Topic 14 Nucleic Acid Structure Lecture Notes 2024 PDF
Document Details
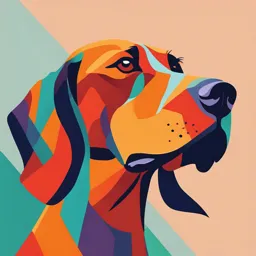
Uploaded by ResplendentHydrogen
Western University
2024
Tags
Summary
These lecture notes provide a detailed explanation of nucleic acid structure, focusing on the chemical compositions of DNA and RNA, their structures, and common terms. The notes include details on important concepts like B-DNA, base pairing, and nucleotides. Ideal for undergraduate-level study of biochemistry or genetics.
Full Transcript
Topic 14 Nucleic Acid Structure Readings: Sections 3.1, 3.2, 20.5 (Eukaryotic DNA is packaged in nucleosomes) By the end of this topic, you should be able to: Explain the chemical structure of nucleic acid polymers, without memorizing the s...
Topic 14 Nucleic Acid Structure Readings: Sections 3.1, 3.2, 20.5 (Eukaryotic DNA is packaged in nucleosomes) By the end of this topic, you should be able to: Explain the chemical structure of nucleic acid polymers, without memorizing the structures of the nitrogenous bases Describe B-DNA and the higher order structures formed by RNA, and the important forces that stabilize these structures Predict the impact of changes in sequence or conditions on the melting temperature of a DNA double helix Identify chemical and structural similarities and differences between DNA and RNA Explain the different levels of structural organization displayed by DNA in eukaryotic cells Describe the structure of the nucleosome The two major types of nucleic acid in living systems are ribonucleic acid (RNA) and deoxyribonucleic acid (DNA). DNA is a crucial molecule, because it holds the genetic material, the information passed from parent(s) to offspring that is required to produce an organism. RNA has several different functions, including acting as the template from which proteins are produced (messenger RNA or mRNA), forming the ribosome (along with proteins) and catalyzing protein synthesis (ribosomal RNA or rRNA), and carrying amino acids to the growing peptide chain during protein synthesis (transfer RNA or tRNA). RNA is also involved in gene regulation and processes like mRNA splicing and telomere maintenance. RNA and DNA are linear polymers composed of ribonucleotide and deoxyribonucleotide monomers, respectively. Each monomer is composed of three parts: a monosaccharide, a nitrogenous base, and a phosphate (see Guided Tour, Nucleic Acid Structure). Monosaccharides Ribonucleotides contain the pentose ribose. Deoxyribonucleotides contain deoxyribose, which is like ribose, except that C2' bears two hydrogen atoms, instead of one hydrogen and one hydroxyl. Nitrogenous bases There are two types of nitrogenous base: purines and pyrimidines. All purines and pyrimidines are planar and relatively hydrophobic. The two purines found in DNA and RNA are adenine (A) and guanine (G). The three pyrimidines are cytosine (C), which is found in both DNA and RNA, thymine (T), which is found in DNA but not RNA, and uracil (U), which is found in RNA but not DNA. Uracil is identical to thymine except that uracil lacks the methyl (-CH 3 ) group. Purines and pyrimidines are joined to the anomeric (1') carbon of ribose or deoxyribose via an Nglycosidic bond. The anomeric carbon is ordinarily in the β configuration when joined to a nitrogenous base. A sugar joined to a nitrogenous base is called a nucleoside. The five common nucleosides are adenosine, guanosine, cytidine, thymidine, and uridine (Table 3.1). Phosphates Phosphates are attached to the 5' position of ribose or deoxyribose by a phosphoester linkage. After a phosphate is attached to this position of a nucleoside, a nucleotide is formed (i.e., a nucleotide has all three groups: a sugar, a base, and one or more phosphates). Phosphate groups are negatively charged at cellular pH. The names of nucleotides are usually abbreviated using the letter for the nitrogenous base followed by an indication of the number of phosphates present (e.g., ADP for adenosine diphosphate). Sometimes (but not always) the abbreviation for a deoxyribonucleotide will begin with a small d (e.g., dTTP). Polynucleotide Structure Mononucleotides are linked together to form an unbranched polynucleotide chain through 3',5' phosphodiester bonds. That is, the phosphate group attached to the 5' carbon of one pentose forms a phosphoester bond with the hydroxyl group at the 3' position of another pentose (see Guided Tour, Nucleic Acid Structure, and Chapter 3.2). The end of a polynucleotide at which the 5' carbon is “free”, or not attached to another monosaccharide, is called the 5' end; likewise, at the 3' end the 3' carbon is free. The sequence of the polynucleotide is represented by giving the letter for each nucleotide base in order, e.g., CAAGTG. By convention polynucleotides are written in the 5' to 3' direction from left to right, unless otherwise indicated. Usually, the context makes it clear whether the polynucleotide is DNA or RNA. All organisms have natural processes to synthesize DNA and RNA with desired sequences (see Topics 15, 18, and 19). Scientists have developed accurate, automated chemical methods to synthesize single-stranded oligonucleotides (or “oligos” for short) of up to about 200 base pairs. Longer sequences can be obtained by joining strands together. Single-stranded oligos have many uses in molecular biology (e.g., as primers for DNA sequencing or PCR), as outlined in Topic 22. The double helix A single strand of DNA is normally not found in the cell. Almost always, two polynucleotide DNA strands associate with each other non-covalently. The strands are antiparallel; that is, they have opposite orientation or chemical "polarity". One strand runs in a 5' to 3' direction, and the other runs 3' to 5': 5' 3' 3' 5' The two polynucleotides are wrapped around each other to form a double helix (Fig 3.3). The double helix is normally right-handed, meaning that if you look at the helix along its axis, and move your gaze along one strand from a point close to you to a point farther away, the curve is in a clockwise direction (Can you relate this to the structure of your right hand?) The base pairs lie on the inside and the phosphates are on the outside. This arrangement minimizes charge repulsion between the phosphates and allows for salt stabilization of the phosphates’ negative charges. Two types of interactions between the bases stabilize the double helix: 1. Base stacking The bases are planar aromatic rings that are nearly perpendicular to the helical axis. Their flat surfaces allow them to stack on top of each other, stabilized by interactions between transient induced dipoles in the rings (known as dispersion forces or London forces). The atoms in each base pair lie at their optimal van der Waals radius from the adjacent base pair. Base stacking is not sequence-specific, in that any base can stack on top of any other base. Base stacking interactions are the major contributor to the stability of the double helix. 2. Base pairing Bases also interact through hydrogen bonds between chemical groups on the edges of their ring structures. These interactions are sequence-specific. In normal double-stranded DNA, A and T base pair with each other and no other base; likewise, G and C base pair exclusively with each other. When the bases are positioned appropriately, the hydrogen bond donors and acceptors of these pairs are perfectly complementary to each other. The AT pair forms two hydrogen bonds, and the GC pair forms three. These base pairing arrangements are called Watson-Crick base pairing, after James Watson and Francis Crick, the scientists who first proposed the correct structure of DNA (though credit should also have been given to Rosalind Franklin). Because A pairs only with T, and G pairs only with C (and vice versa), the sequence on one strand dictates the sequence on the other (i.e., if you know the sequence of one strand, you know the sequence of them both; such strands are said to be complementary strands). Note that each base pair consists of one purine and one pyrimidine base, giving a uniform base pair size, so that the two sugar-phosphate backbones stay the same distance apart. Also, because water is excluded from the interior of the double-helix, it cannot compete with the bases for hydrogen bonding positions. DNA can form different types of double helices, with different geometries. In cells, DNA usually adopts a structure called the “B” form. Features of the B-DNA double helix (Fig 3.3) are: it is narrow, about 2 nm (20 Å) wide it can be very long; human chromosome 1 consists of 245 million base pairs the distance between consecutive bases is about 0.34 nm (so chromosome 1 would be about 8.3 cm long if it were stretched out in a straight double helix) Because of the positions at which the bases are attached to the sugar-phosphate backbone and the arrangement of hydrogen bonding donors and acceptors, the two grooves between the sugarphosphate backbones are not equal in size. The wider groove is called the major groove, and the narrower one is the minor groove. Proteins use the chemical groups that project from the bases into the grooves to recognize the sequence of double-stranded DNA. RNA Differences between DNA and RNA chemical structure: 1. The sugar in RNA is ribose instead of deoxyribose. The presence of a hydroxyl group at the 2' position of ribose makes the phosphodiester bond of RNA sensitive to base (OH–). Unlike DNA, RNA is degraded into mononucleotides in basic solution. 2. RNA contains no thymine but has uracil instead. These bases are very similar, differing only by a methyl group. Thus, uracil can form a Watson-Crick base pair with adenine, just as thymine can. 3. RNA is much shorter than DNA (usually no longer than a few thousand nucleotides, though some RNAs have been found that are over 50 kilobases in length). 4. RNA is synthesized as a single-stranded molecule. When not base-paired with another molecule, the backbone of an RNA strand folds into configurations that allow the strand to base pair with itself (Fig 22.2). Often different sections of one RNA strand interact with each other to form a right-handed, antiparallel double helix, but other structures can also arise, and base-pairing frequently deviates from the Watson-Crick scheme (Fig 21.30). The three-dimensional structures of RNA molecules can be quite complex. Like protein structure, RNA structure is sequence-dependent; this is less true for DNA, in which any sequence forms the same essential structure. 5. The cell is much more likely to chemically modify nitrogenous bases in RNA than in DNA (Fig 21.29). Over 100 different types of modified RNA bases have been identified, making up 1-2% of bases in tRNA and about 0.5% of bases in rRNA. These modified bases can influence the secondary and tertiary structure of RNA. Hybridization of nucleic acids Although we often think of double-stranded DNA in the context of an organism’s genome, any two nucleic acid strands (DNA or RNA, though for simplicity I’ll discuss only DNA in this section) will associate to form a helical structure (or hybridize) in solution if they are complementary enough. This process is reversible, such that DNA strands can be separated (denatured or melted) and brought back together (renatured) by changing the conditions. Denaturation of DNA is often brought about by heating the sample. The melting temperature (Tm) is the temperature at which 50% of a specific double-stranded DNA has become single-stranded. What determines the Tm? Intrinsic factors 1. A:T / G:C ratio. The more GC content, the higher the Tm. GC base pairs are more stable mainly because of increased base-stacking interactions, but also because they have three hydrogen bonds (versus two in AT base pairs) 2. Length. Shorter double helices melt at lower temperatures. 3. Degree of complementarity. Imperfect matches have lower Tm than perfect matches. Extrinsic factors 1. Salt concentration. Because counter-ions neutralize the negative charge of the phosphate backbone and reduce repulsion between strands, increasing the salt concentration raises the Tm. 2. Organic solvent concentration. Compounds such as dimethylformamide or isopropanol increase the hydrophobicity of the solvent and reduce base stacking interactions, lowering the Tm. 3. Hydrogen-bonding compounds. Formamide and urea decrease Tm by weakening hydrogen bonding between the bases. 4. pH. Extremes of pH change the protonation states of the bases, reducing the Tm. pH changes within the range of 5 to 9 do not alter Tm. For fragments of up to about 13 base pairs, the approximate melting temperature is the sum of 2°C for each A:T plus 4°C for each G:C base pair. More complicated equations exist to estimate the Tm of longer sequences, considering both sequence and environmental conditions. Genome packaging (Chapter 20.5 – Eukaryotic DNA is packaged in nucleosomes) The DNA of one human cell would be very long if the double helices were extended in a straight line. The cell must arrange the genome in a compact form so that it will fit within the nucleus. At the same time, the DNA must be available for transcription into RNA. This implies a high degree of organization in DNA packing. DNA is organized with the help of proteins; the complex of DNA and its organizing proteins is called chromatin. In eukaryotes, DNA is associated with small proteins called histones, each of which has fewer than 200 amino acids. Because histones have many lysine and arginine residues, they are positively charged at physiological pH and ideally suited to interact with the negatively charged phosphate groups of DNA. Histones are highly conserved among eukaryotes, reflecting their important function. Bacteria organize their genomes differently than eukaryotes, and we will not discuss bacterial genome packaging in this course. The DNA double-helix helix wraps around a cluster of eight histone proteins: two copies each of histone H2A, histone H2B, histone H3 and histone H4 (Figure 20.31). The DNA makes 1.7 circuits around the histone core, a distance of 147 base pairs. The wrapped DNA and histone core proteins are collectively called a nucleosome core particle. Regions of histones near the N-terminal ends are accessible for post-translational modification while in the nucleosome core particle; the importance of these modifications will be discussed in Topic 19. A linker region, ranging in length from a few bases to about 80 bases, joins the core particles. The nucleosome core particle plus the linker is properly called the nucleosome, although the word nucleosome is often used to refer to the nucleosome core particle only. Nucleosomes compact the DNA strand by a factor of about three. The nucleosomes can pack into a coil called a 30-nm chromatin fibre (Figure 20.33). Exactly how nucleosomes are arranged in this fibre is still controversial. The 30-nm fibre is stabilized by histone H1. In a 30-nm chromatin fibre, the length of the DNA has been compacted by a factor of about 100. It is thought that the 30-nm chromatin fibre forms large loops of 30 000 to 200 000 base pairs (30-200 kbp) that are anchored to the chromosomal scaffold, a group of chromatin proteins that do not belong to the histone family. The loops are packed together to form the chromosome, which is about 1400 nm thick in its fully condensed form, just before cell division (Fig 3.1). Details are still being discovered about how the cell is able to access individual sections of DNA when needed, despite all this packing. Controlling the density and positioning of nucleosome core particles is crucial for proper regulation of transcription, as will be discussed in Topic 19. Topic 14 Review Questions WileyPLUS questions for Chapter 3 – 5, 7, 23, 25, 29, 33, 37 WileyPLUS question for Chapter 20 – 77 14-1. Which statement is false? a) Nucleosomes are present in eukaryotic chromosomes, but not in bacterial chromosomes. b) Each nucleosome contains two molecules each of histone H2A, H2B, H3, and H4. c) A nucleosome core particle contains a core of histone with DNA wrapped around it approximately 1.7 times. d) Nucleosomes are aided in their formation by the high proportion of acidic amino acids in histone proteins. e) Nucleosome formation compacts the DNA into approximately one-third of its original length. Topic 14 Review Question Answers Note: Answers to textbook review questions are in your textbook 14-1. D