Lecture 13 - Bulk & Surface Properties PDF
Document Details
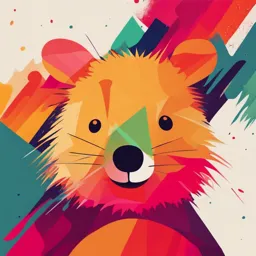
Uploaded by ErrFreeMountRushmore505
University College Dublin
Dimitrios I. Zeugolis
Tags
Summary
This lecture covers bulk and surface properties of biomaterials, focusing on mechanical and dynamic mechanical analysis (DMA) methods. Topics include definitions of surface and bulk, their importance, and various testing techniques for evaluating these properties.
Full Transcript
Bulk & Surface Properties of Biomaterials Dimitrios I. Zeugolis; BSc (Hons), MSc, PhD Regenerative, Modular & Developmental Engineering Laboratory (REMODEL), Charles Institute of Dermatology, Conway Institute of Biomolecular & Biomedical Research, Schoo...
Bulk & Surface Properties of Biomaterials Dimitrios I. Zeugolis; BSc (Hons), MSc, PhD Regenerative, Modular & Developmental Engineering Laboratory (REMODEL), Charles Institute of Dermatology, Conway Institute of Biomolecular & Biomedical Research, School of Mechanical & Materials Engineering, University College Dublin (UCD), Dublin, Ireland www.ucd.ie MEEN40630 Biomaterials www.remodel.ie What is ‘surface’? What is ‘bulk’? Which one is more important? MEEN40630 Biomaterials What is surface? Roughness Surface mobility Wettability Chemical composition [e.g. natural biopolymers (e.g. collagen, fibronectin, elastin) have cell adhesion sides, whilst synthetic polymers do not] What is bulk? Mechanical properties (e.g. stress, strain, modulus, etc.) Thermal properties (denaturation temperature) Chemical structure (e.g. metallic bonds, ionic, covalent) Optical properties Piezoelectric properties Electrochemical properties MEEN40630 Biomaterials Are surface and bulk properties important? Influence pre- and post- implantation properties Are time dependent (surface first, bulk later; why?) MEEN40630 Biomaterials Bulk: Mechanical properties Five basic types of mechanical loading situations: (A) Tensile / Tension: A sample is subjected to a controlled tension until failure. (B) Compression: Compression tests are used to determine a material’s behavior under applied crushing loads and are typically conducted by (A) (B) (C) (D) (E) applying compressive pressure to a test specimen (usually of either a cuboid or cylindrical geometry) using platens or specialized fixtures on a universal testing machine. (C) Shearing: A shear test is designed to apply stress to a test sample so that it experiences a sliding failure along a plane that is parallel to the forces (A) (B) (C) (D) (E) applied. Generally, shear forces cause one surface of a material to move in one direction and the other surface to move in the opposite direction so that the material is stressed in a sliding motion. (D) Torsion: Twisting a sample along an axis. It is a useful test for acquiring information like torsional shear stress, maximum torque, shear modulus, and breaking angle of a material or the interface between two materials. (E) Bending / Flexure / Transverse beam: Measures the behavior of materials subjected to simple beam loading. MEEN40630 Biomaterials Bulk: Mechanical properties Mechanical Properties Derived from Tensile Test Property Formula Unit Young’s Modulus E = Stress / Strain N / m2 (Pa) UTS = Force at break / Original Area (Engineering Ultimate tensile strength / Stress at break N / m2 (Pa) stress) Increase in length required to cause failure Strain % divided by the original length MEEN40630 Biomaterials Dynamic Mechanical Analysis (DMA) Dynamic mechanical analysis: A technique in which the sample's kinetic properties are analysed by measuring the strain or stress that is generated as a result of strain or stress, varies (oscillates) with time, applied to the sample. Static viscoelasticity measurement: A technique in which the change in stress or strain is measured under uniform stress or strain that remains constant across time. Top figure: The sample is clamped in the measurement head of the DMA instrument. During measurement, sinusoidal force is applied to the sample via the probe. Deformation caused by the sinusoidal force is detected and the relation between the deformation and the applied force is measured. Properties such as elasticity and viscosity are calculated from the applied stress and strain plotted as a function of temperature or time. Bottom figure: DMA is used for measurement of various types of polymer materials using different deformation modes. There are tension, compression, dual cantilever bending, 3-point bending and shear modes, and the most suitable type should be selected depending on the sample shape, modulus and measurement purpose. Viscoelastic properties such as: -Storage modulus: E', G' (purely elastic component) -Loss modulus: E", G" (purely viscous component) -Loss tangent: tanδ (=E"/E'), can be measured by DMA, and their dependence on temperature and frequency can be analysed. MEEN40630 Biomaterials Bulk: Mechanical properties of reconstituted collagen fibres as a function of crosslinking Treatment State (number of replicates) Strain at Break ± SD Force at Break ± SD (N) Modulus at 2.2% Strain ± SD (MPa) Dry (n = 4) 0.37 ± 0.07 2.55 ± 0.12 154.23 ± 22.09 DW Overnight Wet (n = 5) 0.33 ± 0.07 0.20 ± 0.04 3.78 ± 1.10 Dry (n = 5) 0.55 ± 0.06 1.71 ± 0.09 102.25 ± 22.98 Genipin Wet (n = 5) 0.40 ± 0.03 0.59 ± 0.09 5.54 ± 3.95 Dry (n = 6) 0.53 ± 0.08 2.63 ± 0.23 59.18 ± 25.20 EDC-NHS Wet (n = 4) 0.54 ± 0.11 0.34 ± 0.03 1.76 ± 0.33 Dry (n = 5) 0.55 ± 0.05 1.62 ± 0.23 34.20 ± 19.10 GTA Wet (n = 5) 0.43 ± 0.04 0.77 ± 0.04 6.90 ± 3.29 Dry (n = 7) 0.29 ± 0.11 1.53 ± 0.10 14.79 ± 5.78 HMDC Wet (n = 5) 0.45 ± 0.15 1.11 ± 0.41 4.39 ± 2.13 MEEN40630 Biomaterials Bulk: Mechanical properties of biomaterials and tissues Elastic Modulus (GPa) Yield Strength (MPa) Tensile Strength (MPa) Strain at Failure (%) Al2O3 350 - 1,000-10,000 0 CoCr Alloy 225 525 735 10 316SS 210 240 600 55 Ti 6Al-4V 120 830 900 18 PMMA 3.0 35-50 0.5 Polyethylene 0.4 30 15-100 PLLA 300 35 Bone (cortical) 15-30 30-70 70-150 0-8 Cartilage 7-15 20 Rat tail tendon 2.1-2.7 120-360 13-31 MEEN40630 Biomaterials Bulk: Thermal properties The thermal properties dictate processing conditions (what does that mean in real life?). The behaviour of materials upon heating, provides information about the material’s chemical or physical structure. Thermal Gravimetric Analysis (TGA), Dynamic Mechanical Thermal Analysis (DMTA), Differential Scanning Calorimetry (DSC) are the most widely used methods employed to evaluate the thermal properties of biomaterials. TGA provides quantitative measurement of mass changes associated with transition and thermal degradation. DMTA is an analytical technique used to understand the relationships between the various viscoelastic parameters (e.g. storage modulus) and temperature of viscoelastic materials. Collagen is a semi-crystalline material due to the presence of ordered and disordered regions. ❑ Shrinkage temperature, measured by DSC, is considered as the melting temperature of the crystalline region ❑ Glass transition, measured by DMTA, is associated with the amorphous region of collagen. DSC is a sensitive technique to investigate thermal changes that are brought about upon heating materials in dry or wet state. In materials science, DSC is employed to evaluate the crystalline structure of materials by measuring the enthalpy of denaturation under dry conditions. MEEN40630 Biomaterials Bulk: Thermal properties Peak temperature is defined as the temperature of maximum power absorption during denaturation. Onset temperature is the temperature at which the tangent to the initial power versus temperature line crosses the baseline. MEEN40630 Biomaterials For biomaterial applications, should DSC be run in dry or wet state? Why? MEEN40630 Biomaterials Bulk: Thermal properties Sample Description ΔHD (J/g) Onset (0C) Peak (0C) Dry 32.86 ± 2.56 96.19 ± 1.42 102.04 ± 1.17 Collagen type I Wet 3.15 ± 0.86 47.61 ± 1.75 52.04 ± 0.53 Dry 8.36 ± 0.13 96.83 ± 0.43 101.56 ± 2.33 Gelatin A Wet 3.20 ± 0.64 27.71 ± 1.11 34.48 ± 1.04 Dry 203.40 ± 3.29 59.15 ± 0.50 67.73 ± 0.33 PEG 40 KDa Wet 1.47 ± 0.18 43.87 ± 0.25 59.88 ± 0.69 Dry 222.87 ± 4.42 60.58 ± 0.10 65.54 ± 0.05 PEO 900 KDa Wet 0.19 ± 0.12 47.40 ± 2.32 57.76 ± 1.09 Dry 76.70 ± 2.50 55.54 ± 0.47 65.66 ± 1.16 PCL Wet 73.65 ± 2.12 53.02 ± 0.65 64.33 ± 1.43 Dry 0.46 ± 0.21 51.77 ± 0.91 55.07 ± 1.72 Chitosan Low Mw Wet 0.33 ± 0.48 52.51 ± 4.43 58.03 ± 1.49 MEEN40630 Biomaterials Bulk: Thermal properties In the biomaterials field, DSC is widely used to measure the denaturation temperature of biomaterials. Biomaterials are materials designed for implantation and continuous contact with body fluids. As such, DSC experimentation should be carried out in the wet state to reflect an in vivo environment. MEEN40630 Biomaterials Other bulk properties Optical properties: ❑The colour of a transparent material is controlled by composition, and therefore demands a high degree of quality control to avoid impurities that could adversely affect colour. ❑Long-term stability of material composition is also important: components should not selectively diffuse into the surrounding tissue, and no chemical changes should occur—either by reactions between the components or in response to light. When light crosses the interface between two media (materials), it is deviated from its original path by an angle that is an increasing function of the difference between the refractive indices of the media. Therefore, the effectiveness of a material as a lens is directly related to its refractive index. Refractive index increases along with the content of electron-rich atoms, which is why lead “crystal” is so useful in both lenses and decorative glassware. ❑Transparency is a qualitative term that describes the ability of a material to transmit light without attenuating (absorbing or scattering) it. To minimize absorption, the primary bonds in the material must be strongly covalent or ionic (and definitely not metallic). Scattering is minimized if the material is free of internal interfaces (which could reflect light) and compositional differences (which would be associated with refractive index differences that could deviate light from an uninterrupted path through the material). Thus, an optimally transparent material will either be a homogeneous single crystal or it will be completely and homogeneously amorphous. There is no commonly accepted quantitative definition of transparency. Instead, it is usual to consider the complementary property, opacity. Piezoelectric properties: Piezoelectricity deals with the coupled mechanical and electrical properties of a material that becomes electrically polarized when it is mechanically strained. There are two forms of piezoelectric effect. Direct piezoelectric effort describes the generation of electric dipoles and polarization as a result of displaced atoms and the associated redistribution of electrons inside a solid when it is mechanically strained. This polarization in turn generates an electric field. By contrast, reverse piezoelectric effect is a phenomenon in which a solid becomes strained when placed in an electric field. MEEN40630 Biomaterials Other bulk properties Electrochemical properties: The electrochemical properties of a biomaterial (typically of metals and alloys) are the material's characteristics in an electrochemically corrosive environment, including electrochemical potentials, reaction constants, etc. These properties dictate the responses of such metallic biomaterials governed by corrosive oxidation and reduction reactions. Oxidation processes take metal (zero valence) and increase its valence to make ions (cations) or oxides (or other solid oxidation products). Reduction processes may include water and oxygen reduction to form hydroxide ions and/or reactive oxygen intermediates, aside from many other biologically based molecules that are susceptible to redox processes. The oxidation and reduction reactions are electrically connected through the metal and complete the circuit through the solution (commonly known as the two half-cell reactions), resulting in currents (electronic and ionic) through both phases. All metallic biomaterials exhibit some form of corrosion process, whether passive dissolution of ions through the passive film, to more aggressive process caused by wear or crevice geometries. Fortunately, the common implantable metals and alloys (with the exception of noble metals such as gold or platinum) have protective oxide films to control corrosion of the material to acceptable levels. These oxides are commonly referred to as passive films, typically of only a few nanometers in thickness, and will limit transport of metallic ions to the implant surface. MEEN40630 Biomaterials Why is surface important? MEEN40630 Biomaterials Surface properties Why is surface important? ❑ Biomaterials interact with host / Communication with cells ❑ Outermost layers are critical / Every surface is reactive ❑ Interaction with surface of biomaterials modifies biological response ❑ The surface is different from the bulk and the mass of a material that makes up the surface zone is very small ❑ Upon implantation, the immune system reacts to biomaterials and chronic response may be brought about ❑ Surfaces readily contaminate can result in implant failure ❑ Release of toxic products or breakdown by-products may cause death of surrounding cells and tissues ❑ The body might try to encapsulate the foreign material in a fibrotic capsule and thus isolate the implantable device ❑ Surface interactions will determine the success of a scaffold ▪ Mimic extracellular matrix supramolecular assemblies ▪ Provide framework for cell attachment, proliferation and growth ▪ Provide framework for the new functional neotissue formation and growth MEEN40630 Biomaterials How deep is the surface? MEEN40630 Biomaterials Surface properties How deep is the surface? The surface is the zone where the structure and composition, influenced by the interface, differ from the average (bulk) composition and structure. This value often scales with the size of the molecules making up the surface. For an “atomic” material, for example gold, after penetration of about five atomic layers (0.5-1 nm), the composition becomes uniform from layer to layer (i.e., you are seeing the bulk structure). At the outermost atomic layer, the organization of the gold atoms at the surface (and their reactivity) can be substantially different from the organization in the averaged bulk, but it is not the atomic/molecular rearrangements we are discussing here. The gold surface, in air, will always have a contaminant overlayer, largely hydrocarbon, that may be roughly 2 nm thick. This results in a difference in composition between bulk and surface. For a polymer, the unique surface zone may extend from 10 to 100 nm (depending on the polymeric system and the chain molecular weight). An interface is the transition between two phases, in principle an infinitely thin separation plane. An interphase is the unique compositional zone between two phases. For the example, for gold, we might say that the interphase between gold and air is 3 nm thick (the structurally rearranged gold atoms + the contaminant layer). MEEN40630 Biomaterials Surface properties What might be measured to define surface structure? (A) Surfaces can be rough, stepped, or smooth. (B) Surfaces can be comprised of different chemistries (atomic, supramolecular, macromolecular). (C) Surfaces may be structurally or compositionally inhomogeneous in the plane of the surface such as phase-separated domains or microcontact printed lanes. (D) Surfaces may be inhomogeneous with depth into the specimen or simply overlayered with a thin film. (E) Surfaces may be highly crystalline or disordered. (F) Crystalline surfaces are found with many organizations such as a silicon unreconstructed surface or a silicon (7 × 7) reconstructed surface. MEEN40630 Biomaterials Surface properties; Overview of methods available Method Principle Depth analysed Spatial resolution Analytical sensitivity Cost Liquid wetting of surfaces is used Low or high depending on the Contact angles to estimate the energy of 3–20 Å 1 mm 250,000 US$ electrons of characteristic energy A focused electron beam Auger electron spectroscopy stimulates the emission of 50–100 Å 80 Å 0.1 atom % > 250,000 US$ (damaging to organic materials) auger electrons Ion bombardment sputters SIMS 10 Å (Static) –1 μm (Dynamic) 100 Å Very high > 250,000 US$ secondary ions from the surface IR radiation is adsorbed and FTIR-ATR 1–5 μm 10 μm 1 mol % 5,000-250,000 US$ excites molecular vibrations Measurement of the quantum tunneling current between a STM 5Å 1Å Single atoms 5,000-250,000 US$ metal tip and a conductive surface Secondary electron emission SEM induced by a focused electron 5Å 10–40 Å High, but not quantitative >50,000 US$ beam is spatially imaged MEEN40630 Biomaterials Surface properties: Wettability (measure via contact angle) Which surface is hydrophobic (does not like water) and which surface is hydrophilic (likes water)? Why? MEEN40630 Biomaterials Contact angle Contact angle analysis involves measuring the angle of contact θ between a liquid and a surface When a drop of liquid is placed on a surface, it will spread to reach a force equilibrium, in which the sum of the interfacial tensions in the plane of the surface is zero Surface energy quantifies the disruption of chemical bonds that occurs when a surface is created Thomas Young described surface energy as the interaction between the forces of cohesion and the forces of adhesion which, in turn, dictate if wetting occurs If wetting occurs, the drop will spread out flat. In most cases, the drop will bead to some extent and by measuring the contact angle formed where the drop makes contact with the solid the surface energies of the system can be measured. (A) An equilibrium is established between surface tension forces contracting a liquid drop to a spherical shape and forces interacting the drop with the surface. The force balance between the liquid–vapor surface tension (γlv) of a liquid drop The increased free energy per unit area for creating a new surface, is directly proportional to the and the interfacial tension between a solid and the drop (γsl), manifested tendency of molecules to adsorb through the contact angle (θ) of the drop and can be used to quantitatively characterize the surface–vapor interfacial tension (γsv). (B) The Zisman method permits a critical surface tension value, an approximation to the solid surface Contact angles directly measure surface wettability and indirectly probe surface energy, tension, to be measured. Drops of liquids of different surface tensions are roughness, heterogeneity, contamination, and molecular mobility. placed on the solid, and the contact angles of the drops are measured. The plot of liquid surface tension versus angle is extrapolated to zero contact angle to give the critical surface tension value. MEEN40630 Biomaterials Contact angle Static or Sessile Drop Method: The most commonly used technique is the static or sessile drop method. The experiment normally calls for the successive addition of fluid droplets until a plateau in the contact angle is reached. Immediately following the advancing contact angle experiment, it is useful to obtain a ‘receding contact angle’ value by monitoring the contact angle as equivalent volume droplets of fluid are successively retracted from the droplet. Wilhemly Plate Method: The Wilhemly plate method is ideal for double-sided samples that need to be tested in temperature-controlled conditions. Certain surfaces may be temperature-sensitive; they are hydrophobic at one temperature and hydrophilic at another. The temperature of a beaker of water is easier to monitor and maintain at a constant temperature than the temperature of fluid droplet. So, the contact angle method has been altered for those samples that need a higher level of control. Captive Air Bubble Method: An alternative to the Wilhemly plate method is the captive air bubble method. In this method, the contact angle is measured between an air bubble of defined volume and the solid surface immersed in the temperature-controlled bath. Capillary Rise Method: The capillary rise method presents the only method of contact angle measurement available for the measurement of tubular materials and coatings. Temperature may be maintained in this method over a short period of time. Five ways that the contact angle (q) can be measured. (A.) Sessile or Static drop. Tilted-drop Measurement: The tilted-drop measurement is another angle measurement. In this technique, a (B.) Wilhelmy plate method. droplet is added to the surface and the advancing and retreating contact angle are measured as the (C.) Captive air bubble method. surface is tilted up until the droplet reaches a point where it almost moves. This technique is useful to (D.) Capillary rise method. measure both the receding and advancing contact angles at the same time. (E.) Tilting substrate method. MEEN40630 Biomaterials Contact angle Issues associated with contact angle measurements: Material Critical surface tension (dynes/cm) Polytetrafluoroethylene 19 ❑ The measurement is operator dependent (for manual, goniometer instruments) Poly(dimethyl siloxane) 24 ❑ Surface roughness influences the results Poly(vinylidine fluoride) 25 Poly(vinyl fluoride) 28 ❑ Surface heterogeneity influences the results Polyethylene 31 ❑ The liquids used are easily contaminated (typically reducing their γlv) Polystyrene 33 ❑ Liquid evaporation and temperature changes can impact measurement Poly(2-hydroxyethyl methacrylate) 37 Poly(vinyl alcohol) 37 ❑ The liquids used can reorient the surface structure Poly(methyl methacrylate) 39 ❑ The liquids used can absorb into the surface, leading to swelling Poly(vinyl chloride) 39 ❑ The liquids used can dissolve the surface Polycaproamide (nylon 6) 42 Poly(ethylene oxide)-diol 43 ❑ Few sample geometries are appropriate for contact angle measurement Poly(ethylene terephthalate) 43 ❑ Information on surface structure must be inferred from the data obtained Polyacrylonitrile 50 MEEN40630 Biomaterials Contact angle examples Contact angle (º) Surface Energy (mN/m) Material Water Diiodomethane Surface Energy Dispersive component Polar Component PGCL 10/90 86 ± 4 37 ± 5 39 ± 0 36 ± 0 3±0 PGLCLTMC 70/5/15/10 81 ± 1 31 ± 0 43 ± 0 40 ± 0 4±0 PGDTMC 55/15/30 86 ± 4 71 ± 2 25 ± 0 17 ± 0 8±0 PLTMC 80/20 95 ± 4 56 ± 1 29 ± 0 27 ± 0 2±0 PGL 15/85 85 ± 3 64 ± 2 28 ± 0 20 ± 0 8±0 PGL 30/70 85 ± 4 59 ± 6 30 ± 0 24 ± 0 5±0 MEEN40630 Biomaterials Swelling Traditionally is measured through weight difference Collagen films were incubated in 1x PBS at room temperature overnight. Prior to being weighed, films were quickly blotted with filter paper to remove excess surface water. The swelling ratio (%) was calculated as [(wet weight – dry weight) / dry weight] * 100 %. For fibres, it is measured through fibre diameter difference Collagen fibres were incubated overnight in PBS (pH 7.4) at room temperature. Subsequently, the fibres were removed and quickly blotted using a filter paper to remove excess surface water. The swelling ratio was then calculated as: 100 x [(Mean Wet Fibre Diameter) – (Mean Dry Fibre Diameter)] / (Mean Dry Fibre Diameter). MEEN40630 Biomaterials Swelling of reconstituted collagen fibres as a function of crosslinking Treatment State Diameter ± SD (µm) Swelling (%) Dry (n = 4) 171 ± 6 DW Overnight 74.27 Wet (n = 5) 298 ± 17 Dry (n = 5) 240 ± 32 Genipin 41.67 Wet (n = 5) 340 ± 47 Dry (n = 5) 201 ± 35 DPPA 54.23 Wet (n = 3) 310 ± 44 Dry (n = 5) 260 ± 26 GTA 17.69 Wet (n = 5) 306 ± 29 Dry (n = 7) 332 ± 75 HMDC 1.20 Wet (n = 5) 336 ± 26 MEEN40630 Biomaterials Chemical composition methods (examples) X-Ray Photoelectron Spectroscopy (XPS) XPS, also called electron spectroscopy for chemical analysis (ESCA), is a technique that allows analysis of the mesoporous material composition based on the electronic state of the species present in the materials. This technique utilizes photoelectrons emitted from the elements within the mesoporous sample upon illumination with X-ray beams with a specific energy (in eV) under extremely high vacuum. The absorption of X-ray photons by the sample leads to ionization and emission of inner shell electrons, whose energy is then measured by an electron-energy analyzer and compiled in a photoelectron spectrum, usually in the range of 0–1400 eV. From the energy profile, the kind of elements or molecular species present in the materials is deduced. Compositional determination of mesoporous materials is possible because each element in the mesoporous material, or in any sample for that matter, has a distinct binding energy that will result in a series of characteristic peaks in the photoelectron spectrum. Shifts in these characteristic peaks occur as changes in electronic or binding interactions of the molecule are altered depending on the specific functional groups present in the materials. Furthermore, the technique allows the determination of possible changes that the functional group in the materials may undergo during chemical conversions (e.g., changes in oxidation states during catalysis). This method is especially powerful in combination with FTIR and solid-state NMR techniques and may allow the full elucidation of the compositions of and the different groups present in the mesoporous materials. However, since XPS is a surface analytical technique as X-rays penetrate only to a certain depth of the sample (ca. < 20 nm), the technique is most suited for analysis of the upper most layers of materials and is thus especially useful for thin film type mesoporous materials. Despite this, XPS can still be successfully used for analysis of the bulk composition of mesoporous materials if it is coupled with other surface etching techniques. Secondary Ion Mass Spectroscopy (SIMS) Secondary ion mass spectroscopy (SIMS) is a valuable technique for identifying the structure and composition of polymer surfaces and complements ESCA. ESCA spectra for similar materials are difficult to resolve, while SIMS can differentiate among several polymers. This is partly due to the smaller sampling depth required by SIMS. In a typical analysis, the surface of the polymer sample is bombarded by primary ions at low current density, principally intended to minimize alteration of the sample surface by irradiation. The polymer surface generates positive and negative ions that are analysed using a mass analyser. The results of detailed analysis provide chemical structure and composition data about the surface. A traditional shortcoming of SIMS, however, is its inability to perform quantitative analysis. Fourier Transform Infrared Spectroscopy (FTIR) Fourier transform infrared (FTIR) spectroscopy is a form of vibrational spectroscopy that is useful in the study of a variety of chemical processes. In the mid-infrared (mid-IR) range, vibrations arise from many environmentally important molecules. It is possible to utilize FTIR spectroscopy as a quantitative analytical method and also as a tool to determine bonding mechanisms in solids and on surfaces. Molecular vibrations can be related directly to the symmetry of molecules, and so it is often possible to determine precisely how a molecule is bonding on surfaces or as a component in a solid phase from its infrared spectrum. Many experimental methods exist for probing samples of various states and at different spectral regions. MEEN40630 Biomaterials Topography analysis using microscopy techniques; SEM Scanning electron microscopy (SEM) SEM functions by focusing a relatively high-energy electron beam (typically, 5–100 keV) on a specimen that is under vacuum. Low-energy secondary electrons (1–20 eV) are emitted from each spot where the focused electron beam impacts. The intensity of the secondary electron emission is a function of the atomic composition of the sample and the geometry of the features under observation. The image of the surface is spatially reconstructed on a phosphor screen / detector from the intensity of the secondary electron emission at each point. Because of the shallow penetration depth of low-energy electrons produced by the primary electron beam, only the secondary electrons generated near the surface can escape and be detected. Nonconductive materials observed in the SEM are typically coated with a thin, electrically grounded layer of metal (e.g. gold) to minimize negative charge accumulation from the electron beam. However, this metal layer is always sufficiently thick (>200 Å) that the electrons emitted from the sample beneath cannot penetrate. Therefore, in SEM analysis of non-conductors, the surface of the metal coating is, in effect, what is being monitored. If the metal coat is truly conformal, a good representation of the surface geometry will be conveyed. However, the specimen surface chemistry no longer influences secondary electron emission. Also, at very high magnifications, the texture of the metal coat and not the surface may be under observation. ESEM: environmental SEM (wet samples) – IS THIS IMPORTANT? Collagen fibre Collagen sponge Electrospun fibres MEEN40630 Biomaterials Topography analysis using microscopy techniques; STM Scanning Tunnelling Microscopy (STM) The STM capitalizes on quantum tunnelling to generate an atom-scale, electron density image of a surface. A metal tip terminating in a single atom is brought within 5–10 Å of an electrically conducting surface. At these distances, the electron cloud of the atom at the “tip of the tip” will significantly overlap the electron cloud of an atom on the surface. If a potential is applied between the tip and the surface, an electron tunnelling current will be established. For most metals, a 1 Å change in the distance of the tip to the surface results in an order of magnitude change in tunnelling current. Even though this current is small, it can be measured with good accuracy. To image a surface, this quantum tunnelling current is used in one of two ways. In constant current mode, a piezoelectric driver scans a tip over a surface. When the tip approaches an atom protruding above the plane of the surface, the current rapidly increases, and a feedback circuit moves the tip up to keep the current constant. Then, a plot is made of the tip height required to maintain constant current versus distance along the plane. In constant height mode, the tip is moved over the surface and the change in current with distance travelled along the plane of the surface is directly recorded. The STM measures electrical current and therefore is well suited for conductive and semiconductor surfaces. However, biomolecules (even proteins) on conductive substrates appear Schematic diagram illustrating the principle of the scanning amenable to imaging. STM does not “see” atoms, but rather monitors electron density. tunneling microscope—a tip terminating in a single atom permits localized quantum tunneling current from surface features (or atoms) to tip. This tunneling current can be spatially reconstructed to form an image. MEEN40630 Biomaterials Topography analysis using microscopy techniques; AFM Atomic Force Microscopy (AFM) The AFM uses a similar piezo drive mechanism to STM. However, instead of recording tunnelling current, the deflection of a tip mounted on a flexible cantilever arm due to van der Waals forces and electrostatic repulsion/attraction between an atom at the tip and an atom on the surface is measured. Atomic-dimension measurements of cantilever arm movements can be made by reflecting a laser beam off a mirror on the cantilever arm (an optical lever). A one-atom deflection of the cantilever arm can easily be measured by monitoring the position of the laser reflection on a spatially resolved photosensitive detector. Other principles are also used to measure the deflection of the tip. These include capacitance measurements and interferometry. Tips are important in AFM as the spatial resolution is significantly associated with tip terminal diameter and Schematic diagram illustrating shape. Tips are made from micro-lithographically fabricated silicon or silicon nitride. Also carbon whiskers, nanotubes, and a the principle of the AFM. variety of nano-spherical particles have been mounted on AFM tips to increase their sharpness or improve the ability to precisely define tip geometry. Tips are also surface modified to alter the strength and types of interactions with surfaces (static SIMS can be used to image these surface modifications). Finally, cantilevers are sold in a range of stiffnesses so the analysis modes can be tuned to the needs of the sample and the type of data being acquired. Since force is being measured and Hooke’s law applies to the deformation of an elastic cantilever, AFM can be used to quantify the forces between surface and tip. Quantitative AFM is now widely used to measure the strength of interaction between biomolecules and the mechanical properties of proteins. Some AFM modes are contact, lateral force, noncontact, tapping, force modulation, and phase imaging. In contact mode, the tip is in contact with the surface (or at least the electron clouds of tip and surface essentially overlap). The pressures resulting from the force of the cantilever delivered through the extremely small surface area of the tip can be damaging to soft specimens (proteins, polymers, etc.). However, for more rigid specimens, excellent topographical imaging can be achieved in contact mode. In tapping mode, the tip is oscillated at a frequency near the resonant frequency of the cantilever. Polyacrylamide film The tip barely taps the surface. The force interaction of tip and surface can affect the amplitude of oscillation and the oscillating frequency of the tip. In standard tapping mode, the amplitude change is translated into topographic spatial information. Many variants of tapping mode have been developed, allowing imaging under different conditions and using the phase shift between the applied oscillation to the tip and the actual tip oscillation in the force field of the surface to provide information of the mechanical properties of the surface (in essence, the viscoelasticity of the surface can be assessed). MEEN40630 Biomaterials AFM analysis examples Material Roughness (nm) PGCL 10/90 90 ± 52 PGLCLTMC 70/5/15/10 69 ± 41 PGDTMC 55/15/30 114 ± 40 PLTMC 80/20 76 ± 48 PGL 15/85 93 ± 42 PGL 30/70 99 ± 37 Tensile Analysis AFM Analysis Material Young’s Modulus (MPa) Young’s Modulus (kPa) PGCL 10/90 110 ± 8 7±3 PGLCLTMC 70/5/15/10 114 ± 17 6±2 PGDTMC 55/15/30 111 ± 14 10 ± 3 PLTMC 80/20 1,288 ± 22 12 ± 3 PGL 15/85 1,601 ± 121 22 ± 9 Why is the tensile modulus different PGL 30/70 2,184 ± 132 15,019 ± 2,916 from the AFM modulus? MEEN40630 Biomaterials Why is the tensile modulus different from the AFM modulus? ‘In the study of a biomaterial for tissue engineering applications, it is essential to understand their potential to endure load present in the native tissue, and also to predict the effect that the biomaterial will have to the surrounding matrix. Literature has shown that native tissues show marginally different magnitude of mechanical properties at their macro- and nano-scale. The mechanical properties of the produced films were assessed using macro- (10-3 to 10-1 m, uniaxial tensile test) and nano- (10-6 to 10-4 m, nanoindentation) techniques to fully elucidate the potential of the scaffolds as implantable Tensile Analysis AFM Analysis devices and as cell culture substrates, respectively. The classical understanding of elastic Material Young’s Modulus (MPa) Young’s Modulus (kPa) behaviour of structures and materials, where the Young’s modulus is a material property that does not depend on size and structures follow standard Hooke’s law and Euler- PGCL 10/90 110 ± 8 7±3 Bernoulli theory. Young’s modulus is a fundamental mechanical property that affects the stiffness and for macroscopic structures, it is considered as a bulk material property, PGLCLTMC 70/5/15/10 114 ± 17 6±2 independent of size that can be obtain using uniaxial mechanical tests. However, nanoscale physical properties of materials, such as mechanical, electrical and thermal PGDTMC 55/15/30 111 ± 14 10 ± 3 properties, can be different from the bulk values as observed in this work. AFM can provide PLTMC 80/20 1,288 ± 22 12 ± 3 information about the mechanical properties of a surface at a length scale that is limited only by the dimensions of the AFM tip. When probing mechanical properties, the attractive PGL 15/85 1,601 ± 121 22 ± 9 and repulsive force interactions between the tip and sample are monitored. Measurements PGL 30/70 2,184 ± 132 15,019 ± 2,916 at such low levels are of particular importance to assess and understand cell responses to substrate elasticity, as cells sense matrix elasticity at molecular scale level via activation of mechanotransduction signalling pathways [e.g. focal adhesion kinase (FAK) and Rho- associated protein kinase (ROCK)] that control several cellular functions, including morphology, adhesion, proliferation, spreading, migration and differentiation.’ S. Ribeiro et al., Acta Biomater, 2021, 121, 303-315, doi: 10.1016/j.actbio.2020.11.026 MEEN40630 Biomaterials AFM analysis examples 1000 kPa 130 kPa 50 kPa (d) (e) 1000 P - Col 1000 G - Col 2.1 µm 94 nm 0.0 µm 0.0 nm Y: m Y: 10 0µ 10 µm µm 1 µm 10 Y: Y: (f) (g) 1000 P + Col 1000 G + Col 2.5 µm 78 nm 0.0 µm 0.0 nm Y: Y: m m 10 10 µm 1 0µ µm 1 0µ Y: Y: Collagen type I coating PDMS substrate stiffness Surface roughness (nm) (h) (i) (mg/ml) 130 P - Col 130 G - Col 1.9 µm 86 nm 0.0 µm 0.0 nm 0 19 ± 23 Y: 10 µm 1 0µ m Y: 10 µm 10 µm 1,000 kPa Y: Y: 0.5 17 ± 21 (j) (k) 130 P + Col 130 G + Col 0 9±7 2.0 µm 0.35 µm 130 kPa Y: m 0.0 µm Y: 1 m 0.00 µm 0.5 29 ± 21 10 0µ 0µ 0µ µm 1 m 1 Y: Y: (l) (m) 0 12 ± 7 50 kPa 50 P - Col 50 G - Col 0.5 12 ± 6 1.9 µm 0.0 µm 96 nm 0.0 nm Y: Y: m m 1 10 µm 1 0µ 0µ 1 0µ m Y: Y: Symbol (in figure) explanation: (n) (o) P: Planar G: Grooved 50 P + Col 50 G + Col 1.9 µm 0.18 µm - Col: without collagen type I coating Y: 10 m 0.0 µm Y: 10 m 0.00 µm 0µ 0µ + Col: with collagen type I coating µm Y: 1 µm Y: 1 1,000, 130, 50 KPa MEEN40630 Biomaterials Scanning Probe Microscopy Modes MEEN40630 Biomaterials Additional methods for surface characterisation of biomaterials MEEN40630 Biomaterials Non-fouling surfaces Non-fouling surfaces (NFSs) or protein-resistant surfaces or stealth surfaces refer to surfaces that resist the adsorption of proteins and/or adhesion of cells. In general, surfaces that strongly adsorb proteins will generally bind cells and surfaces that resist protein adsorption will also resist cell adhesion. In general, hydrophilic surfaces are more likely to resist protein adsorption and hydrophobic surfaces will usually adsorb a monolayer of tightly adsorbed protein. How might NFSs work to inhibit protein adsorption? Most NFSs seem to have strong interactions with water. This water–polymer interaction highly hydrates and expands surface hydrophilic polymer chains. Also, the NFSs bind water tightly, and this water shield separates the proteins from the material of the surface. An important area for NFSs focuses on bacterial biofilms. Bacteria are believed to adhere to surfaces via a conditioning film of organic molecules that adsorbs first to the surface. The Several distinct processes govern biofilm formation bacteria stick to this conditioning film and begin to exude a gelatinous slime layer (the biofilm) that aids in their protection from external agents (for example, antibiotics). Such Four surfaces that can show non-fouling layers are particularly troublesome in medical devices, where they can stimulate significant behavior. The blue dot represents a non- inflammatory reaction to the infected device. If the conditioning film can be inhibited, bacterial fouling chemical moiety such as PEG adhesion and biofilm formation can also be reduced. NFSs offer this possibility. (CH2CH2O)n. (A) Cross-linked network of long, polymeric chains; (B) polymer NFSs have medical and biotechnology uses as blood-compatible materials (where they may brushes grown from the surface; (C) oligo- resist fibrinogen adsorption and platelet attachment), implanted devices (where they may non-fouling headgroups on a self- reduce the foreign-body reaction and simplify device removal), biosensors (where they may assembled monolayer (the yellow dot is the minimize nonspecific protein adsorption and maximize specific binding), urinary catheters, anchor group such as a thiol); (D) affinity separation processes, microchannel flow devices, intravenous syringes and tubing, surfactant or copolymer adsorbed to the and nonmedical uses such as biofouling-resistant heat exchangers and ship hulls. surface. The hydrophobic tails are represented by turquoise dots. MEEN40630 Biomaterials References / Further reading FTIR: D. Peak, FOURIER TRANSFORM INFRARED SPECTROSCOPY, Editor(s): Daniel Hillel, Encyclopedia of Soils in the Environment, Elsevier, 2005, Pages 80-85 SIMS: Sina Ebnesajjad, Chapter 4 - Surface and Material Characterization Techniques, Editor(s): Sina Ebnesajjad, Surface Treatment of Materials for Adhesive Bonding (Second Edition), William Andrew Publishing, 2014, Pages 39-75 XPS: T. Asefa, V. Dubovoy, 9.09 - Ordered Mesoporous/Nanoporous Inorganic Materials via Self-Assembly, Editor(s): Jerry L. Atwood, Comprehensive Supramolecular Chemistry II, Elsevier, 2017, Pages 157-192 Experimental design: Levin A, Sharma V, Hook L, García-Gareta E. The importance of factorial design in tissue engineering and biomaterials science: Optimisation of cell seeding efficiency on dermal scaffolds as a case study. J Tissue Eng. 2018;9:2041731418781696. Published 2018 Jun 25. doi:10.1177/2041731418781696 S. Bell, Experimental Design, Editor(s): Rob Kitchin, Nigel Thrift, International Encyclopedia of Human Geography, Elsevier, 2009, Pages 672-675 Keskin Gündoğdu T, Deniz İ, Çalışkan G, Şahin ES, Azbar N. Experimental design methods for bioengineering applications. Crit Rev Biotechnol. 2016;36(2):368-88. doi: 10.3109/07388551.2014.973014. Epub 2014 Nov 6. PMID: 25373790. Collagen crosslinking: Zeugolis, D. I.; Paul, G. R.; Attenburrow, G., Cross-linking of extruded collagen fibres - A biomimetic three-dimensional scaffold for tissue engineering applications. Journal of Biomedical Materials Research Part A 2009, 89, (4), 895-908. DSC: Zeugolis, D. I.; Raghunath, M., The Physiological Relevance of Wet versus Dry Differential Scanning Calorimetry for Biomaterial Evaluation: A Technical Note. Polymer International 2010, 59, (10), 1403-1407. Bulk: Guigen Zhang, Christopher Viney, 1.2.3 - Bulk Properties of Materials, Editor(s): William R. Wagner, Shelly E. Sakiyama-Elbert, Guigen Zhang, Michael J. Yaszemski, Biomaterials Science (Fourth Edition), Academic Press, 2020, Pages 41-51 MEEN40630 Biomaterials References / Reading material Mechanical properties: Principles of Dynamic Mechanical Analysis (DMA): https://www.hitachi-hightech.com/global/products/science/tech/ana/thermal/descriptions/dma.html Tensile test: https://www.zwickroell.com/industries/materials-testing/tensile-test/ Compression test: https://www.instron.com/en/resources/test-types/compression-test Surface: Buddy D. Ratner, David G. Castner, 1.2.4 - Surface Properties and Surface Characterization of Biomaterials, Editor(s): William R. Wagner, Shelly E. Sakiyama-Elbert, Guigen Zhang, Michael J. Yaszemski, Biomaterials Science (Fourth Edition), Academic Press, 2020, Pages 53-75 Biomaterials characterisation: Ribeiro S, Carvalho AM, Fernandes EM, Gomes ME, Reis RL, Bayon Y, Zeugolis DI. Development and characterisation of cytocompatible polyester substrates with tunable mechanical properties and degradation rate. Acta Biomater. 2021 Feb;121:303-315. doi: 10.1016/j.actbio.2020.11.026. Epub 2020 Nov 20. PMID: 33227488. C.N.M. Ryan, E. Pugliese, N. Shologu, D. Gaspar, P. Rooney, Md N. Islam, A. O'Riordan, M.J. Biggs, M.D. Griffin, D.I. Zeugolis, A combined physicochemical approach towards human tenocyte phenotype maintenance, Materials Today Bio, Volume 12, 2021, 100130 Non-fouling Surfaces: Peng Zhang, Buddy D. Ratner, Allan S. Hoffman, Shaoyi Jiang, 1.4.3A - Nonfouling Surfaces, Editor(s): William R. Wagner, Shelly E. Sakiyama-Elbert, Guigen Zhang, Michael J. Yaszemski, Biomaterials Science (Fourth Edition), Academic Press, 2020, Pages 507-513 MEEN40630 Biomaterials