief overview of the entire process of urine production and then consider details of filtrate production at the renal corpuscle. The processes that occur within the renal tubules are discussed in section 24.6. 24.5a Overview of Urine Formation LEARNING OBJECTIVE 19. Compare and contrast the renal processes of filtration, reabsorption, and secretion. Urine is formed in the kidneys through three interrelated processes: filtration, reabsorption, and secretion ( figure 24.10): Glomerular filtration passively separates some water and dissolved solutes from the blood plasma within the glomerulus. Water and solutes enter the capsular space of the renal corpuscle due to pressure differences across the filtration membrane. Collectively, this separated fluid is called filtrate, which as described, is essentially plasma minus large solutes (e.g., most proteins). Tubular reabsorption occurs when components within the tubular fluid move by membrane transport processes (e.g., diffusion, osmosis, active transport) from the lumen of the renal tubules, collecting tubules, and collecting ducts across their walls and return to the blood within the peritubular capillaries and vasa recta. Generally, all vital solutes and most water that were in the filtrate are reabsorbed, whereas excess solutes, some water, and waste products remain within the tubular fluid. Tubular secretion is the movement of solutes, usually by active transport, out of the blood within the peritubular capillaries and vasa recta into the tubular fluid. Materials are moved selectively into the tubules to be eliminated or excreted from the body. Remember, secretion results in excretion. Figure 24.10 Overview of the Processes of Urine Formation. The three major processes of urine formation are glomerular filtration, tubular reabsorption, and tubular secretion. (In this image, the nephron is “stretched out” for simplicity in understanding urine formation. In reality, a portion of the distal convoluted tubule (DCT) would be located much closer to the glomerulus and adjacent to the afferent arteriole.) (PCT = proximal convoluted tubule) APR Module 13: Urinary: Animations: Urine Formation Watch Video: Kidney Function Notice that the processes of tubular reabsorption and tubular secretion involve the movement of materials in opposite directions. In tubular reabsorption, substances are moved from the tubular fluid into the blood, whereas in tubular secretion, substances are moved from the blood into the tubular fluid. In addition, there is generally more tubular reabsorption than tubular secretion. WHAT DID YOU LEARN? 14 How does tubular reabsorption differ from tubular secretion? 24.5b Filtration Membrane LEARNING OBJECTIVE 20. Identify and describe the three layers that make up the glomerular filtration membrane. Page 960 The filtration membrane is a porous, thin (0.1 micrometer wide), and negatively charged structure that serves as a filter. It is composed of three sandwiched layers formed by two layers of the glomerulus and the visceral layer of the glomerular capsule ( figure 24.11). For a substance in the blood to become part of the filtrate, it must be able to pass through these three layers of the “filter,” from innermost (closest to the lumen of the glomerulus) to outermost: 1. Endothelium of glomerulus. The endothelium of the glomerulus is fenestrated (see types of capillaries in section 20.1c). It allows plasma and its dissolved substances to be filtered while restricting the passage of large structures, such as the formed elements (erythrocytes, leukocytes, and platelets). 2. Basement membrane of glomerulus. The porous basement membrane is composed of glycoprotein and proteoglycan molecules (see section 2.7e). It restricts the passage of large plasma proteins, such as albumin, while allowing smaller substances to pass through. 3. Visceral layer of glomerular capsule. The visceral layer of the glomerular capsule is composed of specialized cells called podocytes (pod′ō-sīt; podos = foot). Podocytes are octopus-like cells that have long, “footlike” processes called pedicels (ped′ĭ-sel; pedicellus = little foot) that wrap around the glomerulus to support the capillary wall but do not completely ensheathe it. The pedicels are separated by thin spaces called filtration slits, which are covered with membrane. Pedicels of one podocyte interlock with pedicels of a different podocyte, similar to how the fingers of your hands can interlock. The membranecovered filtration slits restrict the passage of most small proteins. Figure 24.11 Filtration Membrane. Filtrate is produced in the renal corpuscle when blood plasma is forced across the filtration membrane under pressure. (a) The filtration membrane is composed of three layers, including a fenestrated endothelium of the glomerulus, a basement membrane of the glomerulus, and membrane-covered filtration slits formed by podocytes of the visceral layer of the glomerular capsule. (b) Diagrammatic view of the three layers of the filtration membrane, including materials not filtered (left of membrane) and filtered (right of membrane). APR Module 13: Urinary: Histology: Podocyte: SEM High Magnification Mesangial (mes-an′jē-ăl) cells (more specifically, intraglomerular mesangial cells) are modified smooth muscle cells positioned between the capillary loops of the glomerulus. These pericytes (contractile cells around a capillary) have several diverse functions, which include: (a) forming the extracellular matrix (mesangial matrix) that functions as a structural framework to support the glomerulus, (b) releasing growth factors for the development and maintenance of glomeruli, and (c) releasing molecules that mediate inflammation (see section 22.3e). In addition, mesangial cells engage in (d) phagocytosis of unwanted substances that become trapped on the filtration membrane and (e) contraction to decrease the surface area of the filtration membrane. These last two functions are described in detail in sections 24.5c and 24.5e, respectively. INTEGRATE LEARNING STRATEGY 24.3 The filtration membrane is similar to an elaborate colander or sieve. Use the following to imagine the three layers that form this “sieve” that materials must move through to become filtrate: (1) The glomerulus is a drinking straw with small holes in it (the holes are the fenestrations of the endothelium). (2) The straw is wrapped in modeling clay (representing the basement membrane). (3) The third layer is represented by your hand wrapped around the clay-covered straw. Your hand represents a podocyte and the fingers of your hand, the pedicels. The spaces between your fingers are the filtration slits. If your fingers were thinly webbed, the webbing would represent the membranecovered filtration slits. L. Mouton/PhotoAlto Page 961 WHAT DO YOU THINK? 2 If a substance within the blood does not become part of the filtrate, what happens to it? By what structure do these substances exit the glomerulus? WHAT DID YOU LEARN? 15 How are the components of the filtration membrane of the glomerulus arranged? 24.5c Formation of Filtrate and Its Composition LEARNING OBJECTIVES 21. List examples of substances that are freely filtered, that are not filtered, and that are filtered in a limited way. 22. Describe the phagocytic function of mesangial cells. An average of 180 L of filtrate is produced daily by the kidneys through the glomerular filtration membrane. However, because of the size of the openings in the membrane and the overall negative charge across the membrane, not all substances within the blood plasma are filtered equally ( figure 24.11b). Consequently, substances in the blood can be placed into one of three categories based on the degree to which the substance is filtered. Freely filtered. Small substances such as water, glucose, amino acids, ions, urea, some hormones, water-soluble vitamins (i.e., vitamins B and C), and ketones can pass easily through the filtration membrane and become part of the filtrate. These substances have the same concentration of ions, molecules, and wastes in filtrate as in the plasma. Not filtered. Formed elements (i.e., erythrocytes, leukocytes, and platelets) and large proteins are structures that cannot normally pass through the filtration membrane. These substances are usually restricted from becoming part of the filtrate. Limited filtration. Proteins that are of intermediate size are generally not filtered. They are blocked from filtration either because their size prevents movement through the openings of the filtration membrane or because they are negatively charged and repelled by the membrane’s negative charge. Only limited amounts of these substances become part of the filtrate. Filtrate is characterized as filtered plasma with certain solutes and minimal amounts of protein. Filtrate is caught within the capsular space and then funneled into the proximal convoluted tubule. Components of blood that are not filtered exit the renal corpuscle through the efferent arteriole. The blood then continues through the peritubular and vasa recta capillaries (see figure 24.8). Some of the material being filtered becomes trapped within the basement membrane. One of the functions of the mesangial cells is to phagocytize macromolecules (e.g., antigen-antibody complexes) that become caught within the basement membrane, thus helping to keep the filtration membrane clean. WHAT DID YOU LEARN? 16 What is normally filtered across the filtration membrane? What is not normally filtered? 17 Certain diseases, kidney trauma, heavy metals, and some bacterial toxins can damage the filtration membrane. What effect would this have on relative permeability of the membrane and the substances that are filtered? 24.5d Pressures Associated with Glomerular Filtration LEARNING OBJECTIVES 23. Define glomerular hydrostatic pressure (HPg), and explain why it is higher than the pressure in other capillaries. 24. Name and describe two pressures that oppose HPg. 25. Explain how to calculate the net filtration pressure. 26. Define glomerular filtration rate, the factors that influence it, and the factors that it influences. Filtrate is produced due to the difference between the hydrostatic pressure of the blood in the glomerulus and the opposing pressures of both the osmotic blood pressure and the fluid pressure in the capsular space of the renal corpuscle. This difference determines the amount of filtrate formed. Glomerular Hydrostatic (Blood) Pressure Blood pressure in the glomerulus is called the glomerular hydrostatic (blood) pressure (HPg). It is the driving force that “pushes” water and some dissolved solutes out of the blood within the glomerulus and into the capsular space of the renal corpuscle ( HPg that promotes filtration. Figure 24.12 Pressures That Determine Net Filtration Pressure. Blood colloid osmotic pressure and capsular hydrostatic pressure are subtracted from glomerular hydrostatic pressure to determine net filtration pressure. Concept Overview Physiology Interactives figure 24.12). It is the HPg has a higher value than the blood pressure of other systemic capillaries (60 mm Hg versus about 20 to 40 mm Hg; see section 20.3c). This higher pressure is required for filtration to occur, and it is due to the relative diameter size difference in the afferent and efferent arterioles. The afferent arteriole has a larger internal diameter than the efferent arteriole. Consider that in this anatomic arrangement the “inflow pipe” is larger than the “outflow pipe.” This results in a higher pressure in the intervening structures (i.e., the glomerulus). As a consequence of this relatively high blood pressure, glomeruli are more vulnerable to damage than other capillaries. Page 962 Pressures That Oppose Glomerular Hydrostatic Pressure Two pressures oppose glomerular hydrostatic pressure and thus oppose filtration. They are the blood colloid osmotic pressure and capsular hydrostatic pressure. Blood colloid osmotic pressure (OPg) is the osmotic pressure exerted by the blood due to the unfiltered dissolved solutes it contains. The most important of these solutes are the plasma proteins (colloid). Blood colloid osmotic pressure opposes filtration because it tends to pull or draw fluids into the glomerulus. The typical value is 32 mm Hg. This is similar to the colloid osmotic pressure of 26 mm Hg within other systemic capillaries. Capsular hydrostatic pressure (HPc) is the pressure in the glomerular capsule due to the amount of filtrate already within the capsular space. The presence of this filtrate impedes the movement of additional fluid from the blood into the capsular space, and thus it also opposes filtration. A typical value is 18 mm Hg. Determining Net Filtration Pressure Filtration occurs if the pressure that promotes filtration, HPg, is greater than the sum of the pressures that oppose filtration (OPg and HPc). The difference in these pressures is the net filtration pressure (NFP). To determine NFP, use the following calculation: WHAT DO YOU THINK? 3 An individual with cirrhosis of the liver produces lower-than-normal amounts of plasma proteins. Which pressure is affected: HPg, OPg, or HPc? What do you predict regarding net filtration pressure: Will it increase or decrease as a result? Will more or less filtrate be formed? Explain. Variables Influenced by Net Filtration Pressure The glomerular filtration rate (GFR) is an important variable influenced by net filtration pressure. It is defined as the rate at which the volume of filtrate is formed, and it is expressed as volume per unit time (usually 1 minute). The net filtration pressure (NFP) directly influences the GFR. As NFP increases, usually as the consequence of HPg, the GFR also increases. Likewise, as NFP decreases, GFR decreases. NFP also influences other variables. As the NFP increases and GFR increases, more filtrate is produced. The increase in amount of filtrate results in increased fluid volume moving more rapidly through the tubules, so there is less time to reabsorb substances from the tubular fluid. Consequently, more substances remain in the tubular fluid and are excreted in the urine. The relationship of these variables to an increase in NFP is shown here: The significance of these relationships will be referred to throughout different remaining sections of the chapter. Specifically, notice the direct relationship between HPg and the amount of solutes and water remaining in the tubular fluid. As HPg increases, substances in the filtrate (e.g., NaCl) increase, and as HPg decreases, substances in the filtrate decrease. The amount of filtrate formed is monitored as fluid passes by the macula densa cells of the DCT within the JG apparatus. These cells specifically measure the level of NaCl remaining within the tubular fluid. Note that by monitoring NaCl levels in tubular fluid, the macula densa is indirectly measuring blood pressure in the glomerulus. This concept is discussed in more detail in section 24.5e. WHAT DID YOU LEARN? 18 What is the value of the NFP if the glomerular hydrostatic pressure (HPg) is 65 mm Hg, OPg is 30 mm Hg, and HPc is 20 mm Hg? 19 What happens to the value of the NFP in question 18 if the HPg increases from 65 mm Hg to 75 mm Hg? 20 If HPg increases, what is the effect on NFP? Is the relationship between HPg and NFP direct or inverse? 24.5e Regulation of Glomerular Filtration Rate LEARNING OBJECTIVES 27. Describe what is meant by intrinsic and extrinsic controls, and list examples of each. 28. Compare and contrast the myogenic response and the tubuloglomerular feedback mechanism, which are involved in renal autoregulation. 29. Explain the effects of sympathetic division stimulation on the glomerular filtration rate. 30. Describe the effects of atrial natriuretic peptide on the glomerular filtration rate. GFR is tightly regulated because of its influence on the amount of substances reabsorbed into the blood and the amount excreted in the urine. By being able to control the glomerular filtration rate, the kidney is able to control urine production based on physiologic conditions, such as state of hydration. GFR is primarily influenced both by changing the luminal diameter of the afferent arteriole (“the inflow pipe”) and by altering the surface area of the filtration membrane. The processes involved include (a) intrinsic control (within the kidney), which consists of renal autoregulation that maintains GFR at a normal level, and (b) extrinsic controls (external to the kidney) that involve both nervous system regulation, which decreases GFR, and hormonal regulation, which increases GFR. Renal Autoregulation: Intrinsic Controls Renal autoregulation is the intrinsic ability of the kidney to maintain a constant blood pressure and glomerular filtration rate despite changes (either a decrease or an increase) in systemic arterial pressure. This autoregulation allows the kidney to produce urine at a constant rate despite fluctuations in systemic blood pressure. Renal autoregulation functions by two mechanisms: the myogenic response and the tubuloglomerular feedback mechanism. Refer to figure 24.13 as you read this section on renal autoregulation. Figure 24.13 Renal Autoregulation. Renal autoregulation is the intrinsic ability of the kidney to maintain a normal glomerular filtration rate (GFR) despite changes in systemic blood pressure. The figures depict the response to (a) a decrease in systemic blood pressure, (b) normal resting systemic blood pressure, and (c) an increase in systemic blood pressure. Note here that the increased blood pressure may also stimulate tubuloglomerular feedback, which decreases filtration surface area. (d) Renal autoregulation is effective in maintaining normal glomerular filtration for mean arterial pressure (MAP) between 80 mm Hg and 180 mm Hg. The myogenic (mī′ō-jen′ik; mys = muscle, genesis = origin) response involves contraction and relaxation of smooth muscle in response to changes in stretch (see sections 10.10d and 20.4b). Here we are specifically interested in the response of the smooth muscle in the wall of the afferent arteriole in response to changes in stretch caused by either a decrease or an increase in systemic blood pressure. Page 963 Decrease in Systemic Blood Pressure A decrease in systemic blood pressure (as occurs when you are taking a nap) results in a lower volume of blood entering the afferent arteriole, reducing the stretch of the smooth muscle in the arteriole wall. The myogenic response of the smooth muscle cells in the vessel results in these smooth muscle cells relaxing, causing vasodilation of the afferent arteriole. The wider vessel lumen of the afferent arteriole allows more blood into the glomerulus, which compensates for the lower systemic blood pressure. Glomerular blood pressure and GFR remain normal ( figure 24.13a). Increase in Systemic Blood Pressure In contrast, an increase in systemic blood pressure (as occurs when you exercise) causes an additional volume of blood to enter the afferent arteriole, stretching the smooth muscle in the arteriole wall. Here, the myogenic response of the smooth muscle cells in the vessel results in the smooth muscle cells contracting, causing vasoconstriction of the afferent arteriole. The narrower vessel lumen of the afferent arteriole allows less blood into the glomerulus, which compensates for the greater systemic blood pressure. Glomerular blood pressure and GFR remain normal ( figure 24.13c). If the myogenic response is not sufficient to maintain normal glomerular blood pressure in response to an increase in systemic blood pressure, then glomerular blood pressure increases and the amount of NaCl remaining in the tubular fluid increases (see section 24.5d showing this relationship). Ultimately, an increase in tubular fluid NaCl concentration is detected by macula densa cells in the juxtaglomerular apparatus. This initiates the tubuloglomerular feedback mechanism. The macula densa cells respond by releasing a signaling molecule (most likely ATP). This signaling molecule causes paracrine stimulation (see section 17.3b) as follows: (a) It binds to and stimulates contraction of smooth muscle cells in the afferent arteriole wall. This results in further vasoconstriction of the afferent arteriole and a decreased volume of blood entering the glomerulus. (b) It also binds to and stimulates contraction of mesangial cells associated with glomeruli, which decreases the surface area of the filtration membrane. Both vasoconstriction of the afferent arteriole and the reduction in the filtration membrane surface area cause GFR and the amount of filtrate formed to return to normal levels. Page 964 You might find it helpful to think of the tubuloglomerular feedback as a “backup mechanism” to the myogenic response in response to increased systemic blood pressure. Limitations to Maintaining GFR It is possible to maintain the amount of urine formed in response to changes in systemic blood pressure through renal autoregulation, as just described. However, there are limitations to this regulation. Renal autoregulation is effective in maintaining a normal glomerular blood pressure only if the systemic mean arterial blood pressure (MAP; see remains between 80 and 180 mm Hg ( section 20.5a) figure 24.13d). A decrease in systemic blood pressure results in vasodilation of the afferent arteriole, with maximal arteriole dilation being reached at a MAP of 80 mm Hg. Further decreases in MAP can bring no further arteriole dilation, resulting in a decrease in glomerular blood pressure and glomerular filtration rate. If systemic blood pressure is extremely low (e.g., resulting from a severe hemorrhage), filtration and the elimination of wastes in urine cease, resulting in accumulation of toxic metabolic wastes in the blood. If systemic blood pressure increases, then the afferent arteriole vasoconstricts (and the mesangial cells may contract to decrease filtration membrane surface area). The afferent arteriole vessels are maximally constricted, and the filtration membrane is at its minimum surface area, at a MAP value of approximately 180 mm Hg. Further increases in systemic blood pressure can bring no further arteriole constriction or decrease in filtration membrane surface area, resulting in an increase in both glomerular blood pressure and GFR. Ultimately, the amount of urine increases. Neural and Hormonal Control: Extrinsic Controls The intrinsic controls of renal autoregulation, which were just described, help to maintain the GFR within normal homeostatic limits in response to changes in systemic blood pressure. In comparison, extrinsic controls, which are described here, involve physiologic processes to change GFR to adjust urine output based on physiologic need. GFR can be decreased when the kidney is stimulated by the sympathetic division, and it can be increased with atrial natriuretic peptide stimulation. Decreasing GFR Through Sympathetic Division Stimulation Activation of the sympathetic division as part of the fight-or-flight response (see section 15.4) results in a decrease in GFR through both vasoconstriction of the afferent arteriole and decreased surface area of the filtration membrane. The sympathetic division sends increased nerve signals to the kidneys during exercise or in an emergency ( figure 24.14a). Both afferent and efferent arterioles vasoconstrict as a result. Severe vasoconstriction of the afferent arteriole greatly reduces blood flow into the glomerulus. Glomerular blood pressure and GFR decrease. Figure 24.14 Extrinsic Mechanisms for Changing the Glomerular Filtration Rate Through Nervous and Hormonal Control. Glomerular filtration can be (a) decreased by sympathetic stimulation and (b) increased by atrial natriuretic peptide stimulation. Sympathetic stimulation also causes granular cells of the JG apparatus to release renin, with the subsequent production of angiotensin II (see section 20.6b). Angiotensin II stimulates mesangial cells to contract. This contraction results in a decrease in the surface area of the filtration membrane, and subsequently the GFR decreases. Thus, both vasoconstriction of the afferent arteriole and contraction of mesangial cells contribute to a decrease in GFR, with an accompanying decrease in urine production. Fluid is retained within the blood, maintaining blood volume. These critical adjustments allow the body to conserve fluid under stressful conditions when the sympathetic division is activated, such as during extensive exertion (e.g., running a marathon on a hot day) or severe hemorrhaging. Increasing GFR Through Atrial Natriuretic Peptide Atrial natriuretic (nā′trē-yū-ret′ik) peptide (ANP) increases GFR to eliminate fluid from the blood. This peptide hormone is released from atrial cardiac muscle cells into the blood in response to distension (stretch) of these chambers; increased stretch occurs when there is either an increase in blood volume return or an increase in blood pressure. ANP is transported in the blood to the kidney following its release from the heart. Atrial natriuretic peptide relaxes the afferent arteriole, causing vasodilation. ANP also inhibits the release of renin from the granular cells to ultimately cause relaxation of the mesangial cells, to increase filtration membrane surface area ( figure 24.14b). The net result is an increase in GFR that causes both an increase in urine volume and a decrease in blood volume. Thus, the release of ANP provides a means of increasing urine output to decrease blood volume and blood pressure to within normal limits. The complex interrelationship of the urinary, nervous, and endocrine systems in controlling systemic blood volume and blood pressure is discussed in detail in details for both angiotensin II and ANP are included in Balance, Blood Volume, and Blood Pressure.” chapter 25. Note: The table R.7: “Regulating Fluid Summary of GFR Regulation The glomerular filtration rate can be maintained, decreased, or increased by the following mechanisms: Renal autoregulation maintains GFR. If systemic blood pressure decreases, the afferent arteriole vasodilates (to increase GFR to normal levels). If systemic blood pressure increases, the afferent arteriole vasoconstricts (to decrease GFR to normal levels). Decreasing GFR to normal levels may be facilitated by contraction of mesangial cells, which decreases the filtration membrane surface area. Renal autoregulation is effective in maintaining GFR if MAP is within the range of 80 to 180 mm Hg ( figure 24.13). Direct stimulation by the sympathetic division decreases GFR by causing (a) vasoconstriction of afferent arterioles to decrease blood flow into the glomerulus and (b) renin release with the subsequent production of angiotensin II and contraction of mesangial cells, which decrease the surface area of the filtration membrane. Both GFR and urine production are decreased ( figure 24.14a). ANP increases GFR through (a) vasodilation of the afferent arteriole to increase blood flow into the glomerulus and (b) inhibition of renin release and the subsequent relaxation of mesangial cells, which increase the surface area of the filtration membrane. Both GFR and urine production are increased ( figure 24.14b). Figure 24.15 provides a visual summary of the structures and processes involved in glomerular filtration. INTEGRATE CONCEPT OVERVIEW Figure 24.15 Glomerular Filtration and Its Regulation. A visual summary of glomerular filtration, including (a) the filtration membrane and components of filtrate, (b) determining net filtration pressure, and (c) the mechanisms for controlling glomerular filtration. Concept Overview Physiology Interactives WHAT DID YOU LEARN? 21 Does urine production increase, decrease, or stay the same in response to an increase in glomerular filtration rate? 22 What are the three factors that regulate glomerular filtration rate? Does each of these increase, decrease, or maintain GFR? 23 Renal autoregulation is effective with a MAP between 80 and 180 mm Hg. Would renal autoregulation be effective in an individual with a blood pressure of 300/150 mm Hg? A pressure of 70/55 mm Hg? Explain. (See section 20.5a for formula for calculating MAP.)