Respiratory System Lecture 6 PDF
Document Details
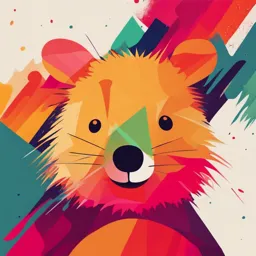
Uploaded by StylishOmaha1982
ATU Sligo
Dr Yasser Abdel-Wahab
Tags
Related
Summary
This document is a lecture on the human respiratory system, covering its anatomy, physiology and functions. It includes an overview of the respiratory system's organization, including the upper and lower respiratory tracts, the importance of oxygen in respiration, and major events in inspiration and expiration.
Full Transcript
Dr Yasser Abdel-Wahab (Module Coordinator) WEEK 6 Introduction to Anatomy and Physiology of the Respiratory System Aims: To give an overview of the Anatomy and Physiology of the Respiratory System Lecture Outlines: 1. The organisation of the respiratory system 2. Overview of the anatomy...
Dr Yasser Abdel-Wahab (Module Coordinator) WEEK 6 Introduction to Anatomy and Physiology of the Respiratory System Aims: To give an overview of the Anatomy and Physiology of the Respiratory System Lecture Outlines: 1. The organisation of the respiratory system 2. Overview of the anatomy of the upper and lower respiratory tract 3. Importance of oxygen in respiration. 4. Primary functions of the respiratory system and types of respiration. 5. Principles governing air movement, ventilation and lung mechanics. 6. Lung compliance and the different modes of breathing. 7. Major events in inspiration and expiration. 8. Respiratory volumes and capacities. 9. Process of diffusion at the respiratory membrane. 10. Mechanisms underlying oxygen and carbon dioxide transport. 11. Control of and major factors affecting breathing 12. Regulation of respiration by the brain. 13. Adaptations in response to low oxygen and exercise. 14. Oxygen as friend and foe. Intended Learning outcomes are: Give an overview of the main divisions of the respiratory system and the respiratory tract. Identify the basic components of the respiratory system and their functions. Describe the primary functions of the respiratory system and the different types of respiration. Describe the physical principles governing air movement, ventilation and lung mechanics. Appreciate the importance of lung compliance and the different modes of breathing. Describe the major events in inspiration and expiration including the movement of the diaphragm and thoracic cage. Appreciate the different respiratory volumes and capacities. Outline the process of diffusion at the respiratory membrane and describe how oxygen and carbon dioxide are transported in the blood. Give an overview of the control of breathing, the major factors affecting breathing and the regulation of respiration by the brain. Appreciate the importance of normal and altered respiratory function in health and disease. 1 Dr Yasser Abdel-Wahab (Module Coordinator) MAIN ORGANS OF THE RESPIRATORY SYSTEM Organs of the respiratory system include: Nose, nasal cavity, pharynx (throat), larynx (voice box), trachea (wind pipe), bronchi and lungs (containing bronchiole tree and alveoli). These can be divided into: Upper respiratory system - Nose & Pharynx and Lower respiratory system - Larynx; Trachea; Bronchi & lungs or in terms of: Conducting portion: Consists of a system of interconnecting cavities and tubes that conduct air into the lungs (nasal cavity, pharynx, larynx, trachea, bronchi and large bronchioles). Respiratory portion: Consists of those portions of the respiratory system where the exchange of gases occurs including the smallest bronchioles and alveoli. Diagram of organs of the respiratory system including branching of airways Divisions of the respiratory system Nose: nasal septum (divides right and left), nasal cavity, vestibule, external portion, external naris (nostrils), internal system, internal nares (conchae). Pharynx (throat): Funnel shaped tube, 13 cm long which starts at the internal nares and extends to the level of Cricoid cartilage (just above thyroid gland). The function of pharynx is providing a passageway for air, food, and a resonating chamber for speech and sounds The pharynx is divided into: 1) The upper most portion Nasopharynx - separated from oral cavity by soft palate Eustachian (auditory) tubes on both sides 2) The middle portion Oropharynx – between soft palate and base of tongue at hyoid bone 3) The Lowest Portion Laryngopharynx or hypopharynx – between hyoid bone and entrance to larynx and esophagus 2 Dr Yasser Abdel-Wahab (Module Coordinator) Larynx (voice box): The wall of the larynx is composed of nine pieces of cartilage as shown in the image below: Three are single: Thyroid cartilage (Adam’s apple) Epiglottis: forms a lid over the glottis Cricoid cartilage Three are paired: Arytenoid cartilages Corniculate cartilages Cuneform cartilages Trachea (windpipe): Passageway for air about 12 cm long and 2.5 cm in diameter located anterior to the oesophagus extends from the larynx to the fifth thoracic vertebra divides into the right and left bronchi Carina – internal ridge separating the right and left bronchi The wall of the trachea consists of a mucosa, submucosa, hyline cartilages and adventitia There are 16 to 20 incomplete rings of cartilage (C shaped) stacked on top of each other. The open portion of the rings faces the anterior of the esophagus Bronchi: the trachea branches into right and left bronchi, with the right having larger diameter than the left. Like trachea they have C shaped rings. These bronchi further subdivide on entering the lungs Secondary (lobular) bronchi – one secondary goes to each lobe of lung 3 lobes in right lung, 2 in left lung Progressively less cartlidge – with small plates around lumen instead of C- shapes Tertiary (segmental) bronchi – each supplies air to a single bronchopulmonary segment (10 segments in right lung, and 8 or 9 in left lung) Bronchioles – no more cartlidge, only smooth muscle Terminal bronchioles - approx 6500 dividing off each tertiary bronchi Methods of examining respiratory system: Bronchography, Bronchogram, Bronchoscopy Lungs: Cone shaped organs in the thoracic cavity separated from each other by the heart and other structures in the mediastinum (see diagram below) Base (bottom of lungs), Apex (top of lungs), costal surface, medistinal (medial) surface and cardiac notch on the left lung Lobes, fissures and lobules Right Lung: 3 lobes – the superior, middle and inferior lobes 3 Dr Yasser Abdel-Wahab (Module Coordinator) Left Lung: 2 lobes – the superior and inferior lobes Lobes are separated by deep Oblique and horizontal fissures Each bronchopulmonary segment of the lung has many small compartments called lobules The lobes are divided by branches of trabeculae (elastic fibers, smooth muscle and lymphatic vessels) in smaller compartments called lobules. These are divided by interlobular septa into pulmonary lobules. In the lobules terminal bronchioles divide to respiratory bronchioles then alveolar ducts which end in multiple and alveolar sacs (approx 150 million alveoli per lung). Alveolar-capillary membrane This membrane is were gas exchange occurs between air in lungs and gases in the blood Consists of (a) Alveolar (epithelial) membrane; (b) Epithelial basement membrane; (c) Capillary basement membrane; (d) Endothelial cells The overall membrane diameter is 0.5 µm. The alveolar epithelium is mostly thin and delicate simple squamous epithelial cells. Septal cells are scattered among the epithelial cells and produce surfactant (oily secretion of phospholipids and proteins) which coats alveolar surface. There are also alveolar macrophages present on the surface of the epithelium to maintain respiratory defence. Diagram of alveoli and the alveolar-capillary membrane Pleural cavity and membranes: There are two pleural cavities separated by the mediastinum, and each lung is maintained in their own pleural cavity. Pleural cavities are lined by a serous membrane (pleura) which is divided into two parts: Parietal pleural: attached to the wall of thoracic cavity, extends over diaphragm and mediastinum Visceral pleura: covers the surface of lungs and extends into fissures The area between the parietal and visceral pleura is lubricated by transduate (pleural fluid) which is secreted by the parietal and visceral pleura and acts to reduce friction caused by inflating and deflating of the lungs. 4 Dr Yasser Abdel-Wahab (Module Coordinator) OYXGEN AND RESPIRATION Importance of oxygen in respiration Oxygen Life-supporting gas (O2) and chemical element. Combines with other chemicals in nearly all living things to produce the energy required for life processes. Also needed for fuels to burn where oxygen combines with the fuel in a chemical reaction releasing energy as heat in the process. How oxygen supports life Land animals and humans get oxygen from air. Fish and most water animals get dissolved oxygen from water. Blood carries oxygen to the cells where it combines with chemicals obtained from food producing energy necessary for cell function. Carbon dioxide is produced by these cells as a waste product. Plants use oxygen in much the same way as animals but can also make oxygen through the process of photosynthesis. Overall function of the respiratory system: 1. Provides extensive area for gas exchange between air and circulating blood. 2. Moves air to and from exchange surfaces of lungs. 3. Protects respiratory surfaces from dehydration, temperature changes and other environmental variations. 4. Provides non-specific defense against invading pathogens. 5. Produces sounds involved in speaking, singing and non-verbal communication. 6. Relays olfactory sensations from nasal cavity to CNS. 7. Helps control body fluid pH. Respiration Is the exchange of gases between atmosphere, blood and cells. Takes place in three steps: – Pulmonary ventilation (or breathing) Inspiration (inflow) and expiration (outflow) of air between atmosphere and lungs. – External (pulmonary) respiration Exchange of gas between air spaces of lungs and blood in pulmonary capillaries. Blood gains oxygen and loses carbon dioxide. – Internal (tissue) respiration Exchange of gases between blood in systemic capillaries and tissue cells. Blood loses oxygen and gains carbon dioxide. Carbon dioxide is generated from cellular respiration. 5 Dr Yasser Abdel-Wahab (Module Coordinator) Diagram representing overview of external and internal respiration and the differences in partial pressures of the gases on gas exchanges mmHg 100 40 40 100 45 40 40 45 40 95 45 45 Maintaining partial pressures of O2 and CO2 helps ensure gas exchange between the lungs and blood and between cells and blood Gases under pressure through thin walled capillaries at lungs and other tissues On average the body tissue take up O2 at a rate of approximately 5 ml per 100 ml of blood and release CO2 at a rate of approximately 4 ml per 100 ml of blood Respiratory Physiology Pulmonary ventilation Is the physical movement of air in and out of RESPIRATORY TRACT Primary function is to maintain adequate alveolar ventilation (air movement into and out of alveoli). This ensures that CO2 does not accumulate in the alveoli and that sufficient O2 is present for absorption into the bloodstream Movement of air into the lungs is controlled by air pressure in the lungs going above and below normal atmospheric pressure. Physical principles governing air movement Diagram representing Boyles law The Gas Laws 1. The total pressure of a mixture of gases is the sum of the pressures of the INDIVIDUAL GASES (Dalton’s Law). 2. Gases, singly or in a mixture, move from areas of higher pressure to areas of lower pressure. 3. If the volume of a container of gas changes, the pressure of gas will change in an INVERSE MANNER (Boyle’s Law), i.e. if 6 Dr Yasser Abdel-Wahab (Module Coordinator) volume decreases the gas pressure increases, and if volume increases the gas pressure decreases. 4. The amount of a gas that will dissolve in a liquid is determined by the partial pressure of the gas and the gas’ solubility in the liquid, i.e. if you increase the gas pressure the gas will move into solution to achieve equilibrium, and if you decrease gas pressure then gas molecules will move out of solution to maintain equilibrium between liquid and gas phases. This is known as Henry’s law. These laws are physical principles governing the properties of gases, their movement and also their diffusion in and out of blood. The diagram below indicates how Henry’s law works, with a can of soda representing a good example of this law. Diagram representing air movement between liquid and gas phase Increasing the pressure When the pressure drives gas molecules into decreases gas molecules solution (new equilibrium leave solution (new established) equilibrium established) Ventilation and lung mechanics Like blood, air moves by bulk flow (F) from high pressure to low pressure (P), i.e.: F=P/R were R = resistance to flow Atmospheric For air to flow in or out of lungs, relevant pressures pressure 760 mmHg are: Air Air – Atmospheric pressure (Patm). – Alveolar pressure (Palv). F= (Patm – Palv)/R Palv < 760 mm Hg Palv > 760 mm Hg Air moves into (inspiration) and out of (expiration) the lungs because the alveolar pressure is made INSPIRATION EXPIRATION 7 Dr Yasser Abdel-Wahab (Module Coordinator) alternately less than, and greater than atmospheric pressure. The movement of air into and out of the lungs is controlled by the pressure within the thoracic cavity, which is controlled by movement of the ribs and diaphragm. Air In this diagram (see right) as the plunger is withdrawn a partial vacuum is created within the pump which draws air into the balloon “Thoracic cavity” causing it to expand. This represents inhalation of air into the lungs. “Lungs If the plunger is pushed into the pump then the vacuum will ” “Thorax decrease and air will be forced out of the balloon. ” Pump plunger During relaxed breathing, when you inhale, Palv drops to around 759 mm Hg. When you exhale Palv increases to around 761 mm Hg. A change of +1 or – 1 mmHg. These changes can reach 860 and 730 mmHg during heavy breathing during exercise. Intrapleural pressure (pressure in the space between parietal and visceral pleurae) also changes during inhalation and exhalation but is usually around -4 mmHg which can reach -18 during strong inhalations. If the visceral or parietal pleura are damaged a pneumothorax can occur with resulting collapased lung, due to air being able to enter the pleural cavity and altering the intrapleural pressure and allowing the elastic fibers of the lung to collapse the lung by recoil. Thus intrapleural pressure is essential to prevent this complete elastic contraction of the lung. Diagram showing pressure changes during inhalation and exhalation 8 Dr Yasser Abdel-Wahab (Module Coordinator) A model of inspiration is shown here (see diagram) Key: Air 1. The chest wall is expanded by contraction of DIAPHRAGM and inspiratory intercostal muscles 4 Chest wall Lung wall (demoted by →). 2. As a result, INTRAPLEURAL pressure decreases (i.e. becomes more sub-atmospheric than between breaths). 3. Pressure difference across lung wall (Palv – Pip 1 (intrapleural pressure) becomes larger and lung wall is PUSHED OUT (i.e. lungs begin to expand). 4. Therefore, alveolar pressure becomes SUB- ATMOSPHERIC, a pressure difference between 3 alveoli and atmosphere is created, and air moves in Intrapleural fluid 2 from the atmosphere. Lung expansion and compliance The degree of lung expansion at any instant is directly proportional to the pressure difference across the lung wall (i.e. between alveolar air and intrapleural fluid, Palv - Pip). Amount any given pressure difference which expands a lung depends on STRETCHABILITY or compliance of lung tissue. Lung compliance Defined as the magnitude of the change in lung VOLUME produced by a given change in pressure difference across lung wall. The larger the ratio (lung volume change / pressure difference change), the greater the COMPLIANCE (i.e. the greater the stretchability) The less compliant the lung the more energy is required for a given amount of EXPANSION. Single most important determinant is surface tension at air-water interfaces within alveoli. A low surface tension increases total lung compliance. Surfactant, a phospholipid produced by type II alveolar cells (Septal cells), markedly reduces the cohesive force between water molecules on the alveolar surface, and hence lowers surface tension. Factors affecting compliance Connective tissue STRUCTURE of the lungs – damage such as Emphysema increases decreases compliance Level of SURFACTANT production – respiratory distress syndrome has inadequate surfactant causing collapse of alveoli and decrease in compliance. Mobility of thoracic cage – arthritis and sketelal disorders can reduce compliance Physiologically, perhaps the most important measure is compliance of the intact respiratory system (i.e. lung and chest taken together) 9 Dr Yasser Abdel-Wahab (Module Coordinator) Modes of breathing During the respiratory cycle (single inhalation and exhalation), the respiration can be classified as either: Quiet breathing o Active inhalation o Passive exhalation This can be either: o Deep breathing or Diaphragmatic breathing, where contraction of diaphragm produces increase in thoracic volume, decreasing pressure and drawing air into lungs o Or Shallow breathing or Costal breathing, where contraction of external intercostal muscles of ribs contract and raise rib cage, increasing thoracic volume, decreasing pressure and drawing air into the lungs In both cases, exhalation occurs through elastic rebound, with relaxation of diaphragm or muscles. Forced breathing o Active inhalation o Active exhalation – contraction of internal intercostal muscles and possible also contraction of abdominal muscles, pushing contents of abdomen up against the diaphragm. The major events in the inspiration of air include: 1. Nerve impulses travel on PHRENIC nerve to muscle fibres in diaphragm causing them to contract. 2. As the dome-shaped diaphragm moves DOWNWARD, the thoracic cavity expands. 3. Simultaneously, external intercostal muscles may contract, raising ribs and increasing size of thoracic cavity even more. 4. Intra-alveolar pressure DECREASES. 5. Atmospheric pressure (which is relatively greater on outside) forces air into respiratory tract through air passages. 6. Lungs fill with AIR. The major events in expiration of air include: 1. Diaphragm and external respiratory muscles RELAX. 2. Elastic tissues of lungs and thoracic cage, which were stretched during inspiration, suddenly recoil, and surface tension COLLAPSES alveolar walls. 3. Tissues recoiling around lungs INCREASES intraalveolar pressure. 4. Air is squeezed out of lungs. 10 Dr Yasser Abdel-Wahab (Module Coordinator) The diagram below shows the movement of the diaphragm during respiration (A) and the ribs during inhalation (B) as discussed above (A) (B) Respiratory volumes and capacities The respiratory system is able to adapt rapidly to meat changes in oxygen demand, going from slow quiet breathing to rapid deep breathing easily. This occurs by varying the number of breaths each minute and the volume of air moved into and out of the lungs during each breath. The RESPIRATORY RATE is the number of breaths you take each minute (usually 12- 18 breaths/min) The TIDAL VOLUME (ml) is the volume of air moved into the lungs during inhalation and is equal to the amount of air breathed out during exhalation (usually about 500 ml at rest). The RESPIRATORY MINUTE VOLUME is the amount of new air being brought into the lungs every minute and can be calculated by multiplying the TIDAL VOLUME by the RESPIRATORY RATE. Resting tidal volume: amount of air moved into or out of lungs during single respiratory cycle at rest Expiratory reserve volume (ERV): amount of air which can be voluntarily expelled after a normal resting respiratory cycle Inspiratory reserve volume (IRV): amount of air which can be actively inhaled above the tidal volume Residual volume: volume of air remaining in lungs after maximum exhalation (1100 – 1200 ml) Minimal volume: amount of air which would remain in collapsed lungs Inspiratory capacity: sum of inspiratory reserve volume and tidal volume Vital capacity: the maximum amount of air which can be actively moved into and out of lungs during a respiratory cycle (= ERV + Tidal volume + IRV) and ranges from 3400 – 4800ml Functional residual capacity: volume of air remaining in lungs after a normal resting exhalation (=ERV + residual volume) 11 Dr Yasser Abdel-Wahab (Module Coordinator) At rest only a small amount of air in the lungs is exchanged. The lungs have a much larger capacity as shown below. This diagram shows the capacity of the lungs and volumes of air for these capacities which are highlighted above Type and patterns of ventilation are shown in this table Name Description Examples Eupnea Normal quiet breathing - Hyperpnea Increased respiratory rate and/or Exercise volume in response to increased metabolism Hyperventilation Increased respiratory rate and/or Emotional volume without increased hyperventilation; metabolism blowing up a balloon Hypoventilation Decreased alveolar ventilation Shallow breathing; asthma; restrictive lung disease Tachypnea Rapid breathing; usually Panting increased respiratory rate with increased depth Dyspnea Difficulty breathing (a subjective Various pathologies or hard feeling sometimes described as exercise ‘air hunger’) Apnea Cessation of breathing Voluntary breath holding; Depression of CNS control centres 12 Dr Yasser Abdel-Wahab (Module Coordinator) Diffusion of gases at the Respiratory Membrane Diffusion at the respiratory membrane is very efficient because: 1. Differences in partial pressure across respiratory membrane are substantial. – Greater differences in partial pressure result in greater rate of gas DIFFUSION. – Increased partial pressure for oxygen (PO2) in alveoli results in increased oxygen diffusion into BLOOD – Decreased PO2 in alveoli results in drop in OXYGEN diffusion into blood. 2. Distances involved in gas exchange are small. – Fusion of alveoli and alveolar basement membrane reduces the distance to 0.5 µm. – Inflammation increases this distance, impairing alveolar gas exchange. 3. Gases are lipid soluble. – Oxygen and carbon dioxide diffuse readily through MEMBRANES. 4. Total surface area is large. – Up to 140 m2. – Damage to alveoli (e.g. emphysema) reduces surface area and hence efficiency of gas transfer. 5. Blood flow and airflow are co-ordinated. – Improves efficiency of pulmonary ventilation and pulmonary circulation. – Blood flow is greatest around alveoli with highest PO2 values, where oxygen uptake can proceed with maximum EFFICIENCY. – Pulmonary embolism or pulmonary obstruction can break down this co- ordination, thus reducing efficiency. The two main gases transported in the blood are oxygen and carbon dioxide. The table below shows the features of gas transport in the blood. Gas Reaction involved Substance transported Oxygen Combines with iron atoms of Oxyhaemoglobin haemoglobin molecules Carbon dioxide About 7% dissolves in plasma Carbon dioxide About 23% combines with Carbaminohaemoglobin amino groups of haemoglobin molecules About 70% reacts with water Bicarbonate ions to form carbonic acid which dissociates to release hydrogen and bicarbonate ions 13 Dr Yasser Abdel-Wahab (Module Coordinator) OXYGEN TRANSPORT Oxygen transport requires binding to haemaglobin contained in the red blood Haemoglobin has 4 globular subunits which each have an iron-containing haem group which can transport up to 4 oxygen molecules There are a number of factors which can alter bioavailable haemaglobin levels thereby affecting transport of oxygen in the blood. These include: Nutritional deficiences (iron deficiency, B vitamin and folate deficiencies) Chronic blood loss o Resulting in reduced Hb concentration o Reduction in oxygen carrying capacity This leads to development of anaemia, which has symptoms including: Weakness Tiredness Reduced exercise tolerance Each red blood cell has about 280 million Hb molecules, with the potential to carry up to one billion oxygen molecules per red cell. Haemoglobin saturation curves and oxygen Haemoglobin saturation: is defined as the percentage of haem units containing bound oxygen at a given moment. If all haemoglobin molecules in blood are fully loaded with oxygen molecules then haemoglobin saturation is 100%. The amount of saturation of haemaglobin with oxygen at different partial pressures of oxygen pO2 is represented by the oxygen-haemaglobin saturation curve as shown in the diagram below. Diagram showing oxygen-haemoglobin saturation curve Any factor which alters the SHAPE or FUNCTION of Hb molecule will ALTER O2 binding. If pO2 INCREASES Hb saturation goes UP Hb STORES O2 If pO2 DECREASES Hb saturation goes DOWN Hb RELEASES O2 14 Dr Yasser Abdel-Wahab (Module Coordinator) NB: Any change in the saturation curve indicates a change in O2 carrying properties of Hb rather than a change in Hb concentration There are differences in partial O2 pressure at the respiratory membrane and at the tissues requiring oxygen, thus altering the ability of Hb to bind (lungs) or release (tissues) O2 Factors affecting the oxygen-haemaglobin saturation curve Factors which can affect haemoglobin and the oxygen carrying function of haemaglobin include: pO2 (mmHg) of blood pH of blood temperature metabolic activity in red blood cells anaemia foetal development Shifting of oxygen-haemoglobin saturation curve to the right The flow chart below shows factors which shift the oxygen-haemoglobin saturation curve to the right and the reason for this shift In addition: In red blood cells glycolysis leads to the formation of 2,3 diphosphoglycerate (2,3- DPG), and an elevation of 2,3-DPG also causes the curve to shift to the right, prompting the release of oxygen from Hb. Importance of pH on oxygen-haemoglobin saturation curve As shown in the chart below, increases and decreases in pH alter the shape of haemoglobin (Hb) thereby altering the oxygen binding properties of Hb. These effects of pH on Hb saturation are known as the Bohr effect. An increase in pH moves the O2-Hb saturation curve to the left. 15 Dr Yasser Abdel-Wahab (Module Coordinator) In addition this shift to the left also occurs when temperature is decreased. CO2 is the main factor affecting pH. CO2 entering the blood from tissues rapidly enters RBC’s. The CO2 reacts reversibly with H2O (catalyzed by the enzyme carbonic anhydrase) to form carbonic acid (H2CO3) which dissociated in H+ and HCO3-. The H+ diffuse into plasma and decrease the blood pH. Other factors affecting oxygen-haemoglobin saturation curve Anaemia The diagram below shows the effects of anaemia on O2 content of blood Anaemia can result from iron deficiency, and a lack of iron translates into markedly reduced ability of Hb ability to transport O2 Foetal development The diagram below shows the oxygen-haemaglobin saturation curve for foetal blood Foetal Hb has higher affinity for O2 This allows the transfer of essential O2 from maternal Hb to the higher affinity foetal Hb at the placenta Ensures foetus receives adequate O2 supply from mother 16 Dr Yasser Abdel-Wahab (Module Coordinator) CARBON DIOXIDE TRANSPORT Carbon dioxide is generated by all peripheral tissues via aerobic metabolism. The build up of CO2 at the tissues is prevented by transport of CO2 to the lungs for expiration into the air. CO2 is transported by one of three means: Conversion to carbonic acid (approx 70% of total CO2) Binding to haemoglobin (approx 23%) Dissolved in plasma (approx 7%) Carbonic acid formation CO2 diffuses into blood and enters RBC’s. It is then converted to carbonic acid by reaction with water catalyzed by the enzyme carbonic anhydrase as shown: CO2 + H2O ↔ H2CO3 ↔ H+ + HCO3- H+ can bind to Hb molecules to prevent huge changes in pH (Hb acts like a buffer). HCO3- moves out of RBC’s by exchange with chloride ions which enter the cells (known as chloride shift – mass movement of Cl- into RBC’s). Binding of CO2 to haemoglobin The CO2 binds to the globular portion of the Hb molecule (not the iron containing haem site) by attachment to exposed amino groups (-NH2) forming carbaminohaemoglobin. Plasma transport In the plasma, CO2 is transported as dissolved gas molecules The diagram below summarizes the transport of CO2 17 Dr Yasser Abdel-Wahab (Module Coordinator) It is important to remember that all reactions of O2 and CO2 involved in transport of these molecules in blood are temporary and reversible. The diagram below summarizes the uptake, transport and release of O2 and CO2 from tissues and the lungs (alveoli). OVERVIEW OF THE CONTROL OF BREATHING Respiration is controlled by both involuntary and voluntary mechanisms Involuntary centres in the brain regulate activity of respiratory muscles and adjust the frequency and depth of pulmonary ventilation to regulate respiratory minute volume o By response to sensory information from lungs o And response to sensory information from other sites Voluntary control of respiration: o Activity in cerebral cortex affects output of respiratory centres (in Medulla Oblongata and Pons o Or spinal cord motor neurons which control respiratory muscles As shown in the diagram below, the respiratory centres in the brainstem include the rhythmicity area in the Medulla Oblongata (divided into ventral respiratory groups and dorsal respiratory groups) and the pneumotactic area of the Pons Ventral respiratory group: Active during forced breathing only Control accessory respiratory muscles involved in: o Active exhalation o Maximal inhalation 18 Dr Yasser Abdel-Wahab (Module Coordinator) When neurons stimulating exhalation are active then neurons stimulating inhalation are inhibited and vice versa. Dorsal respiratory group: Active for both quiet and forced breathing (all respiratory cycles) Regulates external intercostal muscles and diaphragm Pneumotactic area Adjusts output of rhythmicity area groups Regulates rate and depth of breathing in response to sensory stimulo or signals from other centres in the brain Diagram showing regulation of breathing through respiratory centres in the brain The table below shows factors affecting breathing 19 Dr Yasser Abdel-Wahab (Module Coordinator) Regulation of respiration Activities of respiratory centres can be modified by sensory information from: 1. CHEMORECEPTORS sensitive to pCO2, pH and/or pO2 of blood or CSF. 2. Changes in blood pressure in aorta or carotid sinuses. 3. Stretch receptors (BARORECEPTORS) responding to changes in lung volume. 4. Irritating physical or chemical stimuli in nasal cavity, larynx or bronchial tree (NB. Irritants trigger MECHANORECEPTORS reflexes which protect lungs). 5. Other sensations Including: - pain, - change in body temperature - abnormal VISCERAL sensations. Adaptions resulting from low O2 availability Acclimatization At high altitudes the air “is thin”–that is it contains less oxygen. Acclimatization to low pressure of O2 (hypoxia) is coupled with an improved capacity of blood to transport O2. This enhanced capacity is due to an increase in the number of red blood cells (polycythemia). This non-permanent physiological change is advantageous to athletes –with enhanced O2 transport capacity at “normal” altitude. Disease states In chronic diseases of the respiratory system such as emphysema there is an inadequate supply of oxygen to the lungs hence tissues. The body attempts to compensate for this by inducing a similar polycythemia. Notably emphysema can destroy as much as half the ability of the lungs to function before the individual realizes something is wrong. As the heart has to pump harder to get enough oxygen into the blood, emphysema can cause heart ailments that can cause death. Respiratory effects of exercise The rates of gas exchange in both peripheral tissues and lungs are greatly increased during exercise Increased tissue O2 supply depends on: Increases in cardiac output and local blood flow Increased O2 extraction from capillaries (i.e. lower pO2 of tissues) Increased pulmonary O2 uptake depends on: Increased pulmonary blood flow (equaling cardiac output) Increased O2 diffusion gradient in lungs (with increased ventilation) 20 Dr Yasser Abdel-Wahab (Module Coordinator) Oxygen debt after exercise: Ventilation rates do not return to normal immediately after prolonged heavy exercise Oxygen debt arises as ATP consumption by muscles exceeds production by oxidative metabolism O2 consumed at increased rate until oxidative metabolism restores creatine phosphate levels and reduced lactic acid levels to normal (i.e. paying back the O2 debt). The diagram below shows the ventilatory response to exercise Pulmonary Function Tests In clinical practice forced vital capacity and forced expiratory volume are often used to classify respiratory disease as either RESTRICTIVE or OBSTRUCTIVE. Forced vital capacity (FVC) Reduced in restrictive lung diseases such as those that limit compliance(e.g. lung fibrosis) or reduction in lung volume (e.g. removal or collapse) Forced expiratory volume (FEV) Reduced in obstructive lung diseases (e.g. asthma) but ratio of FEV1/FVC is most useful diagnostically(normally should exceed 75 %) Peak expiratory flow (PEF) Amount of air blown out of fully inflated lungs Reduced in obstructive lung diseases (normally around 400 litres/min) Machines for measuring lung function include: Spirometer - measures parameters such as vital capacity. 21 Dr Yasser Abdel-Wahab (Module Coordinator) Pneumotachometer - determines the rate of air movement throughout the respiratory cycle Peak-flow meter - records the maximum rate of air movement during forced exhalation. Oxygen – both friend and foe Oxygen made possible aerobic metabolism with “improved biological energy”(increased supply of high-energy phosphates) and decreased level of undesirable products of anaerobic metabolism (e.g. lactic acid). While oxygen is utilized within the cell (mitochondria) to produce energy, too much oxygen can damage or even destroy the cell. Most oxygen consumed in the body is fully reduced to water (by the mitochondrial oxygen transport chain). However, the reduction of oxygen can also can also pass through two intermediate stages: forming superoxide anion radical (O2.- ) and hydrogen peroxide (H2O2). Both O2.- and hydrogen peroxide can form even more damaging hydroxyl free radical, hydroxyl ion, and singlet oxygen (by Haber-Weiss or Fenton reactions). Oxygen-derived free radicals target key proteins, DNA and lipids (lipid peroxidation) and extensive damage results in cell death. Survival in this dangerous oxidising environment relies on protective mechanisms (e.g. superoxide dismutase, catalase and other antioxidants). Reading Lists: Martini FH & Nath JL, Fundamentals of Anatomy and Physiology, San Francisco, Pearson Benjamin Cummings. Martini’s Fundamentals of Anatomy and Physiology was specially selected for this module on the basis of the quality of the textbook, the inclusion of the valuable Fundamentals of Anatomy and Physiology. It comes with Interactive CD-ROM and supporting WWW site (freely accessible to students purchasing this text). 22