Molecular Biology I: Nucleic Acid Metabolism Lecture 2 PDF
Document Details
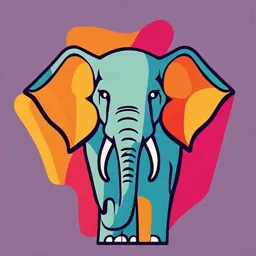
Uploaded by ZippyPelican
null
2024
SC/BIOL
Tags
Related
- BNUR 2003 Biomedical Chemistry & Lab Diagnostics PDF
- BMS100 Central Dogma Pre-learning Video 1: DNA PDF
- 2024S1-Lecture-01-updated PDF: Molecular Biology I
- Nucleic Acid Structure - Chapter 1 PDF
- Week 5 - Nucleic Acid Structure; Transcription F24 PDF
- Topic 14 Nucleic Acid Structure Lecture Notes 2024 PDF
Summary
This document is a lecture on nucleic acid metabolism, focusing on the structure and properties of DNA and RNA, and includes DNA topology. It is part of SC/BIOL 3110, 2024 S1, a molecular biology course likely offered at a university or college.
Full Transcript
MOLECULAR BIOLOGY I: NUCLEIC ACID METABOLISM SC/BIOL 3110, 2024 S1 Lecture 02: Structure and properties of DNA and RNA DNA topology 1 Recap of last lecture: What is Molecular Biology? Molecular Biology = Cell Biology + Biochemistry + Genetics 2 Recap of last lecture: What is Molecular Biology? P...
MOLECULAR BIOLOGY I: NUCLEIC ACID METABOLISM SC/BIOL 3110, 2024 S1 Lecture 02: Structure and properties of DNA and RNA DNA topology 1 Recap of last lecture: What is Molecular Biology? Molecular Biology = Cell Biology + Biochemistry + Genetics 2 Recap of last lecture: What is Molecular Biology? Paul Berg: First to create hybrid DNA molecules (splicing DNA from SV40 and l phage together) Used restriction enzymes as scissors to cut DNA and ligase to reform covalent bonds between compatible nucleotide ends 3 Recap of last lecture: Genetics = study of heredity à what factors carry the heritable traits? By the late 19th C, the molecule of inheritance was proposed to reside in the nuclei of cells What’s in the nucleus? Chemistry and Biochemistry found that the nuclei contain both protein and non-protein components à but which one? Cell biologists using dyes and microscopy discovered chromatin and chromosomes inside nuclei Recognized that segregation of chromosomes mirror the duplication and separation of Mendel’s particles of inheritance So then, what are on chromosomes? 4 Recap of last lecture: Answer: Genes à genotypes and phenotypes Morgan: discovered that the white eye phenotype in Drosophila is linked to the sex of the organism à suggested that eye colour gene is on the sex chromosome. Also found that some genes are linked (often inherited together) but most are inherited independently Hermann Muller found that genes can be mutated by chemical or radiological insults, and the mutated genes can be passed on from one generation to the next What do genes do? Beadle and Tatum à used a Forward Genetic screen approach to study the connections between genes and phenotypes 5 Recap of last lecture: Common approaches (genetic screens) for identifying gene functions: Step 1: Random mutagenesis Targeted mutagenesis Step 2: Identify and collect mutants that display desired phenotype Step 3: Identify genetic change in mutants à = gene responsible for phenotype studied 6 Recap of last lecture: What do genes do? à one gene, one enzyme/polypeptide model (Beadle and Tatum experiment) Screen and select for mutants that display specific phenotype à loss of ability to synthesize a specific amino acid Screen for growth: n + n à 2n mate mutagenized spores with WT spores Complete medium ✔ Minimal medium ✗ Minimal medium + specific aa ✔ 2n à n sporulate to get haploid spores that have identical mutations as original mutated spore isolate strain study what was changed 7 Recap of last lecture: Beadle and Tatum experiment: Isolated 4 mutant strains that grew on minimal medium only when supplemented with arginine At that time, knew that arginine biosynthetic pathway = multi-step process involving different enzymes 8 Recap of last lecture: To test hypothesis: 9 Recap of last lecture: What are genes made up of? Griffith’s transforming principle (heat killed S-strain can transform R à S strain) Difference between S and R strains = presence of polysaccharide coat 10 Recap of last lecture: What substance confers the ability to transform R à S? Avery, MacLeod, McCarty à Griffith’s transforming agent = DNA 11 Recap of last lecture: Hershey and Chase blender experiment: Which part of the bacteriophage is injected into the bacterium to make new phages? Supernatant Pellet Blender 12 Recap of last lecture: DNA is made up of 4 classic nitrogenous bases plus “other” ones “5th” base “6th” base …etc 13 Recap of last lecture: Deamination of nitrogenous bases can result in spontaneous mutagenesis Repaired before DNA rep à ok Not repaired before DNA rep à permanent mutation ? 14 Recap of last lecture: Deamination of nitrogenous bases can result in spontaneous mutagenesis Repaired before DNA rep à ok Not repaired before DNA rep à permanent mutation This is not as big of a problem as 5mC to T à why? 15 Recap of last lecture: Chargaff’s rule states that [A] = [T] and [C] = [G] The polymer chain of nucleotides have directionality (5’ to 3’) X-ray crystallography showed that DNA has helical structure with uniform width 16 Recap of last lecture: Watson and Crick à work mostly based on model building à competed with Linus Pauling who came up with the triple helix model à benefitted from Franklin’s data showing atomic dimensions and structure is helical with uniform width à proposed the double helix model For a more documentary-style account (17 min video), see: http://www.hhmi.org/biointeractive/double-helix 17 Recap of last lecture: Concept of complementarity: Base pairing and complementarity allow genetic information to be copied during DNA replication. 18 Properties of DNA structure: Key features of the double helix: The double helix is a right-handed helix. *For the topics of DNA and RNA structures, as well as DNA topology, read the Chapter posted on eClass for reference 19 Properties of DNA structure: Key features of the double helix: The double helix is a right-handed helix. The two strands of DNA are anti-parallel (run in opposite directions). Each helix has major grooves and minor grooves (where the stacked bases exposed to the outside). The major (and minor) grooves allow proteins (or small molecules) to bind specific DNA sequences without disrupting the double helix. 1 Å = 0.1 nm 20 Properties of DNA structure: Differences between major and minor grooves: The major groove is rich in chemical information – the characteristic patterns of H-bonding and overall exposed shape allow molecular distinction of different base pairs (e.g. A:T vs G:C, A:T vs T:A) Major grooves are big and can accommodate insertion of amino acid side chains –allow proteins to bind in sequence-specific manner. Minor grooves are less able to accommodate amino acid side chains and the exposed surfaces do not allow unambiguous distinction between base pairs (e.g. A:T similar to T:A). 21 Properties of DNA structure: Differences between major and minor grooves: The major groove is rich in chemical information whereas the minor groove is not 22 Properties of DNA structure: Distinct forms/conformations of DNA helices: There are multiple conformations of DNA helices that can form under various in vitro conditions. The B-form of DNA represents DNA found under physiological conditions. The A-form of DNA is shorter and stubbier and found in less hydrated conditions. (can convert B-form DNA to A-form by de-hydration) The A-form helix can also be adopted by RNA-DNA and RNA-RNA helices. The Z-form of DNA has a zig-zag pattern – this form has been found in vivo in prokaryotes but not in eukaryotes so far. The Z-form of DNA contains some purine nucleotides that are in the syn conformation. 23 Properties of DNA structure: Distinct forms/conformations of DNA helices: Top view Note: hole in the centre bases in the centre Side view 24 Properties of DNA structure: Distinct forms/conformations of DNA helices: Can you spot what else is distinct about Z-DNA (compared to A- and B-DNA)? 25 Properties of DNA structure: Comparison of the 3 forms of DNA helices: 26 Properties of DNA: What factors contribute to the stability of dsDNA? 1. Hydrogen bonding between base pairs The hydrogen bonds between complementary bases are a fundamental feature of the double helix, contributing to the thermodynamic stability of the helix and the specificity of base pairing A:T – 2 hydrogen bonds G:C – 3 hydrogen bonds Hydrogen bonds – relatively weak*, and a G:C pair is more stable than the A:T pair * is there any advantage to this? 27 Properties of DNA: What factors contribute to the stability of dsDNA? 2. Base stacking: Base surfaces are hydrophobic. As a result, the most energetically favoured conformation is attained by reducing exposure of the base surfaces to the aqueous environment, which is achieved by the bases stacking on top of one another The van der Waals interactions between bases are weak, but the large number of these interactions within the helix help stabilize the overall structure 28 Properties of DNA: What factors contribute to the stability of dsDNA? 2. Base stacking: Because of the helical nature of DNA, each stack of base pair is rotated relative to the adjacent stack You should be able to figure out the degree of each turn? (assuming there are 10.5 bp per turn) 29 Properties of DNA: What factors contribute to the stability of dsDNA? i.e. what keeps the two strands of a duplex together? A. Forces that keep the strands together: 1. Hydrogen bonding between base pairs A A 2. Base stacking B. Forces that push the strands apart: 1. Electrostatic repulsion: e.g negatively charged phosphate backbone B B Note: under physiological conditions, A >> B, so DNA is very stable 30 Properties of DNA: What factors contribute to the stability of dsDNA? Base pairing through hydrogen bonding is the molecular basis of complementarity ✔ ✔ YES NO ✗ 31 Properties of DNA Concept of complementarity: Moreover, complementarity of the DNA strands also allows for the denature and renature properties of ds DNA 32 Properties of DNA Concept of complementarity: The complementary strands of ds DNA can come apart when heated above physiological temperatures (to near 100 oC) or under conditions of high pH (i.e. alkali conditions), a process known as denaturation This process is reversible – when cooled slowly, the complementary strands of DNA will come together and reform the double helix. This is also called renaturation Explains why Griffith’s heat-killed bacteria can still transfer genetic material 33 Properties of DNA Complementarity and hybridization: The capacity to renature denatured DNA molecules allows formation of hybrid DNA molecules by cooling mixtures of denatured DNA from two different sources This process, also known as hybridization, is the molecular basis for many molecular biology techniques used to manipulate DNA and the genome (will revisit this topic in later lectures) 34 Properties of DNA UV absorption and hyperchromicity: The rings of the nucleotide bases are made of alternating single and double bonds, which has the ability to absorb UV light Each of the 4 nucleotides have different absorption spectrum, and the UV absorption of DNA is the average of all the nucleotides For pure DNA, peak of absorption is at 260 nm (UV light) Interestingly, ds DNA absorbs less UV than ss DNA due to base stacking of the helix In general, ss DNA absorbs ~ 3040% more UV than ds DNA By convention: 1U (A260) = 50 µg/ml of ds DNA 1U (A260) = 33 µg/ml of ss DNA 35 Properties of DNA UV absorption and hyperchromicity: This unique property allows one to measure the transition of ds DNA to ss DNA during denaturation simply by measuring the UV absorption of the DNA solution E.g., increasing the temperature of a DNA solution (to denature ds DNA) results in a jump in A260. This phenomenon is called the hyperchromic shift The sharpness of this curve indicates that DNA denaturation and renaturation are highly co-operative reactions 36 Properties of DNA DNA denaturation or melting: The separation of the two DNA strands is often referred to as “melting” The mid-point of the hyperchromic shift is also known as the melting temperature (Tm), which refers to the temperature at which 50% of the ds DNA has melted 37 Properties of DNA DNA denaturation or melting: Tm is a very important parameter for all hybridization-based molecular biology techniques The Tm is affected by the G:C content of the DNA (the higher the G:C content, the higher the Tm) The Tm is also affected by the ionic strength of the solution. The higher the salt concentration, the higher the temperature needed to melt ds DNA – why? 38 Properties of DNA DNA denaturation or melting: Low [salt] * High [salt] * Tm shifts higher with increasing salt 39 Properties of DNA DNA denaturation or melting: Taking the various factors into consideration, the following formula is used to calculate melting temperature of DNA strands: Tm = 81.5 + 16.6(log10([Na+]) + 0.41(%GC) – 600/length where [Na+] is the molar sodium concentration, (%GC) is the GC ratio, and length is the length of the sequence. There are many different formulae for calculating Tm, all likely giving slightly different numbers à note you are calculating the theoretical Tm In the lab, especially for short oligos, we often use the quick formula below: Tm = 4oC x (number of G’s and C’s) + 2oC x (number of A’s and T’s) Note: you only count the # Gs/Cs and As/Ts on one strand (e.g. the ss primer for PCRs) Calculating Tm is very important for the various techniques based on DNA-DNA or DNA-RNA hybridization. E.g. PCR, Southern blots, Northern blots etc 40 Properties of DNA Other factors that affect DNA stability: The pH of the solution also affect DNA stability Neutral pH allow hydrogen bonding to occur Alkaline conditions denature DNA (breaks hydrogen bonds) Acidic conditions leave apurinic sites, cleaves glycosidic bond and render DNA susceptible to hydrolysis As a side note, alkaline conditions break the phosphodiester bonds in RNA and hydrolyzes RNA. DNA is much more stable in alkaline conditions (even though it gets denatured) 41 RNA structures: Differences between RNA and DNA: 1. Deoxyribose vs ribose sugars: DNA vs RNA missing -OH (alcohol) group 42 RNA structures: Differences between RNA and DNA: 2. DNA has thymine as base whereas RNA replaces thymine with uracil. Found in DNA Found in RNA 43 RNA structures: Differences between RNA and DNA: 3. RNA polynucleotide chain also has directionality (5’ to 3’), but no complementary strand 5’ 3’ 44 RNA structures: Differences between RNA and DNA: Some figures depict RNA as a half helix … 45 RNA structures: Differences between RNA and DNA: Some figures depict RNA as a half helix … NOT LIKELY! More likely like this… 46 RNA structures: RNA strands have secondary and tertiary structures: RNAs are single stranded molecules, but often can fold back and form short stretches of base-pairing. RNAs that have two stretches of complementary sequence near one other can form stem-loops in which the intervening RNA is looped out. Base pairing can also take place between sequences that are not contiguous to form complex structures such as pseudoknots. Typical stem loop Formation of pseudoknot due to base pairing of non-contiguous sequences 47 RNA structures: RNA strands have secondary and tertiary structures: RNA secondary structures are based on conventional Watson-Crick base pairing, as well as non-Watson-Crick base pairing, such as the G:U wobble. G:U base pairing is stabilized by hydrogen bonding between N3 of uracil and the carbonylon C6 of guanine and between the carbonyl on C2 of uracil and N1 of guanine Note that the thermostability of G:U pairing is just as stable as A:T Watson-Crick base pairing. Also, having an additional permissive base pair increases the possibility of selfcomplementarity of RNA à can have elaborate secondary structures! 48 RNA structures: RNA strands have secondary and tertiary structures: For example, tRNAs (or aminoacyl transfer RNAs) have a distinct 4 cloverleaf secondary structure. Note the multiple G:U base pairing that helped stabilize the secondary structure. 49 RNA structures: RNA strands have secondary and tertiary structures: The tRNA molecule further folds back on itself to form a tertiary (3D) structure, which contains 2 helical domains. Tertiary structure of a typical tRNA Another figure illustrating the two helical domains of a typical tRNA 50 RNA structures: RNA strands have secondary and tertiary structures: As illustrated by the tRNA molecules, RNAs can also form short helices. However, the 2’ OH on the ribose of RNA prevents the adoption of a B-form helix, so the helices formed by RNA molecules adopt a structure closer to the A-form of DNA duplex. The double helix formed by RNA is structurally distinct from the DNA double helix, and is less suited for sequence-specific interactions with proteins à remember why? 51 RNA structures: Types of RNAs: Three common types of RNA found in all prokaryotes and eukaryotes: mRNA (messenger RNA) – protein encoding à specifies the order of amino acids for different proteins. tRNA (transfer RNA) – interprets the information on mRNA and delivers individual amino acids to the mRNA being translated on the ribosomes. rRNA (ribosomal RNA) – RNA component of ribosomes (RNA/protein complexes where protein synthesis occurs). Relative abundance of these RNAs in an E. coli cell: rRNA 80% tRNA 15% mRNA 5% 52 RNA structures: Catalytic RNAs: First discovered in the 1980s by Tom Cech et al. Class of RNAs that have catalytic activities – counter to previous belief that only enzymes (proteins) have catalytic activities (hence also known as ribozymes). Ribozymes increase the rate and specificity of phosphodiester bond cleavage of RNA molecules 53 RNA structures: Catalytic RNAs: The biological reactions catalyzed by ribozymes mainly involve phosphoester cleavage or sometimes transfer and religation to another nucleotide The cleavage reaction often involves deprotonation of 2’ OH, which initiates nucleophilic attack on the neighbouring 3´-phosphate, forming 2´-3´ cyclic phosphate à same reaction as RNA hydrolysis in a basic solution cyclic phosphate = transesterification reaction See video file posted on eClass (under Addition Resources) for animation 54 RNA structures: Catalytic RNAs: 1. 1st example: RNaseP = a ribonuclease involved in tRNA processing RNaseP contains both RNA and protein, but RNA alone has catalytic activity 55 RNA structures: Catalytic RNAs: 2. Other examples: Self-splicing introns (found in rRNAs of some organisms) Action of self-splicing ribozymes – removing an intron by selfcatalyzing the splicing reaction. For Group II self-splicing introns, a reaction between the C2’ hydroxyl group of ribose in nucleotide A (right figure) and a target nucleotide in the upstream exon I leads to the breaking of a 5′–3′ phosphoester bond at the end of this exon. One of the cut ends is joined to the ribose C2’ in nucleotide A, closing the intron circle. A reaction between the other cut end and nucleotide A severs the intron tail, leaving the ends of the two exons to be sealed together. 56 RNA structures: Catalytic RNAs: 3. Other examples: Hammerhead ribozymes sequence-specific ribonuclease found in some infectious RNA agents of plants known as viroids viroid RNA genome replicates by a rolling circle mechanism à tandem multicopy genomes – undergo self-cleavage to yield single copy genomes need to acquire 3D architectures to promote specific interactions with cofactors, especially divalent metal ions, and other functional domains for cleaving RNA substrates 57 RNA structures: Catalytic RNAs: 3. Other examples: Hammerhead ribozymes the catalytic domain is made up of 3 stem loops with Stems I and III basepairing with target sequence flanking the cleavage site cleavage reaction involves nucleophilic attack of a 2´-OH on the target 3´phosphate cleavage site 58 RNA structures: Catalytic RNAs: 3. Other examples: Hammerhead ribozymes Hammerhead ribozymes mostly catalyzes cleavage of itself, but can be engineered to catalyze cleavage of target RNA molecules Ribozyme Ribozyme Engineered hammerhead ribozyme designed to anneal to target mRNA can cleave at a specific sequence. 59 RNA structures: Other functional RNAs: ncRNAs (non coding RNAs) – functional RNAs that are not translated into proteins à no detectable ORFs (open reading frames) or no proteins found. tRNAs and rRNAs technically fall into this category Other long ncRNAs (lncRNA), such as XIST and HOTAIR, functions in gene expression regulation; however, their mechanisms of action still unclear. For example, XIST is transcribed from one of the two X chromosomes in female cells. The XIST RNA then coats the same X chromosome that transcribed it, and function to inactivate expression of most of the genes on that chromosome. This process, known as X-inactivation, functions to balance the output of genes located on the X chromosome in females (= dosage compensation). 60 RNA structures: Other functional RNAs: A very simplified representation of XIST and X-inactivation: 61 RNA structures: Other functional RNAs: siRNAs (short interfering RNAs) and miRNAs (micro interfering RNAs) à Both are RNA-mediated mechanisms to inhibit gene expression siRNAs: o ~21nt long RNAs, could be processed from longer RNAs o Often from exogenous (foreign) sources (e.g. viruses that infect plant cells) o Target mRNAs that have perfect complementary sequences and use host machinery to cleave target mRNAs à loss of expression miRNAs: o ~ 22 nt RNAs processed from longer pri- and pre-miRNAs o Transcribed from endogenous genome o Target mRNAs that have perfect or mostly complementary sequences o Use host machinery to either cleave target mRNAs or block their translation 62 DNA topology Topology is the mathematical study that describes properties of topologically constrained objects when they are deformed (e.g. stretched or twisted) 63 Properties of DNA DNA topology: Because the two chains of the double helix are intertwined, they are under topological constraints when the ends of the DNA molecules are fixed. This is true for linear ds DNA fixed at both ends, or for covalently closed circular DNA, which has no ends. OR Both of these are examples of “closed systems” 64 Properties of DNA DNA topology: The problem of topological constraints is especially relevant to processes such as transcription and DNA replication, which require unwinding of the DNA duplex 65