Hard Gelatin Capsules PDF
Document Details
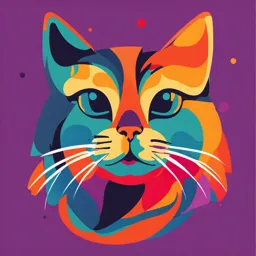
Uploaded by WellWishersProtagonist
Kenyatta University
Erick Kithinji Nega
Tags
Summary
This document provides an overview of hard gelatin capsules, covering topics such as raw materials (like gelatin and colourants), manufacturing processes, formulation techniques for filling, and release of active ingredients within these capsules. It is a valuable resource for understanding the pharmaceutical aspects of this dosage form.
Full Transcript
PPA 510: PHARMACEUTICS V PPA 512: ORAL SOLID DOSAGE FORMS HARD GELATIN CAPSULES BY DR. ERICK KITHINJI NJEGA OUTLINE Introduction Raw materials – gelatin, colourants, processing aids Manufacture Empty capsule properties Capsule filling, Capsule-filling machines, Filling of...
PPA 510: PHARMACEUTICS V PPA 512: ORAL SOLID DOSAGE FORMS HARD GELATIN CAPSULES BY DR. ERICK KITHINJI NJEGA OUTLINE Introduction Raw materials – gelatin, colourants, processing aids Manufacture Empty capsule properties Capsule filling, Capsule-filling machines, Filling of powder formulations, Pellet filling, Tablet filling, Semi-solid and liquid filling. Formulation Powder formulation, Formulation for filling properties, Formulation for release of active ingredients, Formulation optimization, Formulation or position of release INTRODUCTION The word ‘capsule’ is derived from the Latin capsula, meaning a small box. In current English usage it is applied to many different objects, ranging from flowers to spacecraft. In pharmacy, the word is used to describe an edible package made from gelatin or other suitable material which is filled with medicines to produce a unit dosage, mainly for oral use. There are two types of capsule, ‘hard’ and ‘soft’; better adjectives would be ‘two-piece’ in place of ‘hard’ and ‘one-piece’ in place of ‘soft’. INTRODUCTION The hard capsule consists of two pieces in the form of cylinders closed at one end; the shorter piece, called the ‘cap’, fits over the open end of the longer piece, called the ‘body’ RAW MATERIALS Similar raw materials have been used in the manufacture of both types of capsules. Traditionally both contain gelatin, water, colourants and optional materials, such as process aids and preservatives; In addition, soft capsules contain various plasticizers, such as glycerin and sorbitol. The major pharmacopoeias (European, Japanese and US) permit the use of gelatin or other suitable material. Since late 1990s hard capsules have also been manufactured from Hypromellose (hydroxypropy methylcellulose) in order to produce a shell with low moisture content. RAW MATERIALS - GELATIN Gelatin is still the major component used for capsules, and replacement polymer systems need to have the same basic properties. Gelatin possesses five basic properties that make it suitable for the manufacture of capsules: 1. It is non-toxic, widely used in foodstuffs, and is acceptable for use worldwide. 2. It is readily soluble in biological fluids at body temperature. 3. It is a good film-forming material, producing a strong flexible film. The wall thickness of a hard gelatin capsule is about 100 µm. RAW MATERIALS - GELATIN 4. Solutions of high concentration, e.g. 40% w/v, are mobile at 50 °C. Other biological polymers, such as agar, are not. 5. A solution in water undergoes a reversible change from a sol to a gel at temperatures only a few degrees above ambient. This is in contrast to other films formed on dosage forms, where either volatile solvents or large quantities of heat are required to cause this change of state, e.g. tablet film coating. These types of film are formed by spraying and have a structure that could be described as formed of overlapping plates, whereas the gelatin films are homogeneous in structure, which gives them their strength. RAW MATERIALS - GELATIN Gelatin is a substance of natural origin that does not occur as such in nature. It is prepared by the hydrolysis of collagen, which is the main protein constituent of connective tissues. Animal skins and bones are the raw materials used for the manufacture. There are two main types of gelatin: Type A, which is produced by acid hydrolysis, and Type B, which is produced by basic hydrolysis. The acid process takes about 7–10 days and is used mainly for porcine skins, because they require less pre-treatment than bones. RAW MATERIALS - GELATIN The basic process takes about 10 times as long and is used mainly for bovine bones. The bones must first be decalcified by washing in acid to give a soft sponge-like material, called ossein, and calcium phosphates are produced as a by-product. The ossein is then soaked in lime pits for several weeks. After hydrolysis, the gelatin is extracted from the treated material using hot water. The first extracts contain the gelatin with the highest physical properties, and as the temperature is raised, the quality falls. RAW MATERIALS - GELATIN The resulting weak solution of gelatin is concentrated in a series of evaporators and then chilled to form a gel. This gel is then extruded to form strands, which are then dried in a fluidized-bed system. The dried material is graded and then blended to meet the various specifications required. The properties of gelatin that are most important to capsule manufacturers are the Bloom strength and viscosity. The Bloom strength is a measure of gel rigidity. It is determined by preparing a standard gel (6.66% w/ v) and maturing it at 10 °C. RAW MATERIALS - GELATIN Bloom strength is defined as the load in grams required to push a standard plunger 4 mm into the gel. The gelatin used in hard capsule manufacture is of a higher Bloom strength (200–250 g) than that used for soft capsules (150 g) because a more rigid film is required for the manufacturing process. Many materials used in the manufacture of pharmaceuticals are manufactured from raw materials of bovine origin, e.g. stearates and gelatin. The outbreak of Bovine Spongiform Encephalopathy (BSE), which started in the UK, has led to strict rules being introduced by the EU to minimize the risks posed by animal Transmissible Spongiform Encephalopathy (TSE) agents. RAW MATERIALS - GELATIN The European Pharmacopoeia has included a general chapter on minimizing the risk of transmitting TSE agents via medicinal products and guidance on products at risk. Manufacturers of relevant materials have to submit data to the European Department of the Quality of Medicines (EDQM) who will review it and issue a certificate of suitability for their product. This must then be submitted to the national regulatory authorities for medicinal products that contain these materials. The Scientific Steering Committee of the EU has collected data from many countries and has assigned to each a Geographical BSE Risk (GBR). RAW MATERIALS - GELATIN There are four categories ranging from GBR I, which is a country in which BSE has never been detected and has in place a surveillance programme, e.g. New Zealand, to GBR IV which is a country in which the disease is prevalent, e.g. as was the case in the UK. Currently, gelatin for use in pharmaceuticals in the EU is sourced from bovine bones obtained from GBR I countries. RAW MATERIALS - COLOURANTS The colourants that can be used are of two types: 1. water-soluble dyes. 2. Insoluble pigments. To make a range of colours; dyes and pigments are mixed together as solutions or suspensions. The dyes used are mostly synthetic in origin and can be subdivided into; azo dyes (those that have an –N=N– linkage) non-azo dyes, which come from a variety of chemical classes. Most dyes used currently are non-azo and the three most widely used are; a. Erythrosine(E127), b. Indigo carmine (E132) and c. Quinoline yellow (E104). RAW MATERIALS - COLOURANTS Two types of pigment are used: a. Black, Red and Yellow iron oxides (E172) and b. Titanium dioxide (E171), which is white and is used to make the capsule opaque. The colourants that can be used to colour medicines are governed by legislation, which varies from country to country despite the fact that it is based on toxicological testing. In the last 20 years there has been a move away from soluble dyes to pigments, particularly the iron oxides, because they are not absorbed on ingestion. RAW MATERIALS - PROCESS AIDS The USNF describes the use of gelatin containing not more than 0.15% w/w of sodium lauryl sulfate for use in hard gelatin capsule manufacture. This functions as a wetting agent, to ensure that the lubricated metal moulds are uniformly covered when dipped into the gelatin solution. Preservatives were formerly added to hard capsules as an in-process aid in order to prevent microbiological contamination during manufacture. Manufacturers operating their plants to GMP guidelines no longer use them. In the finished capsules, the moisture levels, 13–16% w/ v, are such that the water activity will not support bacterial growth because the moisture is too strongly bound to the gelatin molecule. MANUFACTURE The process in use today is the same as that described in the original patent of 1846. Metal moulds at room temperature are dipped into a hot gelatin solution which gels to form a film. This is dried, cut to length, removed from the moulds and the two parts are joined together. The difference today is that the operation is now fully automated, carried out as a continuous process on large machines housed in airconditioned buildings. There is a limited number of specialist companies that manufacture empty capsule shells for supply to the pharmaceutical and health food industries, who fill them with their own products. MANUFACTURE The first step in the process is the preparation of the raw materials. A concentrated solution of gelatin, 35–40%, is prepared using demineralized hot water, 60–70 °C, in jacketed pressure vessels. This is stirred until the gelatin has dissolved and then a vacuum is applied to remove any entrapped air bubbles. Aliquots of this solution are dispensed into suitable containers and the required amounts of dye solutions and pigment suspensions added. The viscosity is measured and adjusted to a target value by the addition of hot water. MANUFACTURE This latter parameter is used to control the thickness of capsule shells during production: the higher the viscosity, the thicker the shell wall produced. The prepared mixes are then transferred to a heated holding hopper on the manufacturing machine. Hypromellose solutions can be converted into a gelling system by the addition of a gelling agent, such as carrageenan, and a co-gelling agent, such as potassium chloride, and used to manufacture capsules on standard unmodified machines. MANUFACTURE The manufacturing machines are approximately 10 m long, 2 m wide and 3 m high. They consist of two parts, which are mirror images of each other: on one half the capsule cap is made and on the other the capsule body. The machines are also divided into two levels, an upper and a lower. The moulds, commonly referred to as ‘pins’, are made of stainless steel and are mounted in sets on metal strips, called ‘bars’. There are approximately 50 000 mould pins per machine. The machines are housed in large rooms where the humidity and temperature are closely controlled. MANUFACTURE Figure 1 - The sequence of two- piece hard gelatin capsule shell manufacture. MANUFACTURE The sequence of events in the manufacturing process is shown in Figure 1. At the front end of the machine is a hopper, called a ‘dip pan’ or ‘pot’. This holds a fixed quantity of gelatin at a constant temperature, between 45 and 55 °C. The level of solution is maintained automatically by a feed from the holding hopper. Capsules are formed by dipping sets of moulds, which are at room temperature, 22 °C, into this solution. A film is formed by gelling on the surface of each mould. MANUFACTURE The moulds are slowly withdrawn from the solution and then rotated during their transfer to the upper level of the machine, in order to form a film of uniform thickness. Groups of ‘pin bars’ are then passed through a series of drying kilns, in which large volumes of controlled humidity air are blown over them. When they reach the rear of the machine, the bars are transferred back to the lower level and pass through further drying kilns until they reach the front of the machine. MANUFACTURE Here, the dried films are removed from the moulds, cut to the correct length, the two parts joined together and the complete capsule delivered from the machine. The mould pins are then cleaned and lubricated for the start of the next cycle. Hypromellose solutions behave in a similar way to gelatin, except that the speed of gelling is slower and thus the machine output is reduced. The machines are normally operated on a 24-hour basis, 7 days per week, stopping only for maintenance. The output per machine is over 1 million capsules per day, depending upon the size: the smaller the capsule, the higher the output. MANUFACTURE The assembled capsules are not fully closed at this stage and are in a ‘prelocked’ position, which prevents them falling apart before they reach the filling machine. The capsules now pass through a series of sorting and checking processes, which can be either mechanical or electronic, to remove as many defective ones as possible. The quality levels are checked throughout the process using standard statistical sampling plans based on the Military Inspection Standards. MANUFACTURE If required, capsules can be printed at this stage. This is done using an offset gravure roll printing process using an edible ink based on shellac. The information printed is typically either the product name or strength, a company name or logo, or an identification code. The capsules are finally packed for shipment in moisture-proof liners, preferably heat-sealed aluminium foil bags, in cardboard cartons. In these containers they can be stored for long periods without deterioration in quality, provided they are not subjected to localized heating or sudden temperature changes that will affect their moisture content and dimensions. EMPTY CAPSULE PROPERTIES Empty gelatin capsules contain a significant amount of water that acts as a plasticizer for the film and is essential for their function. During industrial filling and packaging operations, they are subjected to mechanical handling and because the gelatin walls can flex, these forces can be absorbed without any adverse effect. The standard moisture content specification for hard gelatin capsules is between 13% and 16% w/w. This value can vary depending upon the conditions to which they are exposed: at low humidities they will lose moisture and become brittle, and at high humidities they will gain moisture and soften. EMPTY CAPSULE PROPERTIES The moisture content can be maintained within the correct specification by storing them in sealed containers at an even temperature. The standard moisture content for Hypromellose capsules is 3% to 6% and when they lose moisture they do not become brittle. Gelatin capsules are readily soluble in water at 37 °C. Their rate of dissolution decreases when the temperature falls below this. Below about 26 °C gelatin capsules are insoluble and simply absorb water, swell and distort. EMPTY CAPSULE PROPERTIES This is an important factor to take into account during disintegration and dissolution testing. Because of this, most pharmacopoeias have set a limit of 37±1 °C for the media for carrying out these tests. Capsules made from hypromellose have a different solubility profile, being soluble at temperatures as low as 10 °C CAPSULE FILLING – Capsule sizes Hard capsules are made in a range of sizes; the standard industrial ones in use today for human medicines range from size 0 to 4 (Table 1). To estimate the fill weight for a powder, the simplest way is to multiply the body volume by its tapped bulk density. Table 1 Capsule size and body fill volumes CAPSULE FILLING – Capsule sizes CAPSULE FILLING – Capsule sizes The fill weight for liquids is calculated by multiplying the specific gravity of the liquid by the capsule body volume multiplied by 0.9. To accommodate special needs some intermediate sizes are produced, termed ‘elongated sizes’, that typically have an extra 10% of fill volume compared to the standard sizes, e.g. for 500 mg doses of antibiotics, elongated size 0 capsules are commonly used. The shape of the capsule has remained virtually unchanged since its invention more than 160 years ago, except for the development of the self-locking capsule during the 1960s, when automatic filling and packaging machines were introduced. CAPSULE FILLING – Capsule sizes Filled capsules were subjected to vibration during this process, causing some to come apart and spill their contents. To overcome this, modern capsule shells have a series of indentations on the inside of the cap and on the external surface of the body which, when the capsule is closed after filling, form an interference fit sufficiently strong to hold them together during mechanical handling. The manufacturer of the empty shells can be identified from the indentations, which are specific to each one. CAPSULE FILLING – Capsule shell filling Hard capsules can be filled with a large variety of materials of different physicochemical properties. The limitations in the types of material that can be filled are shown in Table 2. Table 2 Limitations in properties of materials for filling into capsules Gelatin and hypromellose are relatively inert materials. CAPSULE FILLING – Capsule shell filling The substances to be avoided are; 1. Those which are known to react with gelatin, e.g. formaldehyde, which causes a crosslinking reaction that makes the capsule insoluble, 2. Those that interfere with the integrity of the shells, e.g. substances containing free water, which can be absorbed by the gelatin or hypromellose, causing them to soften and distort. 3. There is also a limitation on the size of capsule that can be easily swallowed, and thus large doses of low-density formulations cannot be used. CAPSULE FILLING – Capsule shell filling Table 3 - Types of material for filling into hard gelatin capsules CAPSULE FILLING – Capsule shell filling The materials that have been filled into hard capsules are given in Table 3. The reason why such a range of materials can be handled is the nature of the capsule- filling process. Empty hard capsules are supplied in bulk containers. First, it is necessary for the filling machine to orientate them so that they are all pointing in the same direction, i.e. body first. To do this, they are loaded into a hopper and from there fall randomly through tubes to a rectification section. Here the capsules are held in tight-fitting slots. CAPSULE FILLING – Capsule shell filling Metal fingers strike them in the middle and because the bodies have the smaller diameter, they rotate away from the direction of impact. Next the capsules are sucked into pairs of bushings that trap the caps in the upper one, because of their greater diameter, thus separating them from the bodies. The bodies are then passed under the dosing mechanism and filled with material. Thus, providing a substance can be measured and dosed, it can be filled into capsules. The caps are then repositioned over the bodies and metal fingers push the bodies up into them to rejoin the two parts. CAPSULE FILLING – Capsule-filling machines The same set of basic operations is carried out whether capsules are being filled on the bench for extemporaneous dispensing or on high- speed automatic machines for industrial products. The major difference between the many methods available is the way in which the dose of material is measured into the capsule body. CAPSULE FILLING – Filling of powder formulations - Bench-scale filling There is a requirement for filling small quantities of capsules, from 50 to 10 000, in hospital pharmacies or in industry for special prescriptions or trials. There are several simple pieces of equipment available, e.g. The ‘Feton’ from Belgium, that consists of sets of plastic plates with predrilled holes to take from 30 to 100 capsules of a specific size. Empty capsules are fed into the holes, either manually or with a simple loading device. The bodies are locked in their plate by means of a screw and the caps in their plate are removed. CAPSULE FILLING – Filling of powder formulations - Bench-scale filling Feton capsule filling machine CAPSULE FILLING – Filling of powder formulations - Bench-scale filling The bodies are released and drop below their plate surface, powder is placed onto this surface and is spread with a spatula so that it fills the bodies. The uniformity of fill weight is very dependent upon good flow properties of the powder. The cap plate is then repositioned over the body plate and the capsules are rejoined using manual pressure. Stainless steel versions of these devices are now available, e.g. Profill (Torpac Inc, USA), that can be cleaned and autoclaved to comply with GMP requirements. CAPSULE FILLING – Filling of powder formulations - Industrial-scale filling The machines for the industrial-scale filling of hard capsules come in great variety of shapes and sizes, varying from semi- to fully automatic and ranging in output from 3000 to 150 000 per hour. Automatic machines can be either; 1. Continuous in motion, like a rotary tablet press, or 2. Intermittent, where the machine stops to perform a function and then indexes round to the next position to repeat the operation on a further set of capsules. CAPSULE FILLING – Filling of powder formulations - Industrial-scale filling The dosing systems can be divided into two groups: 1. Dependent – dosing systems that use the capsule body directly to measure the powder. Uniformity of fill weight can only be achieved if the capsule is completely filled 2. Independent – dosing systems whereby the powder is measured independently of the body in a special measuring device. Weight uniformity is not dependent on filling the body completely. With this system capsules can be part filled. CAPSULE FILLING – Filling of powder formulations - Industrial-scale filling Figure 2 - An auger filling machine using the ring system. Model No. 8. CAPSULE FILLING – Filling of powder formulations - Industrial-scale filling Dependent dosing systems The auger. Empty capsules are fed into a pair of ring holders (Figure 2), the caps being retained in one half and the bodies in the other. The body holder is placed on a variable-speed revolving turntable; the powder hopper is pulled over the top of this plate, which revolves underneath it. In the hopper, a revolving auger forces powder down into the capsule bodies. The weight of powder filled into the body is dependent mainly upon the time the body is underneath the hopper during the revolution of the plate holder. CAPSULE FILLING – Filling of powder formulations - Industrial-scale filling Dependent dosing systems The auger. These machines are semi-automatic, requiring an operator to transfer the capsule holders from one position to the next. They were first developed for large-scale use during the first half of the 20th century and are still widely used in many countries. The contact parts of these machines were originally made from cast iron but are now made from stainless steel to comply with GMP requirements. Their output varies between 15 000 and 25 000 per hour, which is dependent upon the skill of the operator, and they are widely used by the herbal and nutraceutical industries. CAPSULE FILLING – Filling of powder formulations - Industrial-scale filling Figure 3 - A dosing tube or dosator- type machine, Zanasi RM63. CAPSULE FILLING – Filling of powder formulations - Industrial-scale filling Independent dosing systems Most industrial machines in Europe and the USA are fully automatic and use dosing mechanisms that form a ‘plug’ of powder. This is a soft compact formed at low compression forces, between 10 and 100 N, which are significantly less than those used in tableting (10–100 kN). The reason the plug is soft is because it is not the final dosage form, unlike the tablet, as the material will be contained inside a capsule shell. There are two types of plug-forming machine: 1. The ‘dosator’ system and 2. The ‘tamping finger and dosing disc’ system. CAPSULE FILLING – Filling of powder formulations - Industrial-scale filling Dosator. This consists of a dosing tube inside which there is a movable spring- loaded piston, thus forming a variable-volume chamber in the bottom of the tube (Figure 3). This is lowered open end first into a bed of powder, which enters the tube to fill the chamber and form a plug. This can be further consolidated by applying a compression force with the piston. The assembly is then raised from the powder bed and positioned over the capsule body. CAPSULE FILLING – Filling of powder formulations - Industrial-scale filling Dosator. The piston is lowered, ejecting the powder plug into the capsule body. The weight of powder filled can be adjusted by altering the position of the piston inside the tube, i.e. increasing or decreasing the volume, and by changing the depth of the powder bed. This system is the most widely used and the one most described in the literature. Examples of machines that use this system are: a. intermittent motion – Zanasi (IMA), Pedini, Macophar and Bonapace. Their outputs range from 3000 to 60 000 per hour b. Continuous motion – MG2, Imatic (IMA). Their outputs range from 30 000 to 150 000 per hour. CAPSULE FILLING – Filling of powder formulations - Industrial-scale filling Figure 4 - A dosing disc and tamping finger machine. CAPSULE FILLING – Filling of powder formulations - Industrial-scale filling Tamping finger and dosing disc. The dosing disc forms the bottom of a revolving powder hopper (Figure 4). This disc has several sets of accurately drilled holes in which powder plugs are formed by several sets of tamping fingers – stainless steel rods that are lowered into them through the bed of powder. At each position the fingers compress the material in the holes, building up a plug before they index on to the next position. CAPSULE FILLING – Filling of powder formulations - Industrial-scale filling Tamping finger and dosing disc. As the disc rotates, material flows into the holes. At the last position, fingers push the plugs through the disc into capsule bodies. The powder fill weight can be varied by the amount of insertion of the fingers into the disc, by changing the thickness of the dosing disc, and by adjusting the amount of powder in the hopper. The machines that use this system are all intermittent in motion. Examples are manufactured by Robert Bosch, Harro Höfliger, PAM machinery and Qualicaps. CAPSULE FILLING – Pellet filling Preparations formulated to give modified-release patterns are often produced as granules or coated pellets. They are filled on an industrial scale using machines adapted from powder use. All have a dosing system based on a chamber with a volume that can easily be changed. Pellets are not compressed in the process and may have to be held inside the measuring devices by mechanical means, e.g. by applying suction to the dosing tube. CAPSULE FILLING – Pellet filling In calculating the weight of particles that can be filled into a capsule, it is necessary to make an allowance for their size. Unlike powders, which have a much smaller size, they cannot fill as much of the available space within the capsule because of packing restrictions. The degree of this effect will be greater the smaller the capsule size and the larger the particle diameter. CAPSULE FILLING – Tablet filling Tablets are placed in hoppers and allowed to fall down tubes, at the bottom of which is a gate device that will allow a set number of tablets to pass. These fall by gravity into the capsule bodies as they pass underneath the hopper. Most machines have a mechanical probe that is inserted into the capsule to check that the correct number of tablets has been transferred. Tablets for capsule filling are normally film coated to prevent dust generation, and are sized so that they can fall freely into the capsule body but without turning on their sides. CAPSULE FILLING – Tablet filling A recent innovation is the filling of coated minitablets, which have a significantly smaller surface area than the equivalent quantity of pellets, thus, reducing the amount of coating required and improving its uniformity. CAPSULE FILLING – Semi-solid and liquid filling Liquids can easily be dosed into capsules using volumetric pumps (Rowley 2004). The problem after filling is to stop leakage from the closed capsule. This can be done in one of two ways; By formulation or By sealing the capsule. Semi-solid mixtures are formulations that are solid at ambient temperatures and can be liquefied for filling by either heating (thermosoftening mixtures) or by stirring (thixotropic mixtures). After filling, they cool and solidify or revert to their resting state in the capsule to form a solid plug. CAPSULE FILLING – Semi-solid and liquid filling Both types of formulations are filled as liquids using volumetric pumps. These formulations are similar to those that are filled into soft gelatin capsules but differ in one important respect: they can have melting points higher than 35°C, which is the maximum for soft capsules because this is the temperature used by the sealing rollers during their manufacture. Nonaqueous liquids, which are mobile at ambient temperatures, require the capsules to be sealed after filling. The industrially accepted method for this is to seal the cap and body together by applying a gelatin solution around the centre of the capsule after it has been filled. CAPSULE FILLING – Semi-solid and liquid filling When this has been dried it forms a hermetic seal that; prevents liquid leakage, contains odors inside the shell and significantly reduces oxygen permeation into the contents, protecting them from oxidation. An example of such equipment is the Qualicaps Hicapseal machine, which has outputs ranging from 40 000 to 100 000 per hour. CAPSULE FILLING – FORMULATION All formulations for filling into capsules have to meet the same basic requirements: 1. They must be capable of being filled uniformly to give a stable product. 2. They must release their active contents in a form that is available for absorption by the patient. 3. They must comply with the requirements of the Pharmacopoeial and regulatory authorities, e.g. dissolution tests. CAPSULE FILLING – FORMULATION In order to formulate rationally, it is necessary to take into account the mechanics of the filling machines and how each type of product is handled. CAPSULE FILLING – Powder formulation The majority of products for filling into capsules are formulated as powders. These are typically mixtures of the active pharmaceutical ingredient together with a combination of different types of excipients (Table 4). Table 4 - Types of excipient used in powder- filled capsules CAPSULE FILLING – Powder formulation The ones selected depend upon several factors: the properties of the active drug: its dose, solubility, particle size and shape the filling machine to be used the size of capsule to be used. The latter factor defines the free space inside the capsule that is available to the formulator. The easier active compounds to formulate are low-dose potent ones, which in the final formulation occupy only a small percentage of the total volume (< 20%) and so the properties of the mixture will be governed by the excipients chosen. CAPSULE FILLING – Powder formulation Those compounds with a high unit dose, e.g. 500 mg of an antibiotic, leave little free space within the capsule and the excipients chosen must exert their effect at low concentrations (< 5%) and the properties of the mixture will be governed by that of the active ingredient. FORMULATION FOR FILLING PROPERTIES There are three main factors in powder formulation: 1. Good flow (using a free- flowing diluent and glidant) 2. No adhesion (using a lubricant) 3. Cohesion (plug-forming diluent). The factor that contributes most to the uniform filling of capsules is good powder flow, because all machines operate by measuring volumes of powders, and thus, the formulator’s objective is to make powders behave like liquids. FORMULATION FOR FILLING PROPERTIES The powder bed, from which the dose is measured, needs to be homogeneous and packed reproducibly in order to achieve uniform fill weights. Packing is assisted by mechanical devices or suction pads on the filling machines. Low-dose actives can be made to flow well by mixing them with free- flowing diluents, e.g. lactose. The diluent is also chosen for its plug-forming properties: those most frequently used are lactose, starch 1500 and microcrystalline cellulose. FORMULATION FOR FILLING PROPERTIES When space is limited then either glidants, which are materials that reduce inter-particulate friction, such as colloidal silicon dioxide, or lubricants, which are materials that reduce powder-to-metal adhesion, e.g. magnesium stearate, are added, enabling the dosing devices to function efficiently. Glidants exert their effect by coating the surfaces of the other ingredients and thus the mixing of them into the bulk powder has a significant effect on their functioning. FORMULATION FOR RELEASE OF ACTIVE INGREDIENTS The first stage in release from capsules is disintegration of the capsule shell. When gelatin capsules are placed in a suitable liquid at body temperature, 37 °C, they start to dissolve and within 1 minute the shell will split, usually at the ends. With a properly formulated product, the contents will start to empty out before all the gelatin has dissolved. The official tests for disintegration and dissolution were originally designed for tablets. FORMULATION FOR RELEASE OF ACTIVE INGREDIENTS Capsules have very different physical properties and after the contents have emptied, the gelatin pieces remaining will adhere strongly to metal surfaces and may confuse the endpoint of the test. Hypromellose capsules take longer for the time to the first split but after this tend to disperse faster than gelatin capsules. The literature shows that the rate-controlling step in capsule disintegration and product release is the formulation of the contents, which ideally should be hydrophilic and dispersible. The factors that can be modified to make the active ingredients readily available depend upon their properties and those of any excipients being used. FORMULATION FOR RELEASE OF ACTIVE INGREDIENTS The active ingredients have a fixed set of physicochemical properties which, except for the particle size, are out of the control of the formulator. FORMULATION FOR RELEASE OF ACTIVE INGREDIENTS It has been shown that the particle size influences the rate of absorption for several compounds formulated into capsules. For sulfisoxazole (Figure 5), three different particle sizes were filled into capsules and administered to dogs; the smallest particle gave the highest peak blood level. Figure 5 - Effect of particle size on bioavailability of sulfisoxazole FORMULATION FOR RELEASE OF ACTIVE INGREDIENTS This can be explained simply by the fact that the dissolution rate is directly proportional to the surface area of the particles: the smaller the particle, the greater the relative surface area. However, this is not a panacea for formulation problems because small particles tend to aggregate and the effect is lost. It has been shown that the important factor with particle size is the ‘effective surface area’, which is the area of the active available to the dissolution fluid. This is related to the packing of particles and is a measure of how well the fluid can penetrate into the mass. FORMULATION FOR RELEASE OF ACTIVE INGREDIENTS Diluents are the excipients that are usually present in the greatest concentration in a formulation. They were classically defined as inert materials added to a mixture to increase its bulk to a more manageable quantity. Although they are relatively inert chemically, they do play a role in release. The case that first demonstrated this occurred in Australia in the late 1960s. A capsule was reformulated that contained phenytoin (diphenylhydantoin), which is used for the treatment of epilepsy and is taken chronically. FORMULATION FOR RELEASE OF ACTIVE INGREDIENTS The diluent used was changed from calcium sulfate to lactose. In the months following this change, there was an upsurge in reports of side-effects similar to overdosing of product. It was demonstrated that the change had had a significant effect on the bioavailability of the active (Figure 6). The change to lactose gave much higher blood levels of the drug, which was probably due to the fact that it is readily soluble whereas calcium sulfate is not. FORMULATION FOR RELEASE OF ACTIVE INGREDIENTS Figure 6 - Effect of diluent on bioavailability of phenytoin FORMULATION FOR RELEASE OF ACTIVE INGREDIENTS Since this occurrence, the phenomenon has been shown to occur with other actives. The diluent used should be chosen in relation to the solubility of the active. If a soluble diluent such as lactose is added to a poorly or insoluble compound, it will make the powder mass more hydrophilic, enabling it to break up more readily on capsule shell disintegration. The converse is also true: actives that are readily soluble are best mixed with insoluble diluents such as starch or microcrystalline cellulose, because they help the powder mass to break up without interfering with their solubility in the medium. FORMULATION FOR RELEASE OF ACTIVE INGREDIENTS Some excipients, such as lubricants and glidants, are added to formulations to improve their filling properties and these can sometimes have an effect on release. The important thing to avoid in formulations is materials that tend to make the mass more hydrophobic. The most commonly used lubricant for both encapsulation and tableting is magnesium stearate. Simmons et al (1972) studied the dissolution rate of chlordiazepoxide capsule formulations with three levels of magnesium stearate: 0%, 1% and 5% (Figure 7). FORMULATION FOR RELEASE OF ACTIVE INGREDIENTS Figure 7 - Effect of lubricant on release of chlordiazepoxide FORMULATION FOR RELEASE OF ACTIVE INGREDIENTS They found that the dissolution rate was greatly reduced at the highest level of magnesium stearate, which they explained was due to the poor wetting of the powder mass. However, hydrophobic additives are not always deleterious because they reduce the cohesiveness of the powder mass. This was first demonstrated by Nakagawa et al (1980), who were studying the dissolution of different particle sizes of rifampicin with and without magnesium stearate (Figure 8). FORMULATION FOR RELEASE OF ACTIVE INGREDIENTS Figure 8 - Effect of lubricant (magnesium stearate, MS) on in vitro release of rifampicin (after Nakagawa et al 1980). FORMULATION FOR RELEASE OF ACTIVE INGREDIENTS They found that, for the larger particles (180–355 µm), the addition of magnesium stearate reduced the rate, whilst for the smaller particles (