Neuroimaging Methods for Nursing Science PDF
Document Details
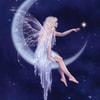
Uploaded by AppealingAmazonite
null
Tags
Summary
This document provides an overview of neuroimaging techniques and their applications in nursing science. It also details various methods like MRI, fMRI, CT, and PET, along with their strengths and limitations for medical research. The methods aid in understanding disease processes and developing effective interventions.
Full Transcript
Reading 13 January 2024 21:24 Source Notes Neuroimaging Methods for Nursing Science Introduction Neuroimaging involves various methods to image brain structure and function. Magnetic Resonance Imaging (MRI) has led to numerous studies, with nurse -led contributions. Despite technological accessibili...
Reading 13 January 2024 21:24 Source Notes Neuroimaging Methods for Nursing Science Introduction Neuroimaging involves various methods to image brain structure and function. Magnetic Resonance Imaging (MRI) has led to numerous studies, with nurse -led contributions. Despite technological accessibility, nurse scientists face challenges in developing neuroimaging research programs. (Atalla et al., 2020 ) Methods Review of Brain Imaging Methods ○ Structural MRI is crucial for clear visualization of neurological structures. ○ Fluid Attenuation Inversion Recovery (FLAIR) enhances sensitivity to pathological abnormalities. ○ Diffusion Tensor Imaging (DTI) measures water molecule diffusion along nerve fibres, providing insights into white matter integrity. ○ Arterial Spin Labelling (ASL) focuses on measuring tissue perfusion, especially cerebral blood flow. ○ Functional MRI (fMRI) measures changes in deoxygenated haemoglobin concentration, reflecting brain metabolic demand. ○ Task-Evoked fMRI studies brain activity in response to specific stimuli. ○ Resting-State fMRI explores brain activation in the absence of stimuli. ○ Computed Tomography (CT) uses X-rays to generate anatomical images. ○ Positron Emission Tomography (PET) enables in vivo imaging of biological processes. ○ Electroencephalography (EEG) and Magnetoencephalography (MEG) map brain activity by detecting ionic currents. Results Structural MRI ○ Shows neurological structures with clarity, useful for examining abnormal brain anatomy. ○ T1-weighted images provide good contrast between grey matter, white matter, and cerebrospinal fluid (CSF). ○ T2-weighted images contrast CSF and brain matter, useful for detecting pathological white matter lesions. Fluid Attenuation Inversion Recovery (FLAIR) ○ Highly sensitive to pathological abnormalities, suppresses CSF signal. ○ Useful for detecting cerebrovascular disease, metastatic disease, and haemorrhage. ○ Excellent for determining the acuteness of white matter disease. Diffusion Tensor Imaging (DTI) ○ Measures water molecule diffusion along nerve fibres, providing insights into white matter integrity. ○ Apparent diffusion coefficient (ADC) identifies areas of damage to white matter caused by ischemia or edema. ○ Sensitive, non-invasive method for studying white matter integrity. Arterial Spin Labelling (ASL) ○ Measures tissue perfusion, particularly cerebral blood flow. ○ Non-invasive alternative to PET in safe conditions. ○ Pseudocontinuous ASL is a popular sequence. Functional MRI (fMRI) ○ Measures changes in deoxygenated haemoglobin concentration. ○ Indicates real-time brain activity during rest or tasks. ○ Task-evoked fMRI studies brain activity in response to stimuli. ○ Resting-state fMRI explores brain activation in the absence of stimuli. Computed Tomography (CT) ○ Uses X-rays to generate anatomical images. ○ Short scan time but involves exposure to ionizing radiation. ○ Contrast agents may be used for better tissue discernibility. Positron Emission Tomography (PET) ○ In vivo imaging of biological processes using radionuclide tracers. ○ PET is a poor modality for structural imaging, often combined with CT for better results. ○ Short half-life of tracer molecules requires proximity to a PET imaging centre. Electroencephalography (EEG) and Magnetoencephalography (MEG) ○ Functional neuroimaging techniques mapping brain activity in real-time. ○ EEG detects electrical fields, whereas MEG detects magnetic fields. ○ Both techniques provide information on dynamic interaction between brain areas. Discussion Choosing the Right Modality ○ Selection based on the nature of the research question (flow of a fluid through a network of tubes or channels): structure, perfusion, connectivity, or real-time activity. ○ Consideration of patient population needs, tolerability, and safety. Patient Safety Considerations ○ CT and PET involve radiation exposure; precautions are necessary, especially for females of childbearing age. ○ Informed consent should include discussion of benefits and risks. Limitations and Funding ○ Nurse researchers must address funding challenges for neuroimaging research. ○ Collaboration with neuroscientists and radiologists is crucial. ○ Overcoming barriers requires additional education and training. Facilitators for Nurse Engagement ○ Adequate time for research conduct, research education, and support. ○ Nurses positioned to contribute valuable patient-centred expertise to interdisciplinary teams. Conclusion Potential for Nursing Research Neuroimaging offers innovative avenues for nursing research. Nurses can use technology to identify biomarkers, understand disease processes, and demonstrate intervention efficacy. Overcoming barriers and engaging in neuroimaging research is crucial for advancing nursing science. The imaged brain I. Structural Imaging X-ray CT scans: measure density differences in brain tissue. MRI scans: create detailed images of brain anatomy based on changes in proton alignment. Diffusion tensor imaging (DTI): reveals white matter structure and connectivity. Ward (2020) II. Functional Imaging fMRI (functional magnetic resonance imaging): measures changes in blood flow related to brain activity. PET (positron emission tomography): tracks injected radioactive tracers to map brain activity. EEG (electroencephalography): measures electrical activity of the brain with scalp electrodes. III. From Image to Cognitive Theory: Experimental Design Carefully controlled tasks activate specific brain regions. Baseline and control conditions help isolate target cognitive processes. Statistical analysis identifies significant areas of brain activity. IV. Analysing Data from Functional Imaging Preprocessing: corrects for movement and artifacts. Statistical analysis: identifies regions with increased activity. Visualization: generates brain maps illustrating active areas. V. Interpreting Data from Functional Imaging Correlation, not causation: brain activity may reflect but not necessarily cause a particular cognitive process. Reverse inference: inferring cognitive functions from activated brain regions. Multiple interpretations: need for caution and additional evidence. VI. Why Do Functional Imaging Data Sometimes Disagree with Lesion Data? Lesions may disrupt multiple functions, making interpretation complex. Functional imaging measures activity, not necessarily function. Different cognitive processes may contribute to similar results. VII. Brain-reading: Is “Big Brother” Round the Corner? Ethical concerns around potential misuse of brain imaging technology. Privacy and security vulnerabilities. Limits of current technology and interpretation. VIII. Summary and Key Points Functional imaging provides valuable insights into brain activity but requires careful interpretation. Experimental design and data analysis are crucial for drawing accurate conclusions. Ethical considerations and limitations must be acknowledged Functional MRI experiments: acquisition, analysis and interpretation of data (Ramsey et al., 2002) Background/Introduction: Functional MRI (fMRI) is widely used in neuroscience, with ongoing developments in acquisition, processing, and interpretatio n of data. The study focuses on sensitivity for changes in fMRI signal associated with brain function and the design of tasks to invoke brain functions. The mechanisms affecting the blood oxygen level -dependent (BOLD) signal are complex and not well understood, but fMRI results agree with known functional topography Methods: Acquisition of data is commonly achieved with techniques that measure BOLD signal changes, and data analysis involves modelin g sources of signal variation and reducing noise in the data time-series Analytical algorithms such as connectivity and component analysis contribute to the extraction of meaningful information from fMRI datasets Results: The study presents an example of a hypothesis -driven experiment that investigated whether practice of a working memory task caused a shift in the neuronal representation of working memory Analysis of fMRI data generally involves multiple regression to model the sources of signal fluctuation, and connectivity ana lyses are mathematically possible Critical Analysis: The study emphasizes the importance of testing clear hypotheses using fMRI and expects that this will be done at a larger sca le, at the expense of descriptive 'brain mapping' studies The interpretation of connectivity maps is limited without firm neuroanatomical hypotheses about the brain structures involve d in the network being examined The study highlights the need for sophisticated questions and tasks that allow for accurate modeling of involved brain functi ons in the future Can you synthesise this study in bullet points in terms of background/introduction (include relevant stats and figures), methods, results (include relevant stats and figures), and critical analysis (make the analysis related to the context of the study and elaborate on the analysis) ? can you synthesise this information into bullet points and put them under PSYC0031 Cognitive Neuroscience Page 1 Extra Imagine you're in a dark room full of bouncing balls. When you turn on a light, the balls jump up and down (excited protons). Some balls settle back down quickly (T1), while others take longer (T2). This "settling down" is called relaxation. T1: ○ This is how fast the balls get back in line with the light after jumping (lining up with the magnetic field). ○ Different types of balls (tissues) have different T1 times, so they settle at different speeds, creating different brightness levels in a special picture called a T1-weighted MRI. T2: ○ This is how quickly the balls' bouncing energy fades away (magnetic signal decays). ○ Different types of balls lose their energy at different rates, again leading to different brightness in a special picture called a T2weighted MRI, which is good for spotting differences in tissues or problems. T2:* ○ Sometimes, the room isn't perfectly lit (magnetic field variations), which can mess with how quickly the balls' energy fades. ○ T2* takes these variations into account for a more accurate picture, especially for things like blood vessels that are affected by light changes. can you synthesise this information into bullet points and put them under subheadings, include lots of detail, include relevant stats and figures, use and rephrase in easy-to-understand language : PSYC0031 Cognitive Neuroscience Page 2