BMS 200 - Macrocirculatory and Microcirculatory Physiology PDF
Document Details
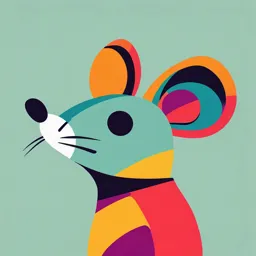
Uploaded by DivineRed
SFU
Tags
Summary
These notes cover Macrocirculatory and Microcirculatory Physiology, focusing on the relationship between venous return, cardiac output, and blood pressure, along with the characteristics of different blood vessels like elastic arteries and capillaries. It also touches on factors like hemorrhage simulation and the function of baroreceptors.
Full Transcript
BMS 200 – Macrocirculatory and Microcirculatory Physiology Objectives Describe the relationship between venous return, cardiac output, mean arterial pressure, pulse pressure, systolic pressure, diastolic pressure, and systemic vascular resistance Relate the following characteristics of elas...
BMS 200 – Macrocirculatory and Microcirculatory Physiology Objectives Describe the relationship between venous return, cardiac output, mean arterial pressure, pulse pressure, systolic pressure, diastolic pressure, and systemic vascular resistance Relate the following characteristics of elastic arteries, muscular arteries, arterioles, capillaries, venules, and veins to their physiologic role Compliance and elasticity Pressure and fluid velocity Cross-sectional area and distribution of total blood volume Describe the histological structure of elastic arteries, muscular arteries, arterioles, capillaries, venules, and veins Describe the mechanisms of venous return and relate it to cardiac preload Describe the cellular physiology of the major baroreceptors and relate it to the overall function of the baroreceptor reflex Apply Poiseuille’s law to the regulation of blood pressure Compare turbulent to laminar flow and describe situations when each would occur Objectives Explain why arterioles are the major site of blood pressure regulation Relate the histological structures of the microcirculation to their physiologic function Contrast the locations as well as the functional and structural differences between continuous, fenestrated, and sinusoidal capillaries Describe the how Starling forces determine bulk flow across the capillary endothelium and relate filtration to the development of edema and inflammation Describe the major mechanisms of autoregulation in the microcirculation and how it suffices to meet the needs of the tissue Describe the role of the autonomic nervous system and the renin- angiotensin-aldosterone system in the homeostatic control of blood pressure Contrast the regulation of blood flow in the following vascular beds: Cerebral, pulmonary, coronary, renal, skeletal muscle, cutaneous Simulating hemorrhage You can simulate hemorrhage by placing a patient in a chamber that applies negative pressure to the lower body ▪ Why is this system a smart experimental system? ▪ What would be the impact on the cardiac system once the vacuum is turned on? ▪ Think about this for end of lecture https://www.sfu.ca/aerospacelab/research-areas/orthostatic- intolerance/lower-body-negative-pressure.html Blood Vessel Histology – General Concepts The walls of arteries and veins contain 3 distinct layers: ▪ Tunica intima ▪ Tunica media ▪ Tunica externa Blood Vessel Histology – General Concepts Tunica Intima: continuous sheet of simple squamous endothelial cells (endothelium) lining the lumen various amounts of subendothelial connective tissue (CT) Internal elastic lamina, a thin layer of elastic fibers, forms the outermost boundary of the tunica intima Blood Vessel Histology – General Concepts Tunica Media: Thickest layer in arteries Circularly arranged smooth muscle cells and fibroelastic CT ▪ elastic content increases greatly with the size of the vessel External elastic lamina, an elastic fiber-rich layer, forms the outermost boundary of the tunica media Blood Vessel Histology – General Concepts Tunica Adventitia: outermost layer of the vessel wall, consisting of dense irregular CT In larger vessels, the tunica adventitia houses vasa vasorum ▪ small blood vessels that supply the tunica adventitia and media thickest of the three layers in veins Arterial Vessels – General Characteristics Points of Note ▪ Elastic arteries have a greater number of elastic membranes for dealing with high-pressure blood flow from the heart. ▪ Muscular arteries have more smooth muscle layers for regulating blood flow to various regions of the body. ▪ Arterioles control the flow into capillary beds and regulate blood pressure through constriction or dilation. ▪ Metarterioles act as transitional vessels between arterioles and capillaries, controlling blood flow into capillaries. Arteries – Elastic and Muscular Elastic arteries: - More elastic fibres ▪ relatively thin external elastic lamina ▪ Very pulsatile, and are the major pressure reservoirs of the circulation ▪ Designed to handle high-pressure blood flow near the heart Muscular arteries: - More smooth muscle ▪ Most of the arteries of the body that are named are muscular arteries ▪ Still pulsatile, but do not serve a major pressure reservoir function ▪ Although a large layer of smooth muscle, cannot completely vasoconstrict and “cut off” blood flow, and not the major source of peripheral resistance ▪ Distribute blood to various organs and tissues, controlling flow through smooth muscle contraction Arteries – Elastic and Muscular Elastic Arteries (Conducting Arteries): Aorta Pulmonary arteries (pulmonary trunk) Common carotid arteries Subclavian arteries Common iliac arteries Arteries – Elastic and Muscular Muscular Arteries (Distributing Arteries): Radial artery Femoral artery Brachial artery Coronary arteries Popliteal artery Muscular artery & accompanying vein Arterioles & Metarterioles Arterioles Control systemic blood pressure and direct blood flow into capillary beds through smooth muscle constriction and dilation. Metarterioles Serve as a microvascular control point, regulating blood flow into individual capillaries through precapillary sphincters, fine-tuning the supply to meet local tissue demands. Arterioles & Metarterioles Major sites of regulation in the cardiovascular system ▪ Arterioles can constrict significantly and restrict blood flow to a capillary bed ▪ Pre-capillary sphincters at the junction of metarterioles and capillaries can greatly reduce flow to a capillary bed AND metarterioles can shunt blood directly to venules Types of capillaries Continuous capillaries: Least permeable, found in tissues that need tight control over what passes through Fenestrated capillaries: Moderately permeable, allowing larger molecules to pass, found in organs involved in filtration and absorption Sinusoidal capillaries: Highly permeable, allowing large particles like proteins and cells to pass, found in specialized organs Types of capillaries Continuous– vast majority of capillaries ▪ Intercellular junctions allow movement of water-soluble substances – for areas that require Fenestrated higher filtration or increased delivery of soluble substances ▪ Glomerulus in the kidney ▪ Endocrine glands, lamina propria of the intestines ▪ Fenestrae are covered/altered by proteins that form a sort of diaphragm whose properties can be altered Types of capillaries Sinusoidal ▪ Discontinuous basal lamina ▪ Larger diameter ▪ Massive gaps between the intercellular junctions ▪ Specialized for allowing cells to move in and out of them ▪ Locations: liver, spleen, lymph nodes bone marrow Capillary Overview Overview of Veins Large Veins Examples: Vena cava, pulmonary veins, portal vein. Structure: Tunica Intima: Well-developed, with endothelium and a prominent subendothelial layer. Tunica Media: Thin, containing relatively fewer smooth muscle cells compared to arteries. Tunica Adventitia: Thickest layer, composed of dense connective tissue, collagen, and elastic fibers. Contains vasa vasorum (small blood vessels) to nourish the vein wall. Function: Large veins return deoxygenated blood to the heart from systemic circulation. They are able to accommodate large blood volumes. Overview of Veins Medium Veins Examples: Femoral vein, renal vein, brachial vein. Structure: Tunica Intima: Thin, with endothelium and a thin subendothelial layer. Tunica Media: Thinner than arteries, with scattered smooth muscle cells. Tunica Adventitia: The thickest layer, with collagen and elastic fibers. May have some vasa vasorum. Valves: Present, especially in the limbs, to prevent backflow of blood due to low pressure. Function: These veins drain blood from organs and limbs, using valves to direct blood flow toward the heart. Overview of Veins Small Veins (Venules) Examples: Postcapillary venules, collecting venules. Structure: Tunica Intima: Endothelial cells with a thin basal lamina. Tunica Media: Very few layers of smooth muscle cells or absent in the smallest venules. Tunica Adventitia: Thin layer of connective tissue. Function: Venules collect blood from capillaries and begin the process of returning it to larger veins. Postcapillary venules also play a role in inflammatory responses, allowing white blood cells to exit the bloodstream and enter tissues. Overview of Veins Overview of Veins Many large and medium veins have valves that are formed from reflections of the tunica intima ▪ More notable in the lower extremities than upper Lumen of veins is much larger and much less likely to be constricted than that of an artery ▪ Therefore systemic veins contain close to 2/3 of the body’s blood volume ▪ Large and medium veins can constrict somewhat in response to increased secretion of catecholamines Properties of Large Vessels Hemodynamics = study of how blood moves in the vascular compartments ▪ Impacted by properties of the vessels, properties of the blood, activity of the heart, and the presence of gravity (and position of the patient) ▪ How do you think we get blood back up to the heart from the legs when standing? Properties of Large Vessels Review: Poiseuille’s Law ▪ Describes the flow of an incompressible and Newtonian fluid (like blood in small vessels) through a cylindrical pipe or tube. ▪ It relates fluid flow rate to the vessel's dimensions and pressure differences. ▪ Small Radius = Higher Resistance: According to the law, smaller vessels have higher resistance. This is crucial in regulating blood flow, especially in smaller arteries and arterioles. The expression ▪ Vessel Length and Viscosity: Increased length and viscosity also increase resistance. For instance, above could be conditions like dehydration can increase blood considered viscosity, leading to higher resistance and decreased flow. conductance to flow… the inverse would Properties of Large Vessels Review: Poiseuille’s Law ▪ Q = flow ▪ (P1 – P2) = pressure gradient ▪ r = radius of the vessel ▪ n = viscosity ▪ l = length of the vessel Which parameters will increase flow as they increase? Decrease flow? The expression Flow increases with increased above could be pressure and vessel radius. considered Flow decreases with increased viscosity and vessel length. conductance to flow… the inverse would Properties of Large Vessels Poiseuille’s law only “works” if flow is not turbulent within the arteries – i.e., flow is laminar Laminar flow - where fluid moves in parallel layers, and each layer flows smoothly without mixing with adjacent layers. Properties of Large Vessels If flow becomes turbulent, then flow is proportional to the square root of the pressure gradient ▪ turbulent flow is less “energy efficient” ▪ In turbulent flow, fluid movement is irregular, with eddies, vortices, and chaotic mixing between layers. ▪ This typically occurs at high flow velocities or in areas with sharp changes in vessel diameter (e.g., bifurcations, atherosclerotic plaques). ▪ In turbulent flow, Poiseuille’s law does not apply because the law assumes a constant resistance to flow. However, in turbulent flow, the resistance increases unpredictably due to chaotic fluid motion and energy dissipation. Properties of Large Vessels The Reynold’s number predicts when turbulent flow will occur ▪ When it is > 2,300, flow will be turbulent Reynold’s number calculation circled below ▪ p = fluid density, D = diameter of the vessel, v = velocity of the fluid ▪ n = viscosity What are pathological situations that could increase the likelihood of turbulent flow? Properties of Large Vessels What are pathological situations that could increase the likelihood of turbulent flow? Atherosclerosis Stenosis Hypertension Aneurysms Valvular heart disease, All create conditions that disturb the smooth, laminar flow of blood, increasing the likelihood of turbulence. Turbulent flow is more likely when blood velocity increases, vessel diameter is irregular or constricted, or viscosity decreases. Properties of Large Vessels Bernoulli Principle: The concept that pressure is constant in a system, regardless of velocity If forward velocity increases in a blood vessel, that increases “forward pressure” ▪ What is the impact on the force that is exerted against the wall of the vessel? Hemodynamic Characteristics of Different Vessels Look carefully at this diagram: Can you explain the changes in pressure and velocity in the ▪ arteries? ▪ capillaries? ▪ veins? Hemodynamic Characteristics of Different Vessels Summary of Changes: From Arteries to Capillaries: Pressure: Decreases significantly. Velocity: Decreases significantly (slowest in capillaries). From Capillaries to Veins: Pressure: Continues to decrease but at a slower rate. Velocity: Increases as blood moves back toward the heart. Hemodynamic Characteristics of Different Vessels Arteries: Pressure: Blood pressure is highest in the aorta and large arteries (around 120 mm Hg during systole). As blood travels through smaller arteries, the pressure gradually decreases but remains relatively high compared to other vessels. Velocity: Blood velocity is high in the aorta due to the forceful contraction of the heart. As blood moves into smaller arteries and arterioles, the velocity starts to decrease slightly due to the increased cross- sectional area. Capillaries: Pressure: Pressure drops significantly in the capillaries, usually around 30 mm Hg or lower. This reduction in pressure is crucial for facilitating the exchange of nutrients and waste between the blood and surrounding tissues. Velocity: Blood velocity is lowest in the capillaries. This is essential because slower flow allows more time for the exchange processes to occur. The vast network of capillaries (huge total cross-sectional area) contributes to this reduction in velocity. Hemodynamic Characteristics of Different Vessels Veins: Pressure: After passing through the capillaries, pressure continues to drop as blood enters the venules and larger veins, eventually reaching around 5-10 mm Hg near the vena cava. The pressure in veins is significantly lower than in arteries. Velocity: Blood velocity begins to increase as it returns to the heart through the venules and larger veins, even though the pressure is low. The cumulative effect of the return flow through the veins results in a higher velocity as it approaches the heart, despite the low pressure. Vascular Systems in Series: Series Circulation: In a series arrangement, blood flows through one vessel, then directly into the next, one after the other. This is less common in the body. Example: The arrangement of blood flow from the heart through the aorta and then through various arteries to a single capillary bed is somewhat sequential but isn't truly series in a broad physiological sense. Implication: In a series circuit, the total resistance to blood flow is the sum of the resistances of each individual vessel. This can lead to higher overall resistance and potentially less efficient blood flow. Vascular Systems in Parallel: Parallel Circulation: In a parallel arrangement, blood can flow through multiple vessels simultaneously. This is the predominant arrangement in the body. Example: The systemic circulation is characterized by many capillary beds in parallel. For instance, when blood reaches an organ, it can be distributed through multiple arterioles leading into many capillaries, allowing different tissues to receive blood concurrently. Implication: In a parallel circuit, the total resistance is less than the resistance of any individual vessel. This is because each additional pathway provides an alternative route for blood flow, which reduces the overall resistance and enhances perfusion. This allows for efficient distribution of blood to various tissues and organs Series vs. Parallel Vascular Circuits There are very few vascular systems in the body that are in series – most are in parallel ▪ Using the diagram below, can you describe why the cumulative resistance of the systemic capillary beds are so low, even though the radius of the vessels are so small (Poiseuille’s law says small vessel = higher resistance)? Series vs. Parallel Vascular Circuits Despite individual capillaries having high resistance due to their small radius their parallel arrangement results in low cumulative resistance, enabling effective blood flow and nutrient exchange in the systemic circulation. Central vs. Peripheral Blood Volume Total blood volume in an “average-sized” adult male is ~ 5 L ▪ 80% in the systemic circulation ▪ 60% in systemic veins ▪ Small arteries and capillaries – 20% of blood volume Veins are very compliant – they’re “floppy” and are easy to distend (up to a point) ▪ If you give someone 500 mL of normal saline, 80% of that stays in the systemic circulation, and most of it (95%) locates in the veins… Thus having a modest effect on arterial blood pressure Compliance Compliance describes how much pressure is required to change the diameter (volume) of a structure… in this case a blood vessel the ability of a blood vessel to expand and accommodate changes in blood volume or pressure. High compliance: small amount of pressure 🡪 large change in volume ▪ In general, veins are more compliant than arteries ▪ Compliance of a vessel – especially larger arteries - decreases with age; vessels become “stiffer” Atherosclerosis Calcification Compliance decreases with contraction of smooth muscle within blood vessels Compliance Arterial Compliance Definition: Arterial compliance is the ability of arteries to stretch and expand when blood pressure increases during systole (heart contraction). Characteristics: Low Compliance: Arteries have relatively low compliance, meaning they do not stretch easily. This is due to their thick muscular walls and elastic fibers, which allow them to withstand high pressure from the blood being pumped by the heart. Function: The low compliance helps maintain high blood pressure, ensuring efficient blood flow to various organs. Arteries can also dampen the pressure fluctuations caused by the heartbeat. Compliance Venous Compliance Definition: Venous compliance is the ability of veins to expand and hold more blood without a significant increase in pressure. Characteristics: High Compliance: Veins have high compliance, allowing them to accommodate larger volumes of blood at lower pressures. Their walls are thinner and less muscular compared to arteries. Function: This high compliance serves as a blood reservoir. During periods of rest or when blood volume increases, veins can expand to store extra blood without a drastic increase in pressure, which helps regulate blood flow and maintain venous return to the heart. Central vs. Peripheral Blood Volume Central blood volume (25%) includes: ▪ vena cavae ▪ heart ▪ pulmonary circulation The central blood volume is important for: ▪ determining preload of the heart (optimal ventricular stretch 🡪 optimal force of contraction) ▪ pathologic situations like pulmonary edema due to congestion We can acutely shift large volumes of blood into or out of the central compartments by varying the amount in the peripheral compartments ▪ Peripheral compartments – veins in the abdominal cavity or limbs Central vs. Peripheral Blood Volume Using this knowledge: ▪ What happens when you go from lying down to standing? ▪ Why is it such a big deal to give someone with CHF an extra litre of IV fluid? ▪ Why is it advantageous to constrict our arteries and veins when confronted with a serious hemorrhage? Hemodynamic Physiology Compliant, yet elastic arteries decrease cardiac work ▪ Elasticity = the tendency for a structure to resume it’s original shape once a distending force (pressure) is removed ▪ If the heart was beating into a rigid pipe, no distention would occur and no significant potential energy – elasticity that can be converted to pressure - could be stored in the vessel wall Therefore blood flow would decrease to close to zero during diastole ▪ Every single systole would have to “charge up the pressure” and apply significant kinetic energy to the column of blood Requires more energy usage Hemodynamic Physiology Increasing venous filling pressure increases cardiac output… ▪ due to improved actin-myosin overlap at optimal preload … but at the same time, as cardiac output increases, the central venous blood volume is removed more quickly ▪ due to increased movement of fluid Hemodynamic Physiology The upright posture poses a number of challenges to venous return to the central blood volume ▪ Pressures can be as high as 90 mm Hg in the feet, but 40 mm Hg at the thigh Solution: ▪ Valves in the leg veins of the lower body ▪ Surround the leg veins with skeletal muscle ▪ Breathing in decreases intrathoracic pressure… Which draws blood upwards Mean Arterial Pressure What is the “average” pressure in the arterial system? ▪ Can we average systolic and diastolic pressures together to form a mean arterial pressure (MAP)? ▪ Since 1/3 of the cardiac cycle is spent in systole, then it stands to reason that the average pressure is the diastolic pressure + 1/3 (systolic pressure – diastolic pressure) ▪ Multiple different ways of writing this equation – “easiest” is below Pulse pressure (PP) = systolic pressure (SP) – diastolic pressure (DP) MAP = DP + PP/3 OR MAP = Diastolic Pressure + 1/3(Systolic Pressure - Diastolic Pressure) The Microcirculation Artery Entering an Organ Branching of Arteries: When an artery enters an organ, it undergoes multiple branches (six to eight times). These arteries are known as distributing arteries, as they distribute blood to different regions of the organ. Arterioles Further Branching: The distributing arteries branch into arterioles, which are smaller blood vessels with a diameter of about 10 to 15 microns. Function: Arterioles typically branch two to five times and serve as the primary site for regulating blood flow into the capillaries. They play a key role in controlling resistance and blood pressure. Metarterioles Formation from Arterioles: As arterioles continue to branch, they lead to metarterioles, which are transitional vessels between arterioles and capillaries. Muscle Layer: Muscular arterioles (the larger arterioles) usually have a single layer of smooth muscle that helps regulate blood flow. Discontinuous Muscle: Metarterioles have a discontinuous layer of smooth muscle along their length, which is not uniform like in the larger arterioles. The Microcirculation Capillaries Structure: Metarterioles lead directly to capillaries, which have an internal diameter ranging from 3 to 9 microns. Function: Capillaries are the sites of nutrient and gas exchange between the blood and surrounding tissues. Their small diameter allows red blood cells to pass through in single file, facilitating this exchange. Pre-Capillary Sphincters Role: At the junction of metarterioles and capillaries are pre-capillary sphincters. These are small bands of smooth muscle that regulate blood flow into the capillary beds. Function: When the sphincters are contracted, blood flow into the capillaries is reduced or halted, directing blood flow to other areas or minimizing flow when tissues are not active. When relaxed, they allow more blood to flow into the capillaries for exchange. Basement Membrane of Capillaries Composition: The basement membrane of capillaries is primarily formed by Type IV collagen. This structure supports the capillary walls and provides a scaffold for endothelial cells, ensuring the integrity and functionality of the capillaries. The Microcirculation Continuous Capillaries Overview Structure and Function: Continuous capillaries are characterized by an uninterrupted endothelial lining, which allows them to maintain a relatively tight barrier between the blood and surrounding tissues. Most continuous capillaries are considered “leaky,” permitting the free movement of small, water-soluble substances (like ions, glucose, and amino acids) while restricting larger molecules and cells. Notable Exceptions Blood-Brain Barrier (BBB) Blood-Testes Barrier The Microcirculation Tight Junctions Composition: In these exceptions, the capillaries have tight junctions formed by proteins called occludins and claudins. These proteins connect adjacent endothelial cells and form a more complete seal compared to regular continuous capillaries. Function of Tight Junctions: The tight junctions in these specialized capillaries significantly reduce paracellular permeability (the movement of substances between cells), ensuring that only specific substances can cross the endothelial barrier. This sealing mechanism is crucial for protecting sensitive environments, like the brain and testes The Microcirculation Pinocytosis Process: Continuous capillaries engage in a process known as pinocytosis, where they continuously endocytose (internalize) small pockets of extracellular fluid from the vascular space. This involves the formation of small vesicles that capture fluid and any dissolved substances. Transport Across the Basement Membrane: Once internalized, these vesicles transport the fluid and its contents across the endothelial cells and release them into the surrounding tissue. This mechanism allows for the regulated exchange of substances between the bloodstream and the tissue, even in areas where tight junctions restrict other forms of transport. The Microcirculation Effect of Inflammation Increased Pinocytosis: During inflammation, the rate of pinocytosis in continuous capillaries is increased. This enhanced fluid uptake can help facilitate the transport of immune cells and substances necessary for the inflammatory response, such as antibodies and nutrients, to the affected area. Implication of Increased Permeability: Inflammatory mediators can also lead to changes in the permeability of these capillaries, allowing larger molecules and immune cells to pass through more readily. This is part of the body’s response to injury or infection, as it helps bring more immune cells to the site of inflammation. Continuous capillaries Caveolae – “small caves” ▪ endocytosis and transcytosis of macromolecules across endothelial cells ▪ vesicles can move slowly through the endothelial cell Serve as important structures in endothelial cells that facilitate the endocytosis and transcytosis of macromolecules. They allow for controlled transport across the endothelial barrier while intercellular clefts provide a selective pathway for small molecules. Continuous capillaries Pinocytic vesicles can coalesce to form vesicular channels Intercellular clefts ▪ just small enough so albumin doesn’t get through Movement of substances across capillaries Substances move down their concentration gradient (diffusion) except for water ▪ Water moves down its concentration gradient AND down its hydrostatic gradient ▪ Where the equilibrium lies can be calculated using the Starling equation – REVIEW from BMS 100, see next slide The speed with which substances diffuse down their gradient is determined by Fick’s law ▪ REVIEW as well – slide after next Starling forces - simplified **Starling forces govern fluid exchange in the body by balancing the hydrostatic pressure that pushes fluid out of capillaries and the oncotic pressure that pulls fluid back in.** Variables: = the “leakiness” of the capillary wall to water ▪ The “inverse” of resistance = hydrostatic pressure = osmotic pressure “cap” = the fluid within the capillary “ISF” = the fluid within the interstitial space = how much protein leaks through the capillary wall Fick’s law - defined describes the diffusion of substances, particularly the movement of gases or solutes across a barrier, such as cell membranes or capillary walls. F = flow/flux ▪ number of molecules of a substance diffusing from point A to point B over time = concentration gradient ▪ Difference in concentration on either side of the membrane A = surface area of the membrane = a constant that increases when: ▪ The substance is a smaller molecule that dissolves better in the barrier ▪ The permeability of the barrier to the substance increases t = thickness of the membrane Movement of substances across capillaries Movement of substances across capillary walls is necessary for life ▪ However, too much results in edema Factors that keep water in the capillary: ▪ albumin and other plasma proteins a small amount leaks through 🡪 a small oncotic pressure in the interstitial space Factors that reduce excessive edema in tissue ▪ glycosaminoglycans that absorb water like a “sponge” (lots of CHO moieties) ▪ lymphatics in most tissue We’ll discuss edema more next day Regulation of blood flow at the level of the capillary Autoregulation – refers to the intrinsic ability of a capillary bed to regulate its own blood flow based on local tissue factors, rather than relying on systemic factors like overall blood pressure. This ensures that tissues receive an adequate blood supply that meets their metabolic needs, even when systemic blood pressure varies. Myogenic regulation ▪ Maintains a relatively constant rate of tissue flow despite changes in MAP Higher pressures in the vessel (smaller vessels, i.e. arterioles) 🡪 smooth muscle stretching → increased constriction (stretch-activated calcium channels) Decreased pressure 🡪 decreased stretch → dilation ▪ Likely has very little physiologic significance by itself – metabolic autoregulation seems to be much more important However, some hormonal signals work by changing the sensitivity of myogenic constriction/dilation Autoregulation at work The safe range for blood flow is about 80% to 125% of normal usually occurs at arterial pressures of 60 to 160 mm Hg At pressures above about 160 mm Hg, vascular resistance decreases because the pressure forces dilation to occur at pressures below 60 mm Hg, the vessels are fully dilated, and resistance cannot be appreciably decreased further. Regulation of blood flow at the level of the capillary Autoregulation – metabolic factors ▪ O2, CO2, H+, lactate, adenosine, K+ accumulate and lead to vasodilation Can you explain why these substances change their local tissue concentrations in metabolically active tissue? Important exception – pulmonary microcirculation – see later slides ▪ increased blood flow leads to washout of the same metabolites ▪ Can lead to reactive hyperemia – after a period of vasoconstriction, metabolites build up and lead to a period of vasodilation and greatly increased perfusion ▪ Interestingly, mitochondria will produce H2S in hypoxic situations – may be the major metabolic autoregulatory mediator in some tissues Metabolic effects on arterioles Hormonal factors in regulating tissue flow Vasodilation and nitric oxide NO is produced by endothelial cells - shear stress causes its release from the endothelium ▪ Shear stress = the force placed on a blood vessel along the axis of blood flow (increases with pressure, velocity of flow, turbulence) ▪ Detected by mechanoreceptors that modulate nitric oxide synthase (which produces NO from L-arginine) NO diffuses to nearby vascular smooth muscle ▪ NO activates soluble guanylyl cyclase 🡪 cGMP production 🡪 activation of PK G 🡪 relaxation (through dephosphorylation of myosin) of smooth muscle Net result – shear stress increases 🡪 nitric oxide production 🡪 vasodilation in arterioles 🡪 a decrease in shear stress Nitric oxide mechanism Hormonal Controls Vasodilation Histamine: vasodilates arterioles, constricts venules → edema Bradykinin: circulating protein, activated by inflammatory signals ▪ Potent vasodilator Prostaglandin E2 and I2 Epinephrine, norepinephrine through beta-2 receptors Hormonal Controls Vasoconstriction Epinephrine, norepinephrine through alpha-1 receptors Serotonin – released in tissue damage → local vasoconstriction ▪ When released by platelets or GI tract, sometimes results in vasodilation Thromboxane A and Prostaglandin F constrict 2 Angiotensin II ADH (anti-diuretic hormone) Reaction to damage (platelet plug formation) Hormonal Control - SNS Catecholamines Epinephrine has a much greater effect than norepinephrine on β2 receptors, and they are roughly equal for α1, α2, and β1 In general, β2 causes vasodilation in skeletal muscle, cardiac muscle and liver, and and α receptors cause vasoconstriction in all tissues including muscle Comparison of Tissue Vascular Beds Skeletal Muscle Compression of Capillaries: During muscle contractions, the skeletal muscle fibers shorten and exert pressure on the capillaries that supply blood to the muscle. This compression can temporarily restrict blood flow within the capillary bed during contraction. Emptying the Venous Tree: When skeletal muscles contract, they help pump blood back to the heart by compressing veins. This is particularly effective in emptying the venous tree, which can increase the pressure gradient driving blood flow from arteries to veins. Increased Blood Flow During Exercise Increased Flow Through Capillary Beds: After the muscle contractions stop, there is a rapid increase in blood flow to the capillary beds. This increased flow delivers oxygen and nutrients necessary for recovery and continued activity. Vasomotor Control: Alpha-1 (α1) Receptors: These receptors are typically associated with vasoconstriction of vascular smooth muscle (VSM). Activation of α1 receptors in skeletal muscle arterioles can reduce blood flow. Beta-2 (β2) Receptors: In contrast, β2 receptors mediate vasodilation in response to certain stimuli, such as epinephrine (adrenaline) during physical activity, leading to increased blood flow. Comparison of Tissue Vascular Beds Metabolic Factors Overriding Autonomic Nervous System (ANS) Influence Lactate, Potassium (K+), Adenosine: During intense exercise or muscle activity, metabolic byproducts accumulate: Lactate: Produced during anaerobic metabolism, signaling the need for increased blood flow and oxygen delivery. Potassium (K+): Released by muscle cells during activity, causing local vasodilation to increase blood flow. Adenosine: Released as a result of ATP breakdown; acts as a potent vasodilator. These metabolites override the vasoconstrictive effects mediated by the ANS, leading to vasodilation and enhanced blood flow to active skeletal muscles. Comparison of Tissue Vascular Beds Rest vs. Exercise: Oxygen Extraction and Blood Flow Resting State: At rest, skeletal muscles extract approximately 25-30% of the available oxygen (O2) from the blood flowing through the capillary bed. Blood flow during rest is relatively low, which matches the lower metabolic demands of the muscle at this time. Exercising State: During exercise, blood flow to skeletal muscles can increase up to 20-fold. This dramatic increase is facilitated by vasodilation and recruitment of additional capillaries. The number of perfused capillaries can increase 3 to 4 times, allowing for greater surface area for gas exchange and nutrient delivery. Oxygen extraction can increase to as much as 90% during maximal exercise, meeting the heightened metabolic demands of the working muscle. Comparison of Tissue Vascular Beds Cerebral pH and adenosine regulate neural blood flow ▪ mostly mediated by local increases in H+/adenosine or by carbon dioxide diffusing across the BBB ▪ Other vasomediators blocked by BBB, so it doesn’t really respond to circulating vasodilatory/constricting influences Brain is also capable of modulating ANS activity, thus it can control blood flow to other tissues ▪ wide range of mechanisms, including baroreceptor input, CO2 concentrations, endocrine feedback loops ↑ intracranial pressure → ↓ perfusion ▪ in response to this, the brain increases mean arterial pressure (Cushing reflex) Comparison of Tissue Vascular Beds Pulmonary Controlled by O2 concentrations ▪ constriction in response to decreased oxygen levels ▪ how does this compare to other vascular beds? Why does this make sense? Skin Sympathetic is most important - alpha1 receptors constrict important for regulating body temperature Comparison of Tissue Vascular Beds Coronary Most sensitive to O2 and adenosine Mechanical compression during systole → poorer blood flow ▪ Can you describe two reasons why the heart is in big trouble if diastole shortens too much? Renal Myogenic and tubuloglomerular feedback also controlled by a variety of hormones (catecholamines, angiotensin II) Simulating hemorrhage You can simulate hemorrhage by placing a patient in a chamber that applies negative pressure to the lower body ▪ Why is this system a smart experimental system? ▪ What would be the impact on the cardiac system once the vacuum is turned on? https://www.sfu.ca/aerospacelab/research-areas/ orthostatic-intolerance/lower-body-negative-pressure.html Simulating hemorrhage Definition: Lower Body Negative Pressure (LBNP) simulates hemorrhage by redistributing blood into the lower extremities. Key Concept: Mimics central hypovolemia without physical blood loss. Why is LBNP a Smart Experimental System? Controlled: Precise pressure adjustments mimic varying hemorrhage levels. Non-invasive: No actual blood loss, safe and reversible. Physiological Realism: Accurately simulates cardiovascular effects of hemorrhage. Real-time Monitoring: Continuous tracking of heart rate, blood pressure, and more. Versatile Applications: Study hemorrhage, orthostatic intolerance, shock, syncope. Simulating hemorrhage Cardiovascular Impact of LBNP Decreased Venous Return: Blood pools in lower extremities, reducing preload. Reduced Stroke Volume & Cardiac Output: Less blood fills the heart, decreasing output. Compensatory Tachycardia: Heart rate increases to maintain blood pressure. Peripheral Vasoconstriction: Sympathetic response causes vessel constriction to maintain pressure. Hypotension Risk: Prolonged LBNP can lead to a dangerous drop in blood pressure. BMS 200 – Shock and Ischemic Heart Disease Objectives Categorize edema by pathophysiologic mechanism and common disorders that underly each mechanism Contrast the pathophysiologic mechanisms, situations, and clinical features of hyperemia and congestion Differentiate between hypotension, heart failure, and shock Describe the pathophysiologic mechanisms and pathological entities that contribute to the four categories of shock Describe the major stages of shock Apply the pathophysiology of the stages and categories of shock to the selection of rational therapeutic interventions Distinguish between the following ischemic heart disease entities: Acute coronary syndromes, stable angina, unstable angina, NSTEMI, STEMI, sudden cardiac death, vasospastic (Prinzmetal) angina Objectives Describe the physiologic parameters that determine the metabolic demands of the heart and relate these to the pathophysiology of ischemic heart disease Describe, where clinically and pathophysiologically useful, the biomedical mechanism for the major risk factors for development of CAD as they relate to atherosclerosis Compare the pathophysiologic situations that cause subendocardial and transmural infarcts Describe the pathophysiologic events that occur during cardiac ischemia Describe the basic epidemiology, clinical features, useful diagnostic procedures, complications, and prognoses of the major forms of ischemic heart disease Briefly describe the pharmacologic mechanism of action and related adverse effects of common medications used to treat ischemic heart disease Hmmmm… A quick case: Your 62-year-old uncle had a stent placed in one of his coronary arteries a year ago, and he states that it has had an excellent impact on his energy and general feeling of well-being. At a family dinner he is sitting at rest, appearing uncomfortable. He takes out a spray canister, sprays it inside his mouth, and massages his chest. You ask him how he’s feeling, and he replies it’s the same old chest discomfort that he’s been dealing with over the past few days. It’s been a little more frequent and he attributes it to stress in the workplace as he transitions to retirement. He states that it’s nothing to worry about, it’s the same discomfort he gets if he works out too hard, and it usually goes away pretty quickly. After 5 minutes he uses the spray again. What are your thoughts about this situation? Edema = excess fluid in interstitial spaces Usual causes: ▪ blood hydrostatic pressure increase Arterial or venous ▪ drop in blood oncotic pressure ▪ increased vascular permeability ▪ blockage of lymphatic flow Many pathologies can cause edema via these basic mechanisms ▪ edema fluid that is low in protein and cells is known as a transudate - Low protein, low cellular content, caused by pressure imbalances. ▪ edema that is high in protein and cells is known as an exudate (typical of inflammation) - High protein, high cellular content, caused by inflammation and vessel damage. Starling forces - Recall Describe the movement of fluid across capillary walls Explains how fluid moves between blood vessels and surrounding tissues. These forces are a balance between two pushing forces (hydrostatic pressures) and two pulling forces (oncotic pressures). Starling forces - Recall Variables: = the “leakiness” of the capillary wall to water ▪ The “inverse” of resistance = hydrostatic pressure = osmotic pressure “cap” = the fluid within the capillary “ISF” = the fluid within the interstitial space = how much protein leaks through the capillary wall Edema and the microcirculation Oncotic pressure - the pull force that draws water into the blood vessels.pressure Hydrostatic Mainly - created the pushby large force that proteins. moves water out of the blood vessels into the surrounding tissues. It is generated by the pressure of the blood See notes against thebelow walls of the for description vessels, of in the especially each type arteries. How do Starling forces regulate fluid exchange Moveme between blood vessels (arterioles and venules) and the surrounding tissue (interstitium).? nt of Fluid Arterial Side (Left) Across Pc (Capillary hydrostatic pressure) is higher than πc (Capillary oncotic pressure). Capillari This means that the force pushing fluid out of es the capillary (Pc) is stronger than the force pulling fluid back into the capillary (πc). As a result, fluid is driven into the interstitium (surrounding tissue), depicted by the red arrows moving outward. This fluid helps nourish tissues. Venous Side (Right): Pc is now lower than πc The capillary oncotic pressure (πc), driven by proteins like albumin, pulls fluid back into the Edema Causes 1. Increased hydrostatic pressure ▪ can be caused by a generalized global increase in arteriolar blood pressure One significant example is malignant hypertension, where extreme increases in blood pressure overwhelm the normal balance of fluid movement across the capillaries. Can you think of an endocrine cause? Think of an excess of hormones that regulate blood volume and vascular tone. ▪ Usually caused by the factors indicated on the chart on the previous slide Also Decreased venous drainage which can be regional (i.e. a single obstructed vein) or global (i.e. congestive heart failure) can increase hydrostatic pressure Edema 2. Increased sodium and water retention ▪ some people tend to retain more sodium after increases in their diets ▪ pathologies of the kidneys can impair sodium elimination as can decreased perfusion to the kidneys ▪ Endocrine causes: syndrome of inappropriate ADH secretion adrenal cortical pathologies – too much aldosterone Edema reduced lymphatic drainage ▪ malignancies that infiltrate the lymph nodes ▪ surgeries that resect the lymph nodes ▪ Rarely infections that cause intense fibrosis of lymph nodes and their channels infestation by a parasitic organism – filiariasis ▪ AKA elephantiasis Edema 3. Decreased oncotic pressure ▪ What plasma protein is most responsible for blood oncotic pressure? ▪ Nephrotic syndrome – excess leakage of protein from the glomerulus (renal capillary complex) Protein filters from blood, through glomerular capillary 🡪 protein enters the renal tubules and is excreted in the urine 🡪 decreased oncotic pressure ▪ Hepatic failure ▪ Protein-losing enteropathies or malnutrition Edema 4. Damage to the endothelium or just excessive leakiness can obviously lead to edema ▪ Usually associated with inflammation In tissues that cannot “tolerate” excess interstitial fluid, can be disastrous ▪ i.e. pulmonary edema due to damage to alveolar epithelium and capillary endothelium Edema General clinical features ▪ tends to be dependent edema – more noticeable in areas of the body that are most inferior to the heart ▪ Many renal diseases can cause a generalized edema that is apparent in areas that contain “looser” connective tissue known as anasarca if generalized different from the massive urticarial edema of anaphylaxis ▪ Pulmonary and brain edema are probably the most severe forms of edema the edema is not just a symptom, but a causative factor in the pathophysiology Unique features of the interaction of the microvasculature and air spaces (lungs) and the inflexible cranial cavity (brain) result in more severe consequences Which is anasarca? Which is angioedema? Hyperemia and congestion Both from locally increased blood volume hyperemia: arteriolar dilation (e.g., at sites of inflammation or in skeletal muscle during exercise) leads to increased blood flow ▪ affected tissues turn red (erythema) because of the engorgement of vessels with oxygenated blood ▪Example is the return of blood flow to tissue that is warming after being out in the cold Hyperemia and congestion Both from locally increased blood volume congestion: a passive process resulting from reduced outflow of blood from a tissue (sometimes called passive hyperemia) ▪ can be systemic (heart failure) or local (venous obstruction) ▪ Congested tissues take on a dusky reddish-blue color (cyanosis) due to red cell stasis and the accumulation of deoxygenated ▪ Eventually red blood cells can extravasate, causing hemosiderin deposition in tissues Hemosiderin = degradation product of hemoglobin found mostly within macrophages Congestion Long-standing congestion - chronic passive congestion ▪ Stasis of poorly oxygenated blood causes chronic hypoxia ▪ Result in degeneration or death of cells and tissue fibrosis ▪ Capillary rupture at sites of chronic congestion → small foci of hemorrhage ▪ phagocytosis and catabolism of erythrocyte debris → Accumulations of hemosiderin-laden macrophage Congestion in individual tissues Pulmonary congestion ▪ Acute: Alveolar capillaries engorged with blood ▪ Chronic: Septa become thickened and fibrotic Alveolar spaces contain hemosiderin-laden macrophages ("heart failure cells") You’ll learn more about pulmonary histology later this semester Hepatic congestion ▪ Acute: Hepatocytes degenerate, sinusoids and venules are distended with blood Those near the hepatic artery circulation undergo less severe hypoxia and develop fatty change Pulmonary congestion Not much air in that lung… Congestion in individual tissues Fig 7-5 Passive congestion of liver. A. photomicrograph of liver shows dilated centrilobular sinusoids. The intervening plates of hepatocytes exhibit pressure atrophy. B. A gross photograph of liver shows nutmeg appearance, reflecting congestive failure of the right ventricle. C. Late changes in chronic passive congestion characterized by dilated sinusoids (arrows) and fibrosis (note the blue staining of collagen in this trichrome stain). Venous Congestion Type Causes Appearance Clinical Features Pulmonary Left heart failure, mitral Engorgement of pulmonary Shortness of breath stenosis or regurgitation capillaries and venules, (dyspnea), wheezing, alveolar edema, heart difficulty breathing with failure cells, brown lying flat (orthopnea) induration Hepatic Right heart failure, Enlarged liver, centrilobular Right upper abdominal constrictive pericarditis necrosis, nutmeg liver pain, elevated liver enzymes, ascites, peripheral edema, jugular venous distension Deep Veins Blood clot formation (DVT), Dilated and tortuous veins, Swelling, pain, tenderness, incompetent valves venous ulcers, potential of skin changes thrombus formation White vs. Red Infarct White Red Location Organs with a single blood Organs with a dual blood supply, such as the kidney supply, such as the lung, or spleen. intestine, or testis. Main mechanism Arterial occlusion Venous occlusion (atherosclerosis, thrombosis, or embolism) Appearance Pale/ pale yellow Red/ reddish-blue Tissue Feel Dry, firm Wet, congested Damage Abrupt, severe Slow, gradual Tissue-specific infarcts Pulmonary infarcts – complication of a pulmonary embolus in the setting of CHF Difficulty providing oxygenated blood to the larger lung structures (bronchi, bronchioles) Necrosis & hemorrhage in the affected lung Already discussed intestinal infarcts in BMS 150 ▪ Consequence of blocking arterial flow or impaired venous flow (due to obstruction, strangulation volvulus) Splenic infarct ▪ Wedge-shaped, white infarcts located under the capsule Shock final common pathway for several potentially lethal clinical events ▪ severe hemorrhage or dehydration ▪ extensive trauma or burns ▪ myocardial infarction ▪ massive pulmonary embolism ▪ Sepsis and anaphylaxis Shock is a profound hemodynamic and metabolic disturbance in which the circulatory system fails to supply the microcirculation adequately, with consequent inadequate perfusion of vital organs Categories of Shock Often anaphylactic and neurogenic shock are classified as distributive shock Septic shock is kind of its own entity Shock – a useful classification system Hypovolemic Obstructive ▪ Hemorrhage, dehydration, third-spacing of fluid ▪ Cardiac tamponade, ▪ Too little fluid in vessels pulmonary embolus, Distributive pneumothorax ▪ Anaphylaxis, spinal shock ▪ Too many vessels ▪ Heart is working, dilated, not enough but blood can’t blood to keep the leave the heart pressure up Cardiogenic Septic ▪ Heart attack, heart failure, ▪ Shock is due to poor dysrhythmias ▪ Heart isn’t working blood distribution AND inflammatory damage Shock – a useful classification system Septic shock a severe and potentially life-threatening condition that occurs as a result of sepsis, which is the body's extreme response to an infection. In septic shock, the body's response to infection leads to widespread inflammation, causing significant changes in blood circulation and resulting in a dangerously low blood pressure and inadequate blood flow to vital organs. Shock - Fatal Shock is fatal primarily due to inadequate tissue perfusion leading to cellular death, multiple organ dysfunction, systemic inflammatory responses, and failure of compensatory mechanisms. The rapid progression and complexity of the condition necessitate early recognition and prompt intervention to improve survival outcomes. Septic shock – simplified pathophysiology There is no one diagnostic test for septic shock – instead a range of scoring systems are used to identify patients that are at higher risk of suffering serious outcomes In general, the following occur (more in Emerg Med): ▪ Dysregulated vascular reflexes 🡪 inappropriate vasodilation and often edema, sometimes due to damage to the endothelium ▪ Higher levels of pro-inflammatory cytokines 🡪 adverse impacts on tissues such as the heart and kidneys ▪ Movement of leukocytes into a wide range of organs 🡪 dysfunction ▪ Inappropriate activation of the coagulation and complement cascades Stages of shock – applies to all causes Stage I – compensated ▪ Tachycardia, but blood pressure is normal Can you describe the physiology behind why tachycardia makes sense, and what physiologic events cause it? Stage II – decompensated ▪ Body is no longer effectively compensating for the reduced flow to tissues ▪ Tachycardia and hypotension ▪ Much more difficult to reverse at this stage Other stages? FYI Stage III – irreversible ▪ If the situation is not corrected very quickly, death will likely ensue – very difficult to treat patients in this stage ▪ High tachycardia or bradycardia, hypotension, evidence of decreased organ function (renal failure, impaired heart function, decreased level of consciousness) ▪ This stage is not always described in texts Multi-organ dysfunction: ▪ As the name suggests, multiple organs “fail” 🡪 lose function and/or become ischemic ▪ Labile BP/hypotension, decreased LOC or coma, physiologic evidence of organ dysfunction Prognosis is poor Shock – (very) general clinical findings hypovolemic and cardiogenic shock: ▪ hypotension ▪ a weak, rapid pulse ▪ tachypnea ▪ cool, clammy, cyanotic skin septic shock: ▪ same as above, but skin may initially be warm and flushed because of peripheral vasodilation Other signs will appear that are more specific for the underlying condition Ischemic Heart Disease Definition: ▪ Supply of blood to the myocardium is inadequate for metabolic demands of the heart Blood supply is either completely blocked or reduced ▪ By far, most common cause is atherosclerosis of the coronary arteries Other causes – aneurysms, autoimmune attack or coronary vessels, strange episodes of vasospasm Epidemiology – 7 million deaths/year are due to ischemic heart disease worldwide – leading cause of death ▪ Every minute, someone in the US dies from myocardial infarction Pathogenesis - IHD Ischemic heart disease develops due to: ▪ Progressive narrowing of coronary arteries 🡪 hypoperfusion of myocardium 🡪 heart failure More on heart failure in e-learning ▪ Sudden occlusion of a major coronary artery resulting in an infarct An atherosclerotic plaque ruptures 🡪 acute clot formation 🡪 blocks the artery or gives rise to an embolus that blocks blood flow further downstream Exacerbated by any situation that increases metabolic activity of the heart ▪ Balance between nutrient/oxygen supply and cardiac metabolic demand What are factors that acutely affect the heart’s metabolic demands? Heart rate – The faster the heart rate, the more energy used Wall tension – Determined by the volume and pressure within the heart chambers – A dilated, enlarged heart uses more energy than one that is normal size – Laplace’s law Contractility – How “hard” the heart contracts at any given time – What intracellular ion? - Intracellular Ca2+ Causes of IHD By far the most common primary cause is coronary atherosclerosis ▪ Many of the other factors exacerbate oxygen delivery or cardiac oxygen consumption Pathogenesis - Influence of atherosclerosis Atherosclerosis is the major cause of ischemic heart disease (90% of cases) – When the lumen of a large coronary artery is reduced by 50 - 75%, there are usually symptoms of IHD during increased activity (i.e. stable angina) – At 80 - 90% reduction, often there are symptoms at rest (i.e. unstable angina) Often, there is more than one cause for ischemic heart disease – i.e. hypertension and a hypertrophic heart in combination with atherosclerotic coronary arteries General Pathophysiologic Concepts A healthy person has substantial coronary flow reserve and myocardial perfusion can increase to five times resting levels with intense exercise ▪ myocardial circulation is mainly controlled by constriction and dilation of small, intramyocardial branches less than 400 μm in diameter ▪ In advanced atherosclerosis of the main epicardial coronary arteries, luminal stenosis decreases blood pressure distal to the narrowed zone Maximal blood flow to the myocardium is not impaired until about 75% of the cross-sectional area of an epicardial coronary artery is compromised by atherosclerosis ▪ Resting blood flow is not reduced until >90% of the lumen is occluded Pathophysiology - Types of IHD presentation Stable angina ▪ If there is a plaque or thrombosis causing occlusion, the obstruction is thought to be stable/unchanging Acute coronary syndromes (ACS) ▪ Unstable angina Could be a thrombus that forms and is broken down constantly over a plaque Could be a very significant (limits a lot of flow, lumen is only 10-20% of regular diameter) stable occlusion Other “rare” conditions, like vasospastic angina, could be the cause ▪ Non-ST elevation myocardial infarction ▪ ST-elevation myocardial infarction Pathophysiology – unstable angina Prinzmetal angina (vasospastic or variant angina) Technically a type of unstable angina, but has a much better prognosis ▪ Caused by coronary artery spasm ▪ Occurs early morning; unrelated to exertion ▪ Does not usually cause infarction ▪ Responds well to vasodilators Nitroglycerine, calcium channel blockers ▪ Typical patient population – younger ( < 60 years) women Adaptations to chronic ischemia in the heart Hypertrophy and changes to the molecular mechanisms of contraction in cardiac myocytes (more on this later) ▪ Ischemic cardiomyopathy Development of coronary collateral circulation ▪ Extensive collateral connections develop in hearts with severe coronary atherosclerosis 🡪 provide enough arterial flow to prevent infarction completely or to limit infarct size if occlusion occurs Pathological pathways during an acute infarct In the heart, the sub-endocardial vessels are the most threatened ▪ The pressure during systole is the highest in the sub- endocardial (inner) small blood vessels, and the subendocardial muscle is the last to relax ▪ The heart ONLY receives oxygenated blood during diastole… especially closer to the interior of the chamber This microcirculatory reality explains the pathological pattern of different types of infarct ▪ Blockade of a major epicardial vessel 🡪 infarcted (dead) tissue across the whole wall ▪ Unstable plaques, partial plaques, global hypoperfusion 🡪 infarcted tissue just in the subendocardium This type of obstruction would likely result in stable angina, not an ACS Severely narrowed lumen could cause These types of obstruction angina at would likely result in rest (therefore unstable angina or an unstable) infarct (ACS) Pathophysiological development of MI The first few minutes: swelling of cells and mitochondria, loss of glycogen ▪ Reversibly injured myocytes show subtle changes of sarcoplasmic edema, mild mitochondrial swelling, and loss of glycogen ▪ “stunned myocardium” = cells that are not dead, but cannot contract After 30 to 60 minutes of ischemia myocyte injury has become irreversible ▪ mitochondria are greatly swollen and deformed ▪ Nuclei show clumping and margination of chromatin and the sarcolemma is focally disrupted ▪ Disruption of cell membranes 🡪 release of intracellular substances into the circulation ▪ Findings of coagulative necrosis Necrosis – mechanisms of injury: ▪ Depletion of ATP ▪ Mitochondrial damage ▪ Calcium accumulation ▪ Oxidative stress / free radicals ▪ Membrane damage ▪ Denatured proteins ▪ DNA damage Kumar et. al., Robbins and Cotran Pathologic Mitochondrial damage Mitochondrial membranes can be damaged by free radical attack if levels of cytosolic calcium increase too high in the cell, the MPTP can open ▪ Loss of mitochondrial membrane potential ▪ Releases H+ ▪ Further increase in cytoplasmic calcium Mitochondrial calcium “dumped” Increased into cytosol cytosolic ▪ Inability to generate ATP calcium 🡪 and ultimately necrosis MPTP ▪ This channel is poorly opens 🡪 understood.. But leakage important of H+ and calcium Kumar et. al., Robbins and Cotran Pathologic Pathophysiological development of MI Days 2 – 3: ▪ Neutrophils enter necrotic tissue - only gain access at the edge of the infarct, where blood still flows ▪ Interstitial edema and microscopic areas of hemorrhage may also appear ▪ muscle cells are more clearly necrotic, nuclei disappear and striations become less prominent Days 5 – 7: ▪ neutrophils have been replaced by macrophages, myofibroblasts begin depositing collagen (scar tissue) ▪ Dangerous period – the wall is weak Pathophysiological development of MI Week 1 and later: ▪ Collagen deposition with notable myofibroblasts and macrophages 🡪 ▪ Week 3: mostly scar tissue, new vessels and macrophages have disappeared from the infarct ▪ Later: scar becomes more “solid” as it is remodelled Pathophysiological development of MI What happens if you quickly restore blood flow, before too many myocardial cells have died? Do you “save the day”? ▪ Sort of… Reperfusion injury = damage to cardiomyocytes that occur after blood flow is restored to ischemic tissue ▪ Many myocardial cells will undergo contraction band necrosis when blood flow is restored Contraction band = hypercontracted and disorganized sarcomeres with thickened Z disks. Happens when calcium floods in across disrupted sarcolemma and ROS are generated by damaged mitochondria that suddenly have access to oxygen Can lose some myocardial cells even after blockage is removed (but still advantageous to restore blood flow) Seems that inflammatory damage contributes to reperfusion injury as well Transmural vs. non-transmural infarcts Transmural infarcts ▪ Large, “permanent” occlusion of a coronary artery ▪ Thought to be same entity as an ST-elevation infarct (STEMI) Non-transmural ▪ Can have a variety of pathological patterns ▪ Global hypoxia, many small vessels occluded, transient occlusion ▪ Thought to be the same entity as a non-ST-elevation infarct (NSTEMI) MI – Typical Vascular Patterns Which vessels are most often involved? – Anterior descending branch of left coronary artery (50%) – apical, anterior, and anteroseptal wall left ventricle infarcts – Right coronary artery (30-40%) – infarct of the posterior basal left ventricle and the posterior 1/3 to 1/2 of the interventricular septum (“inferior” infarct) – Left circumflex artery (15-20%) – lateral left ventricle wall. Vascular territories in IHD IHD – clinical manifestations Asymptomatic Pain – chest pain = angina pectoris – Different patterns of chest pain – referred to left arm/shoulder, retrosternal, interscapular, can also appear similar to gastro-esophageal reflux – Pain is typically “crushing” or squeezing in character – rarely described as sharp Dyspnea, Fatigue Palpitations Diaphoresis Congestive heart failure symptoms (more later) IHD – Clinical Manifestations How does it usually present? ▪ Acutely: Stable angina Unstable angina Myocardial infarction (two types, ST elevation and non-ST elevation infarcts) Sudden cardiac death ▪ Chronically: Heart failure ▪ Particular type of heart failure sometimes referred to as ischemic cardiomyopathy Ischemic Heart Disease – clinical features Stable angina: ▪ Chest or arm pain (or discomfort) ▪ Reproducibly associated with physical exertion or stress Pattern is consistent with previous episodes ▪ Relieved in a short time period (3 – 20 min) by: Rest Nitroglycerine Ischemic Heart Disease – clinical features Unstable angina – any of: ▪ New onset chest pain/discomfort, or presents differently than before ▪ Occurs at rest or not relieved by nitroglycerine/rest ▪ Is severe, and/or pain is accelerating in intensity (crescendo pattern) Myocardial infarction: ▪ Ischemic heart disease resulting in the death of heart muscle ▪ Type of infarct is determined by ECG and/or pathologic criteria Unstable angina is much more likely to be associated with an infarct than stable angina Together, unstable angina and myocardial infarction are known as acute coronary syndromes (ACS) Ischemic Heart Disease – clinical features Sudden cardiac death ▪ Somewhat poorly named – people can be resuscitated from it and can survive “death” ▪ Criteria are not exact – however, it involves: No acute infarcts A dysrhythmia with sudden onset that is due to long-term coronary ischemia The dysrhythmia is fatal unless aggressive resuscitation measures are undertaken ▪ 90% of the time sudden cardiac death is a complication of long-term IHD Although it is due to long-term coronary vascular disease, it presents suddenly and catastrophically with patient collapse IHD – clinical features Often the pain of an MI is more severe than stable, unstable angina ▪ Many exceptions to this rule, though MIs can masquerade as heartburn Atypical presentations during an MI are not uncommon ▪ Interscapular pain ▪ Discomfort vs. pain ▪ Sometimes dyspnea, acute severe fatigue is the main complaint IHD - Diagnosis ECG – what patterns would you see? ▪ More discussion in dysrhythmias ▪ Can be used for general IHD and for MI Cardiac enzymes ▪ See next slide ▪ troponin (T and I), CK-MB most reliable, specific markers Angiogram Echocardiogram Nuclear medicine imaging ▪ Certain isotopes are taken up more avidly by damaged/ischemic cardiac tissue IHD - general treatment Treat causes of imbalance between energy supply and demand to the myocardium ▪ ASA – antiplatelet agent, reduces the risk of a life-threatening thrombus developing Prevents existing thrombi from enlarging a number of other anti-platelet agents exist ▪ Antihypertensives (especially ACE inhibitors) ▪ Beta blockers ▪ Calcium channel blockers Reduces contractility, some vasodilate a little ▪ Nitroglycerine Decreases preload, coronary vasodilator, decreases afterload ▪ Good blood glucose and serum lipid control ▪ Percutaneous coronary intervention (PCI) or coronary artery bypass grafting (CABG) in certain cases Most with stable angina don’t benefit that much from stenting (no survival benefit, does not prevent future MI) so done on a case-by-case basis MI - treatment – NSTEMI – no “clot-busting” drugs like tissue plasminogen activator – STEMI – “clot-busting” drugs can be life-saving – Thrombolytic drugs end in –plase (reteplase, alteplase, used to use streptokinase) – More when we learn about the coagulation cascade – For both, revascularization (angioplasty or putting in a stent) is an effective treatment modality – However, revascularization requires access to a team experienced with the treatment Resuscitation must be immediate or death often occurs when dysrhythmias develop Chronic medications same as those for stable, unstable angina IHD - complications Myocardial infarction: ▪ Death occurs acutely in 25% - 35% of cases due to dysrhythmia (ventricular fibrillation), heart block, heart failure, asystole (cardiac arrest) ▪ Also a high mortality days – months post-infarct Life-threatening dysrhythmias Cardiac rupture – highest risk at 3 – 7 days post-MI due to coagulative 🡪 liquiefactive necrosis in the wall of the myocardium or at the attachment point of a papillary muscle Poor wall motion 🡪 clot development (more later) Pericarditis BMS 200 – Cardiology 6 Heart Failure, Cardiomyopathies, and Valvular Heart Disease Outcomes Describe the general pathological findings and pathophysiologic consequences of: Valvular stenosis, valvular regurgitation, valvular prolapse Relate the pathophysiology of common valvular diseases to findings on the physical exam Describe the basic epidemiology, pathogenesis, clinical features, and prognosis of the following valvular heart disorders: Aortic regurgitation, Calcific aortic stenosis, Bicuspid aortic valves Mitral valve prolapse, Mitral regurgitation, Rheumatic heart disease Describe the basic epidemiology, pathogenesis, clinical features, and prognosis of the following cardiomyopathies: Hypertrophic cardiomyopathy, Dilated cardiomyopathy (hereditary) Restrictive cardiomyopathy CAD-related ventricular failure, Hypertensive heart disease Outcomes Relate the cellular and gross pathophysiologic adaptations in heart failure to underlying etiologic and risk factors Compare the pathogenesis, clinical features, diagnostic findings, and complications of left-sided and right-sided congestive heart failure Compare the pathogenesis, clinical features, diagnostic findings, and complications of HFpEF and HFrEF Briefly describe the pharmacologic mechanism of action and related adverse effects of common medications used to treat heart failure Heart Failure – an Introduction A normal ventricle should be: ▪ Compliant – diastolic filling occurs at low atrial pressures, and the atria do not have to undergo hypertrophy to fill the ventricle at the end of diastole The ventricle should be able to relax quickly and most filling should take place in early diastole ▪ “Strong” – a ventricle should generate enough force at rest with low diastolic pressures/preload to meet the needs of the body Calcium should be quickly released and re- sequestered each cycle There should be a significant reserve of function for when activity increases Heart Failure – an Introduction Heart failure can occur for many reasons: ▪ Increased afterload (overload) of the ventricles over long periods of time Ventricles become hypertrophic, increasing wall thickness and eventually chamber size Molecular changes 🡪 decreased contractility ▪ Impaired oxygen supply in a setting of chronic ischemic heart disease May or may not involve sites of infarcted tissue ▪ Impaired ability to relax (reduced compliance) due to fibrosis or poorly-characterized molecular changes ▪ Disorders that damage the myocardium and impair compliance or contractility Known as cardiomyopathies Heart Failure – an Introduction Prevalence of heart failure U.S. adults ≥20 years of age by sex and age from the National Health and Nutrition Examination Survey (NHANES), 2013– 2016 Biggest underlying etiologies/risk factors for heart failure Note – kind of weird stats here, don’t quite add up to 100% Atherosclerosis alone (not shown here) is responsible for about 2/3 Heart Failure – P-V loops The pathogenesis of heart failure is still being clarified, though it’s clear that there are two major phenotypes: ▪ Systolic dysfunction (A) – impaired force of contraction/contractility 🡪 reliance on elevated preload for adequate cardiac output ▪ Diastolic dysfunction (B) – elevated diastolic pressures are evident, but force of contraction/contractility is maintained Despite elevated diastolic pressures, there may be impaired EDV Heart Failure – P-V loops The pathogenesis of heart failure is still being clarified, though it’s clear that there are two major phenotypes: ▪ Systolic dysfunction (A) is mostly referred to in the literature as heart failure with reduced ejection fraction (HFrEF) ▪ Diastolic dysfunction (B) – is mostly referred to in the literature as heart failure with preserved ejection fraction (HFpEF) although in the diagram below the EF is reduced slightly from normal Pathophysiologic Pathways in CHF As heart failure progresses, two major problems arise: “Forward flow” problems – impaired cardiac output to a range of tissues impairs function ▪ Major tissues that experience decreased perfusion include the brain, the heart, the kidneys, and the extremities Sometimes reduced flow to the viscera can lead to abdominal pain, but uncommon ▪ Impaired venous return from the pulmonary veins 🡪 LV Pathophysiologic Pathways in CHF “Backwards” problems – congestion ▪ As LV CO declines, blood congests in the pulmonary venous circulation 🡪 elevated pressures in pulmonary capillaries 🡪 development of pulmonary edema and thickening of arterioles/arteries in the lung ▪ As RV CO declines, blood congests in the systemic venous circulation 🡪 elevated pressures in systemic capillaries 🡪 edema Hepatic congestion & splenomegaly Dependent edema Pathophysiologic Pathways in CHF Since the left ventricle experiences the greatest afterload, it is usually the “first to fail” ▪ As pulmonary congestion increases, the afterload of the RV also increases 🡪 development of RV failure There are situations where the right ventricle is the “first to fail” ▪ Lung disease 🡪 areas that are Cor pulmonale. hypoxic/poorly ventilated 🡪 heart from a patient with pulmonary vasoconstriction ▪ Known as cor pulmonale – pulmonary hypertension common causes include COPD and markedly hypertrophied obstructive sleep apnea right ventricle RV free wall thickness nearly equals the left Comparison of Microcirculation - Review Pulmonary Microcirculation Controlled by O concentrations 2 ▪ constriction in response to decreased oxygen levels ▪ how does this compare to other vascular beds? Why does this make sense? Pathophysiologic Pathways in CHF In the end, both ventricles suffer dysfunction in heart failure and there is some reduction in both compliance (diastolic dysfunction) and force of contraction (systolic dysfunction) ▪ However, most cases of heart failure can be clearly dichotomized into HFrEF and HFpEF The heart is typically hypertrophied in two common patterns that differ from physiologic, or “healthy” hypertrophy that is common in athletes ▪ Concentric hypertrophy ▪ Eccentric hypertropy Pathophysiologic Pathways in CHF Concentric hypertrophy ▪ Thought to be earlier in the development of HF ▪ Thickened ventricular wall, no increase in chamber size ▪ Increased thickness is thought to minimize wall stress Eccentric hypertrophy ▪ Ongoing remodeling 🡪 eccentric hypertrophy as myocytes increase in length ▪ Usually associated with a decrease in ejection fraction and increased symptoms Pathophysiologic Pathways in CHF Molecular adaptations in the failing heart: The structure of myocytes and surrounding tissue is altered over time – known as ventricular remodelling ▪ Increased expression of fetal forms of myosin that use ATP more effectively but generate less force ▪ Increased expression of TGF-beta leads to deposition of extracellular matrix in the extracellular spaces ▪ Myocytes themselves enlarge, but the capillary network in the hypertrophic heart tends to be less extensive than in physiologic hypertrophy Pathophysiologic Pathways in CHF Signaling pathways implicated in HF: Angiotensin II – as cardiac output to the kidneys decreases 🡪 increased AT II ▪ AT II can also be released by “stressed” cardiac cells AT II can directly bind to myocyte and myofibroblast receptors 🡪 hypertrophy, proliferation of myofibroblasts, and increased deposition of connective tissue Increased AT II also increases volume and vasoconstriction 🡪 worsened edema and afterload Pathophysiologic Pathways in CHF Signaling pathways implicated in HF: Beta-adrenergic signaling is increased in early heart failure 🡪 receptor downregulation Long-term beta-adrenergic signaling also results in hypertrophy and fibrosis, and even eventually apoptosis of myocytes ▪ long-term activation of the SNS in the heart is maladaptive, even though short-term activation improves cardiac function ▪ Exact signaling mechanisms are currently being studied Pathophysiologic Pathways in CHF Signaling pathways implicated in HF: Other detrimental pathways: ▪ Endothelin-1: potent vasoconstrictor that is also a growth factor for cardiomyocytes ▪ Inflammatory cytokines: activate JNK and MAPK pathways that seem to be linked to maladaptive remodeling and apoptosis Pathophysiologic Pathways in CHF Signaling pathways implicated in HF: Calcium homeostasis in the failing heart ▪ Ryanodine receptors release less calcium per AP ▪ SERCA calcium uptake is inhibited ▪ The net effect seems to be elevated diastolic calcium levels and impaired calcium spikes during contraction Beneficial pathways: ▪ Activation of IGF-1 and PI3K pathways seem to be the major routes that drive physiologic (healthy) hypertrophy CHF Pathophysiology & Neurohormonal Activation Activation of the SNS and RAAS initially leads to: ▪ Increased HR, BP, contractility ▪ Retention of sodium and water Increases preload and cardiac output Over time, things get out of hand ▪ Excessive vasoconstriction and volume retention Baroreceptor dysfunction that permits elevated pressures and decreases PNS tone ▪ Increased ADH release 🡪 increased volume ▪ Excessive SNS activation 🡪 decreased renal perfusion… which leads to chronically elevated release of renin + AT2 to maintain blood flow to the kidney RAAS - Review This model applies to renal release of renin & AT2 from the kidney ▪ Does not apply to release of AT2 from cardiomyocytes Renin release can be caused by decreased perfusion AND activation of the SNS Protection against fluid overload Both atrial (ANP) and B-type natriuretic peptide (BNP) have similar functions ▪ BNP is released by “stretched” ventricles Over time the heart failure patient seems to become resistant to BNP and ANP ▪ no longer leads to sodium and water loss ▪ FYI – some medications (known as neprilysin inhibitors) help reduce this resistance Heart Failure – General Clinical Features Symptoms ▪ Fatigue is universal ▪ “Left-sided” symptoms: orthopnea, dyspnea, angina, impaired cognitive function Can cause chronic kidney disease (CKD) and exacerbate ischemic heart disease ▪ “Right-sided” symptoms: dependent edema, RUQ pain Signs ▪ Pitting edema, hepatosplenomegaly Hepatojugular reflux is a poorly-characterized sign – alternatives include blood pressure measurement during Valsalva ▪ Elevated JVP, displacement of the apical impulse, S3 or S4 ▪ Crackles, wheezing, and sometimes pleural effusions depending on the extent of pulmonary edema New York Heart Association Classification Functional Limitation Clinical Assessment Class Commonly-used clinical staging tool Class I None Ordinary physical activity does not cause undue fatigue, dyspnea, palpitations, or Doesn’t always angina correlate well to Class II Mild Comfortable at rest. Ordinary physical activity objective (e.g., carrying heavy packages) may result in fatigue, dyspnea, palpitations, or angina measurements (i.e. EF) Class III Marked Comfortable at rest. Less than ordinary physical activity (e.g., getting dressed) leads How one judges to symptoms symptom severity/quality Class IV Severe Symptoms of heart failure or angina are present at rest and worsened with any activity of life Heart Failure - Diagnosis HF is usually diagnosed with echocardiography ▪ HFrEF – decreased ejection fraction Normal EF is > 50%, HFrEF is diagnosed when EF < 40% ▪ HFpEF – normal (>50%) EF but: Left ventricular hypertrophy Atrial enlargement, abnormal ventricular wall movement More challenging diagnosis than HFrEF BNP – a natriuretic factor that is released by the ventricle in response to increased strain ▪ Can be helpful to diagnose both chronic HF and acute exacerbations of CHF Chest X-ray can help identify cardiomegaly and pulmonary edema ▪ Not as specific or sensitive as echocardiography, though Chronic IHD 🡪 CHF Chronic IHD (coronary artery disease) is the most common cause of heart failure (60% of CHF) ▪ the majority of the rest of the cases are due to hypertension (2 most nd common), valvular abnormalities, or patients with congenital heart disease Clinical Features of CHF due to IHD: ▪ Myocardial hypertrophy and fibrosis are present as with most etiologies of CHF May see “scars” caused by healed old infarcts ▪ Although there is both systolic and diastolic function, usually presents as HFrEF – the left ventricle is usually the first to be involved Review - atherosclerosis Progression from fatty streak 🡪 deposition of oxidized LDL 🡪 migration and activation of macrophages 🡪 ▪ Calcification, accumulation of cholesterol, foam cell development ▪ Increased deposition of extracellular matrix under the intima ▪ A variably-stable fibrous cap with underlying necrotic tissue and immune cells ▪ Stenosis of the lumen and impaired blood flow Review – risk factors and the development of atherosclerosis Smoking, high blood pressure, oxidative stress increase endothelial damage Lp(a) – likely increases endothelial damage through increasing immune cell recruitment at a developing plaque ▪ May also inhibit breakdown of clots Diabetes and dyslipidemia (including metabolic syndrome): ▪ Diabetes – LDL is more likely to be incorporated into the intima in the setting of AGEs in the endothelium – likely site of oxidation of LDL ▪ AGEs can also increase general inflammation, leading to increased oxidative stress ▪ Increased LDL 🡪 increased oxidized LDL 🡪 deposition in fatty streaks 🡪 activation of macrophages (via the scavenger receptor) Chronic hypertension 🡪 CHF As mentioned above, long-term hypertension can cause CHF (second most common cause of CHF) ▪ Typically see concentric LV hypertrophy – wall thickens, but the chamber size does not tend to increase Over time can proceed to eccentric hypertrophy ▪ Microscopy shows hypertrophied cardiomyocytes ▪ As with many causes of heart failure, often hypertrophy does not result in increased capillary density Heart failure is one of the leading causes of death due to hypertension Medications for CHF and/or angina Beta-blockers – used for angina and CHF ▪ Most beta-blockers used in IHD or CHF are relatively selective for the beta-1 epi/norepinephrine receptor ▪ IHD: Reduce cardiac oxygen demand – thus effective prophylactic for angina, other complications of IHD ▪ CHF: reduces and even reverses cardiac remodeling (less fibrosis, hypertrophy, cell death) Cardiac glycosides (digoxin) – used in CHF ▪ Cardiac glycosides inhibit the sodium-potassium pump You would think that would be a bad idea – but see next slide ▪ Increase contractility by increasing intracellular calcium, but also increase vagal tone (resulting in a slower heart rate) Increasing vagal tone helps decrease oxygen demand Mechanism of Action - digoxin Calcium extrusion from the cytosol is partially determined by the sodium gradient Sodium-calcium exchanger As the sodium gradient is decreased with digoxin, so is the activity of the sodium-calcium exchanger Results in a somewhat increased cytosolic calcium concentration during systole Medications for CHF Diuretics – used for CHF ▪ In general, diuretics reduce blood volume, usually by increasing water and sodium loss at the kidney tubule Loop and thiazide diuretics – inhibit sodium reabsorption by inhibiting particular sodium transporters earlier in the nephron Spironolactone – blocks the aldosterone receptor 🡪 loss of sodium and water in the distal nephron ACE inhibitors (ACE-i) block angiotensin-converting enzyme that converts AT1 to AT2 ▪ They likely also have beneficial impacts on heart remodeling, just like beta-blockers FYI – CHF strategies CHF doesn’t occur in isolation ▪ IHD, hypertension are the major causes and both are linked to diabetes Prevention of these causes ▪ Early adoption of medications that positively impact remodelling Medications for angina Calcium channel blockers Can cause vasodilation with limited impact cardiac conduction or contractility ▪ Drugs in this class are known as dihydropyridine (DHP) calcium channel blockers (FYI example – amlodipine and nifedipine) Can cause slowing of AV conduction (slows heart rate) and decreased contractility, but with variable effects on vasodilation ▪ These medications are known as nondihydropyridine calcium channel blockers FYI – verapamil is the prototype medication here - diltiazem decreases heart rate and causes vasodilation Nitrates – converted to nitric oxide 🡪 Decreased preload (through vasodilation of veins, decreased venous return) 🡪 decreased oxygen demand Decreased afterload (through vasodilation of arterioles) 🡪 decreased oxygen demand Coronary vasodilation 🡪 increased blood supply Dyslipidemia medications – basic MOAs HMG CoA-reductase inhibitors (statins) – reduce the hepatocyte’s ability to produce cholesterol 🡪 depletion of the hepatocyte’s “intracellular supply” of cholesterol 🡪 upregulation of the LDL receptor on the hepatocyte cell membrane ▪ This increases clearance of LDL from the circulation ▪ These medications also: Decrease circulating triglyceride levels Improve endothelial function Seem to also reduce oxidative stress and inflammation at the plaque PCSK9 inhibitors block a protease known as PCSK9 on the hepatocyte membrane ▪ This protease degrades the LDL receptor – if it is blocked, then there are more LDL receptors available to clear LDL Dyslipidemia medications – basic MOAs Ezetimibe – reduces the absorption of dietary and biliary cholesterol in the small intestine ▪ This leads to a decrease in cholesterol stores in the hepatocyte 🡪 increased LDL receptor expression Niacin (not used as often now) - inhibits lipolysis in adipose tissue ▪ Therefore less release of FFAs 🡪 less production of VLDL by the liver ▪ This in turn leads to decreased circulating LDL, but main effect is decreased in TG (VLDL) synthesis ▪ Also increases HDL… however importance of this is not known Valvular Pathology - Generalities Valves are lined by endocardium with underlying dense irregular connective tissue, connected to the fibrous rings Pathological processes that damage valves: ▪ Congenital disorders – malformation of structures (improper migration, other causes) Examples – pulmonic stenosis and bicuspid aortic valves ▪ “Wear and tear” chronic damage – often a chronic inflammatory/calcific process on top of increased physical stresses Examples - Aortic stenosis and sclerosis, mitral valve calcification ▪ Inflammatory (due to infection, autoimmunity) Examples - Bacterial endocarditis, Rheumatic heart disease, lupus, ankylosing spondylitis ▪ Acute impairments in valvular function Ischemia 🡪 impaired papillary muscle function 🡪 mitral regurgitation Aortic dissection 🡪 widening of aortic root 🡪 regurgitation ▪ Idiopathic - Mitral valve prolapse Review – stenosis, prolapse, incompetence, regurgitation Pulmon Stenosis – the valve has a more narrow than normal ic orifice, and/or it is difficult to open Stenosi ▪ Either way 🡪 increased strain across the wall of the heart s proximal to the stenosis🡪 hypertrophy and complications ▪ Can also initially result in impaired outflow to structures after the stenosis 🡪 physiologic adaptations to poor outflow Regurgitation – backflow of blood across a valve ▪ Backflow of blood to chamber proximal to the regurgitation 🡪 BOTH increased EDV/preload + impaired outflow distal to the regurgitation 🡪 eventual chamber enlargement ▪ Incompetence (insufficiency) = the valve does not close completely ▪ Prolapse = excessive (backwards) valve movement into the proximal chamber Mitral Valve Prolapse Selected Valvular Pathologies - Today Aortic regurgitation Bicuspid and calcific aortic stenosis Mitral valve prolapse and mitral regurgitation Rheumatic heart disease Selected Valvular Pathologies - Today What do you need to know about these valvular pathologies? Roughly how common are they in relation Aortic regurgitation to each other? How are they caused? What sorts of symptoms are most likely? Bicuspid and calcific What will