Organic Pharmaceutical Chemistry Lecture 4 PDF
Document Details
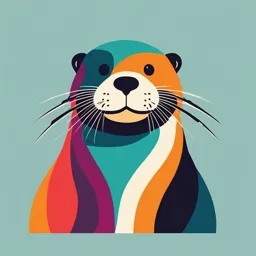
Uploaded by ExuberantVignette2712
University of Al-Ameed College of Medicine
Dr. Abbas Abdulridha
Tags
Related
- Organic Pharmaceutical Chemistry Lecture 4 - Drug Metabolism (PDF)
- Organic Pharmaceutical Chemistry Lec 4 - Drug Metabolism PDF
- Organic Pharmaceutical Chemistry Lecture 4 - Drug Metabolism PDF
- Organic Pharmaceutical Chemistry Lecture 5 - Phase I Reactions PDF
- Organic Pharmaceutical Chemistry - Third Stage - PDF
- Drug Design Strategies PDF
Summary
This is a lecture covering organic pharmaceutical chemistry, specifically focusing on bioprecursor prodrugs and their activation mechanisms. It details various activation methods including oxidation, reduction, and chemical activation, providing substantial chemical details and explanations. The lecture is likely for a postgraduate-level course at a pharmaceutical college.
Full Transcript
University of Al-Ameed College of Pharmacy Organic Pharmaceutical Chemistry lecture 4 5th class/ 1st semester Bioprecursor prodrugs Dr. Abbas Abdulridha 2. Bioprecursor prodrugs It does not has a carrier or promoiety, but it contains a functionalit...
University of Al-Ameed College of Pharmacy Organic Pharmaceutical Chemistry lecture 4 5th class/ 1st semester Bioprecursor prodrugs Dr. Abbas Abdulridha 2. Bioprecursor prodrugs It does not has a carrier or promoiety, but it contains a functionality that can converted to the active molecule. Activation could be through oxidation, reduction, phosphorylation, and chemical activation, …. Oxidation is the most common type of activation owing to the abundant of endogenous oxidizing enzymes. Phosphorylation is widely used in the antiviral compounds and many drugs use this type of activation. Example of prodrugs require oxidative activations is Nabumetone 2.1. Proton transfer A good example of chemical activation is seen with the proton pump inhibitors such as omeprazole. Omeprazole as pro-‐drug is converted to its active form in acidic medium. As weak base it specially concentrates in the acidic secretory canaliculi of the parietal cell, where it is activated by a proton-‐catalyzed process to generate a sulfenamide intermediate. The sulfenamide interacts covalently with sulphydryl groups of cysteine residues in the extracellular domain of the proton pump (H+/K+-‐ATPase) there by inhibiting its activity. ✓ The pKa of the pyridine ring of omeprazole is about 4, so it is not protonated and able to cross the secretory canaliculus of the parietal cell. ✓ The pH inside the cell is below 1, so this initiates the protonation reaction below. In this case, chemical activation is provided by the highly acidic environment in and around the parietal cell of the stomach 1. This allows protonation of nitrogen on the benzimidazole ring 2. followed by attachment of the pyridine nitrogen. 3. Ring opening then gives the sulfenic acid that subsequently cyclize with the loss of water 4. Attachment by a sulfhydryl group present on the proton pump of the parietal cell occurs and inactivates this enzyme, then preventing further release of H+ into the GI tract, which is useful in treating gastric ulceration. 12 2.2. Oxidative activation Gastric irritations are produced by NSAIDs is a serious concern, particularly in patients using such medicines for a long period or have a pre-existing conditions. The irritation is partly associated with an acidic functional groups in this class of drugs. The carboxylic groups is unionized in the acidic pH of stomach and increase lipophilicity, which allows the molecule to pass gastric mucous cells ✓ The intracellular pH of these cells is more basic than that of the stomach lumen, and the NSAID becomes ionized. ✓ This results in backflow of H+ from the lumen into these cells, with concomitant cellular damage. ✓ This type of damage could be prevented if the carboxylic acid function could be eliminated from these agents. ✓ However this functional group is required for activity. Nabumetone The NSAID Nabumetone (Relafen) is good example of a prodrug that requires oxidative activation. Nabumetone contains no acidic functionality and passes through the stomach without producing the irritation normally associated with this class of agents. Subsequent absorption occurs in the intestine, and metabolism in the liver produces the active compound. 2.3. Reductive activation Prodrugs require reduction to be activated are less common because of the fewer reducing enzymes in vivo. Anticancer prodrugs designed to target specifically tumour cells should 1. increase therapeutic effectiveness and 2. decrease systemic side effects in the treatment of cancer. Reductively activated prodrugs have been designed to target hypoxic tumour tissues, which are known to overexpress several endogenous reductive enzymes. In addition, exogenous reductive enzymes can be delivered to tumour cells through fusion with tumour-specific antibodies or overexpressed in tumour cells through gene delivery approaches. Many anticancer prodrugs have been designed to use both the endogenous and exogenous reductive enzymes for target- specific activation and these prodrugs often contain functional groups such as quinones, nitroaromatics, N-oxides, and metal complexes. eg. Mitomycin C it has modest selectivity for the hypoxic tissues; indicated in bladder and lung cancer. Mitomycin C contains a quinone functionality that undergoes reduction to give a hydroquinone. This is important because of the differential effect of the quinone and hydroquinone on the electron pair of the nitrogen. Whereas the quinone has an electron-withdrawing effect on this electron pair, the hydroquinone has an electron-releasing effect, which allows these electrons to participate in the expulsion of methoxide and the subsequent loss of the carbamate to generate a reactive species that can alkylate DNA. The cascade of events that leads to an alkylating active drug species is initiated by the reduction of the quinone functionality in mitomycin C. The selectivity of mitomycin for hypoxic cells is minimal, however. ✓ The selectivity is determined in part by the reduction potential of the quinone, which can be influenced by the substituents attached to the ring. ✓ In an effort to modify the reduction potential of mitomycin C, various analogues have been prepared and tested for antineoplastic activity. It was hoped that the reduction potential could be altered so that the analogues would only be activated in hypoxic conditions, such as those found in slow-growing solid tumors that are poorly vascularized. ✓ Although mitomycin was the first agent used clinically to be recognized as requiring reductive activation, it is only modestly selective for hypoxic cells. 10 Tirapazamine is much more selective agent. It is reported to be 100 to 200 times more selective for hypoxic cells than for normal cells. ✓ The mechanism of activation involves a one-electron reduction that is catalysed by a number of enzymes, including cytochrome P-450 and cytochrome P-450 reductase to give a radical species. This species, which is shown as a carbon-centered radical, can initiate breaks in the DNA chain under hypoxic conditions. 11 2.4. Oxidative Activation a. N-Dealkylation CH3 CH3 CH3 N N N N N N N N N CH3 P450 CH3 P450 NH.. 2 O N N O Cl O CH3 Cl H Cl X X X CH3 N CH3 N N N N -H2 O N Cl N.. Cl NH HO X X alprazoal am (X = H) triazolam (X = Cl ) 8.77 b. O-Dealkylation Analgesic activity of phenacetin is a result of O-dealkylation to acetaminophen. O HN CH3 OR phenacetin (R = CH2 CH3 ) acetam inoph en (R = H) 8.78 c. Oxidative Deamination Neoplastic (cancer) cells have a high concentration of phosphoramidases, so hundreds of phosphamide analogs of nitrogen mustards were made for selective activation in these cells. H HO: H O N P-450 N H NH O O 2 P Cl P Cl H P O O N O N O Cl N B: Cl Cl Cl cyclophosphami de 8.80 8.81 8.79 H2N O O -O P Cl Cl spont aneous N H HPO4= + NH3 + HN or + phos phoram id ase Cl Cl 8.83 8.84 8.82 DNA DNA O H O H + N N N OH N OH HN HN H 2N N N H2N N N DNA 8.86 8.85 Cyclophosphamide was very effective, but it required liver homogenates (contains P450) for activation. d. N-Oxidation Pralidoxime chloride is an antidote for nerve poisons. It reacts with acetylcholinesterase that has been inactivated by organophosphorus toxins. has a quaternary nitrogen → fully charged and cannot pass through the blood–brain barrier into the CNS. ✓antidote cannot work on any enzymes that have been inhibited in the brain. Pro-2-PAM It is a prodrug of pralidoxime which avoids this problem. As a tertiary amine it can pass through the blood–brain barrier and is oxidized to pralidoxime once it has entered the CNS. 16 e. S-Oxidation Poor oral bioavailability of brefeldin A. Converted to Michael addition sulfide prodrug (8.98). S-Oxidation and elimination gives brefeldin A. Scheme 8.27 :B H HO H HO H HO H H O O HO RSH [O] O O CH3 HO RS H HO RS O CH3 H O CH3 H H O H brefeldin A 8.97 8.98 8.99 -RSOH antitumor, antiviral agent 2.5. Aromatic Hydroxylation Cyclohexenones as prodrugs for catechols N -H2 O N N P450 N HO O O OH O P450 8.100 aromatic hydroxylation oxidation next to sp2 carbonyl N HO OH 8.101 2.6. Transamination Stimulation of pyruvate dehydrogenase results in a change of myocardial metabolism from fatty acid to glucose utilization. Glucose metabolism requires less O2 consumption. Therefore, utilization of glucose metabolism would be beneficial to patients with ischemic heart disease (arterial blood flow blocked; less O2 available). O Arylglyoxylic acids (8.104) COOH stimulate pyruvate dehydrogenase, but have a short duration of action. R 8.104 Oxfenicine (8.105) NH2 is actively transported and is transaminated by COOH aminotransferase in the heart to 8.104 (R = OH). R oxfenicine (R = OH) 8.105 2.7.Reductive Activation Azo Reduction COOH NHSO2 N=N OH Anaerobic cleavage by N bacteria in lower sulfasal azi ne 8.106 bowel COOH H2N OH + NHSO2 NH2 N 8.107 sulfapyri dine 8.108 For inflammatory bowel disease 2.7. Disulfide Reduction To increase the lipophilicity of thiamin for absorption into the CNS. OH OH.. N GSH N N S S O N S- O H O H CH3 N NH2 CH3 N NH2 +B H 8.113 nonenzymatic OH + N: N N OH N S S CH3 N NH2 CH3 N NH2 OH thiami n 8.114 poorly absorbed into CNS CH3O To diminish toxicity of primaquine and target it for cells with N the malaria parasite, a macromolecular drug delivery HN NH2 system was designed. prim aquine 8.115 antimalarial, toxic Intracellular thiol much higher than in blood; selective reduction inside the cell CH3O lacto se N O HN serum N S S H alb umin NH3+ primaquine 8.116 for improved lacto se uptake in liver The therapeutic index of 8.116 is 12 times higher than 8.115 in mice. 2.8. Nucleotide Activation =O SH 3PO O O O SH N N O—P—O—P—O- N N N N HO OH O- O- =O 3PO O N N hypoxanthine-guanine H phos phoribo syltrans feras e 6-m ercaptopurine HO OH 8.122 Anti-leukemia drug 8.123 Inhibits several enzymes in the purine nucleotide biosynthesis pathway. 2.9. adenosine deaminase acyclovir Only 15-20% of acyclovir is absorbed Therefore, prodrugs have been designed to increase oral absorption. Hydrolyzes here NH 2 N N Prodrug for a prodrug H 2N N N HO O 8.126 adenosine deaminase acyclovir 2.10. Phosphorylation Activation acyclovir resembles structure of 2-deoxyguanosine O O N HN N HN H 2N N N H2N N N HO RO O O HO acyclovir (R = H) 2-deoxyguanosine Acyclovir 8.124(R=H) 2-deoxyguanosine 8.125 viral thymidine kinase R = PO3= Uninfected cells do not viral guanylate kinase phosphorylate acyclovir (selective R = P2O6-3 toxicity) viral phosphoglycerate kinase R = P3O9-4 ✓ Acyclovir triphosphate is a substrate for viral -DNA polymerase but not for normal -DNA polymerase ✓ Incorporation into viral DNA leads to a dead-end complex (not active). Disrupts viral replication cycle and destroys the virus. ✓ Even if the triphosphate of acyclovir were released, it is too polar to be taken up by normal cells. High selective toxicity Resistance to Acyclovir Modification of thymidine kinase Change in substrate specificity for thymidine kinase Altered viral -DNA polymerase Hydroxylates here N N H 2N N N HO O 8.127 xanthine oxidase acyclovir This prodrug is 18 times more water soluble than acyclovir. 2.9. Decarboxylation Activation An imbalance in the inhibitory neurotransmitter dopamine and the excitatory neurotransmitter acetylcholine produces movement disorders, e.g. Parkinson’s disease. In Parkinson’s there is a loss of dopaminergic neurons and a low dopamine concentration. Dopamine treatment does not work because it cannot cross blood- brain barrier, but there is an active transport system for L-dopa (levodopa, R = COOH). HO HO NH2 H R levodopa (R = CO OH) 8.133 After crossing blood-brain L-aromatic amino acid barrier decarboxylase dopamine (R = H) Does not reverse the disease, only slows progression. Levodopa, utilizes a receptor on the BBB known as Large amino acid transporter (LAT) which allows it to be transported across the blood-brain barrier and decarboxylated into Dopamine. Combination Therapy Peripheral L-aromatic amino acid decarboxylase destroys >95% of the L-dopa in the first pass. Maybe only 1% actually gets into brain. To protect L-dopa from peripheral (but not CNS) degradation, inhibit peripheral L- aromatic amino acid decarboxylase with a charged molecule that does not cross the blood-brain barrier. HO HO OH HO O NH 2 NHNH3+ HO H3 C N N OH COO- H H carbi dopa benserazi de 8.134 8.135 (used in U.S.) (used in Europe and Canada) ✓ Selectively inhibits peripheral L-amino acid decarboxylase. ✓ The dose of L-dopa can be reduced by 75%. Bioprecursor prodrug (Sulindac) Comparing soft-drug vs co-drug vs carrier-linked Carrier-linked prodrug: drugs attached through a metabolically labile linkage to another molecule (promoiety), which is not necessary for the activity, but impart some desirable property of the drug. Co-drugs (Mutual prodrug): Prodrugs consist of two pharmacologically active drugs that are coupled, each one acts as a promoiety for the other. Soft drug: it is active drug designed to undergo a controllable deactivation or metabolism in vivo after achieving their therapeutic effect.