Week 8-The Central Nervous System PDF
Document Details
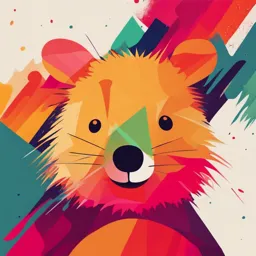
Uploaded by PrivilegedRubellite7992
Tags
Summary
This document is about the central nervous system. It covers learning outcomes, development and the regions of the CNS. Includes information on brain regions and anatomy.
Full Transcript
The Central Nervous System The learning outcome: • • • • • • • • • • • • • Describe how space constraints affect brain development. Name t he major regions of t he adult brain. Name and locate the ventricles of the brain. Brain Regions and Organization List the major lobes, fissures, and function...
The Central Nervous System The learning outcome: • • • • • • • • • • • • • Describe how space constraints affect brain development. Name t he major regions of t he adult brain. Name and locate the ventricles of the brain. Brain Regions and Organization List the major lobes, fissures, and functional areas of t he cerebral cortex Explain lateralization of cortical function Differentiate between commissures, association fibers, and projection fibers Describe the general function of the basal nuclei (basal ganglia). Identify the three major regions of t he brain stem, and note the functions of each area. Describe the structure and function of the cerebellum. Describe how meninges, cerebrospinal fluid, and t he blood brain barrier protect the CNS. Explain how cerebrospinal fluid is formed and describe its circulatory pathway. Describe the gross and microscopic structure of the spinal cord. Folding during development determines the complex structure of the adult brain • The brain and spinal cord begin as an embryonic structure called the neural tube forms, its anterior (rostral) end begins to expand and constrictions appear that mark off the three primary brain vesicles: 1. Prosencephalon or forebrain 2. Mesencephalon or midbrain 3. Rhombencephalon or hindbrain Folding during development determines the complex structure of the adult brain Brain Regions and Organization The term embryonic scheme, we will consider the brain in terms of the medical scheme and the four adult brain regions: (1) Cerebral Hemispheres, (2) Diencephalon, (3) Brain Stem (midbrain, pons, and medulla oblongata), and (4) Cerebellum. A slice of brain tissue reveals patterns of darker and lighter areas called gray matter and white matter: • Gray Matter consists of short, nonmyelinated neurons and neuron cell bodies. • White Matter consists mostly of myelinated axons with some nonmyelinated axons, primarily in fiber tracts. The dense coating of fatty myelin is what gives white matter its color. • Gray and white matter have a unique distribution in the brain. The basic pattern of the CNS is a central cavity surrounded by gray matter, external to which is white matter. The basic pattern of the CNS is a central cavity surrounded by gray matter, external to which is white matter. Ventricles • The brain ventricles are continuous with one another and with the central canal of the spinal cord. • The hollow ventricular chambers are filled with Cerebrospinal Fluid and lined by ependymal cells. • The paired Lateral Ventricles, one deep within each cerebral hemisphere, are large C-shaped chambers that reflect the pattern of cerebral growth. • Anteriorly, the lateral ventricles lie close together, separated only by a thin Median Membrane Called The Septum Pellucidum. • Each lateral ventricle communicates with the narrow Third Ventricle in the diencephalon via a channel called an Inter Ventricular Foramen. • The third ventricle is continuous with the Fourth Ventricle via the canal-like Cerebral Aqueduct that runs through the midbrain. • The fourth ventricle lies in the hindbrain dorsal to the pons and superior medulla. It is continuous with the Central Canal of the spinal cord inferiorly. • Three openings mark the walls of the fourth ventricle: the paired Lateral Apertures in its side walls and the Median Aperture in its roof. • Connect the ventricles to The Subarachnoid Space, a fluid-filled space surrounding the brain. The Brain Ventricles The cerebral hemispheres consist of cortex, white matter, and the basal nuclei • The cerebral hemispheres form the superior part of the brain and are the most visible parts of an intact brain. Together they account for about 83% of total brain mass. • Picture how a mushroom cap covers the top of its stalk, and you have a good idea of how the paired cerebral hemispheres cover and obscure the diencephalon and the top of the brain stem. • Elevated ridges of tissue called Gyri separated by shallow grooves called Sulci "furrows"-mark nearly the entire surface of the cerebral hemispheres. • Deeper grooves, called Fissures, separate large regions of the brain: • The median longitudinal fissure separates the cerebral hemispheres. • The transverse cerebral fissure separates the cerebral hemispheres from the cerebellum below. The more prominent gyri and sulci are also important anatomical landmarks that are similar in all humans. • Several sulci divide each hemisphere into five Lobes-frontal, Parietal, Temporal, Occipital, and Insula. • All but the last are named for the cranial bones that overlie them. The cerebral hemispheres consist of cortex, white matter, and the basal nuclei The cerebral hemispheres consist of cortex, white matter, and the basal nuclei • The central sulcus, which lies in the frontal plane, separates the frontal lobe from the parietal lobe. • Bordering the central sulcus are the precentral gyrus anteriorly and the postcentral gyrus posteriorly. • The parieto-occipital sulcus, located more posteriorly on the medial surface of the hemisphere, separates the occipital lobe from the parietal lobe. • The deep lateral sulcus outlines the flap like temporal lobe and separates it from the parietal and frontal lobes. • A fifth lobe of the cerebral hemisphere, the Insula, is buried deep within the lateral sulcus and forms part of its floor. The insula is covered by portions of the temporal, parietal, and frontal lobes. The cerebral hemispheres consist of cortex, white matter, and the basal nuclei • The cerebral hemispheres fit snugly in the skull. The frontal lobes lie in the anterior cranial fossa, and the anterior parts of the temporal lobes fill the Middle cranial fossa • The posterior cranial fossa, however, houses the brain stem and cerebellum. The occipital lobes are located well superior to that cranial fossa. Each of the cerebral hemispheres has three basic regions: 1. A superficial cerebral cortex of gray matter, which looks gray in fresh brain tissue 2. Internal white matter 3. Basal nuclei, islands of gray matter situated deep within the white matter Cerebral Cortex • The cerebral cortex is the "Executive Suite" of the nervous system, where our conscious mind is found. • It enables us to be aware of ourselves and our sensations, to communicate, remember, understand, and initiate voluntary movements. • The cerebral cortex is composed of gray matter: neuron cell bodies, dendrites, associated glia and blood vessels, but no fiber tracts. • It contains billions of neurons arranged in six layers. Although only 2-4 mm (about 1/8 inch) thick, it accounts for roughly 40% of total brain mass. • Modern imaging techniques allow us to see the brain in action-PET scans show maximal metabolic activity in the brain, and functional MRI scans reveal blood flow. • They show that specific motor and sensory functions are localized in discrete cortical areas called Domains. • However, many higher mental functions, such as memory and language, appear to be spread over large areas of the cortex in Overlapping Domains. Functional and structural areas of the Cerebral Cortex Functional and structural areas of the Cerebral Cortex The body maps in the primary motor cortex and somatosensory cortex of the Cerebrum Multimodal Association Areas • The association areas that have all been tightly tied to one kind of primary motor or sensory cortex. • Most of the cortex, though, consists of complexly connected multimodal association areas that receive inputs from multiple senses and send outputs to multiple areas. In general, information flows as follows: sensory receptors primary sensory cortex sensory association cortex multimodal association cortex The multimodal association areas can be broadly divided into three parts: 1. The Anterior Association. 2. Posterior Association. 3. Limbic Association Areas. Anterior Association Area: • • • • Also called the prefrontal cortex, is the most complicated cortical region of all. It is involved with intellect, complex learning abilities (called cognition), recall, and personality. It contains working memory, which is necessary for abstract ideas, judgment, reasoning, persistence, and planning. These abilities develop slowly in children, which implies that the prefrontal cortex matures slowly and depends heavily on feedback from our social environment. The multimodal association areas can be broadly divided into three parts: The posterior association area • Is a large region encompassing parts of the temporal, parietal, and occipital lobes. • This area plays a role in recognizing patterns and faces, localizing us and our surroundings in space, and binding different sensory inputs into a coherent whole. • Attention to an area of space or an area of one's own body is also a function of this part of the brain. • Many parts of the posterior association area are also involved in understanding written and spoken language. Limbic Association Area • includes the cingulate gyrus, parahippocampal gyrus, and hippocampus. • The limbic association area provides the emotional impact that makes a scene important to us. • hippocampus establishes memories that allow us to remember this incident. Lateralization of Cortical Functioning • Both cerebral hemispheres for almost every activity, and the hemispheres appear nearly identical. Nonetheless, there is a division of labor, and each hemisphere has abilities not completely shared by its partner. This phenomenon is called Lateralization. • Although one cerebral hemisphere or the other "Dominates" each task, the term cerebral dominance designates the hemisphere that is dominant for language. • In most people (about 90% ), the left hemisphere has greater control over language abilities, math, and logic. • The other hemisphere (usually the right) is more free-spirited, more involved in visual-spatial skills, intuition, emotion, and artistic and musical skills. • Most individuals with left cerebral dominance are right-handed. In the remaining 10% of people, the roles of the hemispheres are reversed or the hemispheres share their functions equally. • Typically, right-cerebral-dominant people are left-handed. Some "lefties" who have a cerebral cortex that functions bilaterally are Ambidextrous. • The two cerebral hemispheres have almost instantaneous communication with each other via connecting fiber tracts, as well as complete functional integration. • And while each hemisphere is better than the other at certain functions, Neither Hemisphere Is Better At Everything. Cerebral White Matter • The second of the three basic regions of each cerebral hemisphere is the internal cerebral white matter. • The white matter deep to the cortical gray matter is responsible for communication between cerebral areas and between the cerebral cortex and lower CNS centers. • White matter consists largely of myelinated fibers bundled into large tracts. These fibers and tracts are classified according to the direction in which they run as association, commissural, or projection • Association fibers connect different parts of the same hemisphere. Short association fibers connect adjacent gyri. Long association fibers are bundled into tracts and connect different cortical lobes. • Commissural fibers connect corresponding gray areas of the two hemispheres. Allow the two hemispheres to function as a coordinated whole. The largest commissure is the Corpus Callosum, which lies superior to the lateral ventricles, deep within the longitudinal fissure. Less prominent examples are the anterior and posterior commissures • Projection fibers either enter the cerebral cortex from lower brain or cord centers or descend from the cortex to lower areas. Sensory information reaches the cerebral cortex and motor output leaves it through these projection fibers. They tie the cortex to the rest of the nervous system and to the body's receptors and effectors. The projection fibers run vertically. At the top of the brainstem, the projection fibers on each side form a compact band, the internal capsule, that passes between the thalamus and some of the basal nuclei. Superior to that point, the fibers radiate fanlike through the cerebral white matter to the cortex. This distinctive arrangement is called the Corona Radiate. Cerebral White Matter White fiber tracts of the cerebral hemispheres. Commissural, association, and projection fibers run within the cerebrum, and between the cerebrum and lower CNS centers Basal Nuclei • Deep within the cerebral white matter is the third basic region of each hemisphere, a group of subcortical nuclei called the basal nuclei or basal ganglia. • They are primarily involved in the control of movement. Although the definition of the precise structures forming the basal nuclei is controversial, most anatomists agree that each hemisphere's basal nuclei include the caudate nucleus, putamen, and globus pallidus. • The comma-shaped caudate nucleus arches superiorly over the diencephalon. Together with the putamen, it forms The Striatum so called because the fibers of the internal capsule passing through them create a striped appearance. Although the putamen ("pod") and globus pallidus ("pale globe") together form a lens-shaped mass, sometimes called the Lentiform Nucleus, these two nuclei are functionally separate. • The basal nuclei are functionally associated with the Subthalamic Nuclei (located in the lateral "floor" of the diencephalon) and the substantia nigra of the midbrain. The basal nuclei receive input from the entire cerebral cortex, as well as from other subcortical nuclei and each other. Via Relays through the thalamus, the output nucleus of the basal nuclei (globus pallidus) and the substantia nigra project to the premotor and prefrontal cortices and so Influence Muscle Movements Directed By The Primary Motor Cortex. Basal Nuclei • The basal nuclei have no direct access to motor pathways. The precise role of the basal nuclei has been elusive because of their inaccessible location and because their motor functions overlap with those of the cerebellum. • The basal nuclei play a role in cognition and emotion. In all of these cases, the basal nuclei seem to filter out incorrect or inappropriate responses, passing only the best response on to the cortex. • For example, in motor activity, the basal nuclei are particularly important in starting, stopping, and monitoring the intensity of movements executed by the cortex, especially those that are relatively slow or stereotyped, such as arm-swinging during walking. • Additionally, they inhibit antagonistic or unnecessary movements. Disorders of the basal nuclei include Huntington's disease and Parkinson's disease. Basal Nuclei (a) Three-dimensional view of the basal nuclei (basal ganglia), showing their position in the cerebrum. (b) Transverse section of cerebrum and diencephalon showing the relationship of the basal nuclei to the thalamus and the lateral and third ventricles. The Diencephalon • Includes largely of three paired: Thalamus, Hypothalamus, and Epithalamus. These gray matter areas enclose the third ventricle • Forming the central core of the forebrain and surrounded by the cerebral hemispheres, Thalamus • Consists of bilateral egg-shaped nuclei, which form the superolateral walls of the third ventricle. • Thalamus is a Greek word meaning "inner room," which well describes this deep, well-hidden brain region that makes up 80% of the diencephalon. • Within the thalamus are a large number of nuclei, most named according to their location. Each nucleus has a functional specialty, and each projects fibers to and receives fibers from a specific region of the cerebral cortex. • Afferent impulses from all senses and all parts of the body meet on the thalamus and synapse with at least one of its nuclei. For example, the ventral posterolateral nuclei receive impulses from the general somatic sensory receptors (touch, pressure, pain, etc.), and the lateral and medial geniculate bodies "knee shaped" are important visual and auditory relay centers, respectively. • Within the thalamus, information is sorted out and "edited." Impulses having to do with similar functions are relayed as a group via the internal capsule to the appropriate area of the sensory cortex and to specific cortical association areas. • As the afferent impulses reach the thalamus, we have a crude recognition of the sensation as pleasant or unpleasant. However, specific stimulus localization and discrimination occur in the cerebral cortex. Thalamus • In addition to sensory inputs, virtually all other inputs ascending to the cerebral cortex funnel through thalamic nuclei. These include: • Inputs that help regulate emotion and visceral function from the hypothalamus (via the anterior nuclei) • Instructions that help direct the activity of the motor cortices from the cerebellum and basal nuclei (via the ventral lateral and ventral anterior nuclei, respectively) • Inputs for memory or sensory integration that are projected to specific association cortices • In summary, the thalamus plays a key role in mediating sensation, motor activities, cortical arousal, learning, and memory. It is truly the gateway to the cerebral cortex. Hypothalamus • Named for its position below (hypo) the thalamus, the hypothalamus caps the brain stem and forms the infero-lateral walls of the third ventricle • Merging into the mid brain inferiorly, the hypothalamus extends from the Optic Chiasma (crossover point of the optic nerves) to the posterior margin of the Mammillary Bodies. • Like the thalamus, the hypothalamus contains many functionally important nuclei. The mammillary bodies, paired pealike nuclei that bulge ventrally from the hypothalamus, are relay stations in the olfactory pathways. • Between the Optic Chiasma And Mammillary bodies is the Infundibulum, a stalk of hypothalamic tissue that connects the pituitary gland to the base of the hypothalamus. Hypothalamus • Despite its small size, the hypothalamus is the main visceral control center of the body and is vitally important to overall body homeostasis. Few tissues in the body escape its influence. Its chief homeostatic roles are to: • Control the autonomic nervous system.The hypothalamus regulates ANS activity by controlling the activity of centers in the brain stem and spinal cord. In this role, the hypothalamus influences blood pressure, rate and force of heartbeat, digestive tract motility, eye pupil size, and many other visceral activities. • Initiate physical responses to emotions. The hypothalamus lies at the "heart" of the limbic system (the emotional part of the brain). It contains nuclei involved in perceiving pleasure, fear, and rage, as well as those involved in biological rhythms and drives (such as the sex drive). The hypothalamus acts through ANS pathways to initiate most physical expressions of emotion. • Regulate body temperature. The body's thermostat is in the hypothalamus. Hypothalamic neurons monitor blood temperature and receive input from other thermoreceptors in the brain and body periphery. Accordingly, the hypothalamus initiates cooling (sweating) or heat-generating actions (shivering) as needed to maintain a relatively constant body temperature • Regulate food intake. In response to changing blood levels of certain nutrients (glucose and amino acids) or hormones (cholecystokinin, ghrelin, and others), the hypothalamus regulates feelings of hunger and satiety. • Regulate water balance and thirst. Osmoreceptors excite hypothalamic nuclei that trigger the release of antidiuretic hormone (ADH) from the posterior pituitary. • Regulate sleep-wake cycles. Its suprachiasmatic nucleus (our biological clock) sets the timing of the sleep cycle primarily in response to daylight-darkness cues received from the visual pathways. • Control endocrine system function. First, its releasing and inhibiting hormones control the secretion of hormones by the anterior pituitary gland. Second, its supraoptic and paraventricular nuclei produce the hormones ADH and oxytocin. Epithalamus • The most dorsal portion of the diencephalon, the epithalamus, forms the Roof Of The Third Ventricle. • Extending from its posterior border and visible externally is the Pineal Gland Or Body, The pineal gland secretes the hormone Melatonin and, along with hypothalamic nuclei, helps regulate the sleep-wake cycle. • The posterior commissure forms the caudal border of the epithalamus. The Brain Stem • From superior to inferior, the brain stem regions are Midbrain, Pons, and Medulla Oblongata. • Each roughly an inch long, collectively they account for only 2.5% of total brain mass. • The tissues of the brain stem are organized in a similar manner to that of the spinal cord, deep gray matter surrounded by white matter fiber tracts. • However, the brain stem has nuclei of gray matter embedded in the white matter, a feature not found in the spinal cord. • Brain stem centers produce the rigidly programmed, automatic behaviors necessary for survival. • Positioned between the cerebrum and the spinal cord, the brain stem also provides a pathway for fiber tracts running between higher and lower neural centers. • Additionally, brain stem nuclei are associated with 10 of the 12 pairs of cranial nerves, so the brain stem is heavily involved with innervating the head. Midbrain • It is located between the diencephalon and pons. On its ventral aspect two bulging cerebral peduncles form vertical pillars that seem to hold up the cerebrum • The crus cerebri "leg of the cerebrum“ of each peduncle contains a large pyramidal (corticospinal) motor tract descending toward the spinal cord. • The superior cerebellar peduncles, also fiber tracts, connect the midbrain to the cerebellum dorsally • Running through the midbrain is the hollow cerebral aqueduct, which connects the third and fourth ventricles. • It delineates the cerebral peduncles ventrally from the tectum to the midbrain's roof. • Surrounding the aqueduct is the periaqueductal gray matter, which is involved in pain suppression and links the fear-perceiving amygdaloid body and ANS pathways that control the "fight-or-flight" response. • The periaqueductal gray matter also includes nuclei that control two cranial nerves, the oculomotor and the trochlear nuclei. Midbrain The corpora quadrigemina, the largest midbrain nuclei, raise Four Domelike Protrusions on the dorsal midbrain surface: • The superior colliculi: are visual reflex centers that coordinate head and eye movements when we visually follow a moving object, even if we are not consciously looking at it. • The inferior colliculi are part of the auditory relay from the hearing receptors of the ear to the sensory cortex. They also act in reflexive responses to sound, such as the startle reflex that causes you to turn your head toward an unexpected noise. • Also embedded in each side of the midbrain white matter are Two Pigmented Nuclei, the substantia nigra and red nucleus. • The bandlike Substantia Nigra is located deep to the cerebral peduncle. Its dark color reflects a high content of melanin pigment, a precursor of the neurotransmitter (dopamine) released by these neurons. The substantia nigra is functionally linked to the basal nuclei and many scientists consider it part of the basal nuclear complex. Degeneration of the dopaminereleasing neurons of the substantia nigra is the ultimate cause of Parkinson's disease. • The oval Red Nucleus lies deep to the substantia nigra. Its reddish hue is due to its rich blood supply and to the presence of iron pigment in its neurons. The red nuclei are relay nuclei in some descending motor pathways that cause limb flexion, and they are embedded in the reticular formation, a system of small nuclei scattered through the core of the brain stem. Midbrain The Pons (pons = bridge) • It is the bulging brain stem region wedged between the midbrain and the medulla oblongata. • Dorsally, the fourth ventricle separates it from the cerebellum. • The pons is chiefly composed of conduction tracts. They are oriented in two different directions: 1. The deep projection fibers run longitudinally as part of the pathway between higher brain centers and the spinal cord. 2. The more superficial ventral fibers are oriented transversely and dorsally. They form the middle cerebellar peduncles and connect the pons bilaterally with the two sides of the cerebellum dorsally. • These fibers issue from numerous pontine nuclei, which relay "conversations" between the motor cortex and cerebellum. • Several cranial nerve pairs issue from pontine nuclei. They include the Trigeminal, Abducens and Facial Nerves • Other important pontine nuclei are part of the reticular formation and some help the medulla oblongata maintain the normal rhythm of breathing. The Pons Medulla Oblongata • Is the most inferior part of the brain stem. It blends gradually into the spinal cord at the level of the foramen magnum of the skull. • The central canal of the spinal cord continues upward into the medulla, where it broadens out to form the cavity of the fourth ventricle. • Together, the medulla and the pons form the ventral wall of the fourth ventricle. Structures of the Medulla Oblongata • Flanking the midline on the medulla's ventral aspect are two longitudinal ridges called pyramids, formed by the large pyramidal (corticospinal) tracts descending from the motor cortex. • Just above the medulla-spinal cord junction, these fibers crossover to the opposite side before continuing into the spinal cord. This crossover point is called the decussation of the pyramids. As a result of this crossover, each cerebral hemisphere chiefly controls the voluntary movements of muscles on the opposite side of the body. • The inferior cerebellar peduncles are fiber tracts that connect the medulla to the cerebellum dorsally. • Situated lateral to the pyramids, the olives are oval swellings. These swellings are caused mainly by the wavy folds of gray matter of the underlying inferior olivary nuclei. • These nuclei relay sensory information on the degree of stretch in muscles and joints to the cerebellum. • The rootlets of the Hypoglossal Nerves emerge from the groove between the pyramid and olive on each side of the brain stem. • Other cranial nerves are the Glossopharyngeal Nerves and Vagus Nerves. The fibers of the Vestibulocochlear Nerves synapse with the cochlear nuclei (auditory relays), and with numerous vestibular nuclei in both the pons and medulla. The vestibular nuclei mediate responses that maintain equilibrium. • Also housed in the medulla are several nuclei associated with ascending sensory tracts. The most prominent are the dorsally located Nucleus Gracilis and Nucleus Cuneatus, associated with a tract called the medial lemniscus . These serve as relay nuclei in a pathway which general somatic and proprioceptive sensory information ascends from the spinal cord to the somatosensory cortex. Functions of the Medulla Oblongata • The small size of the medulla belies its crucial role as An Autonomic Reflex Center involved in maintaining homeostasis. The medulla contains these important functional groups of visceral motor nuclei: • Cardiovascular center. This includes the cardiac center, which adjusts the force and rate of heart contraction to meet the body's needs, and the vasomotor center, which changes blood vessel diameter to regulate blood pressure. • Respiratory centers. These generate the respiratory rhythm and (together with pontine centers) control the rate and depth of breathing. • Various other centers. Additional centers regulate such activities as vomiting, hiccuping, swallowing, coughing, and sneezing. ***Notice that many functions listed above are also attributed to the hypothalamus. The hypothalamus controls many visceral functions by relaying its instructions through medullary reticular centers. The cerebellum • The cauliflower-like cerebellum, exceeded in size only by the cerebrum, accounts for about 11% of total brain mass. • The cerebellum is located dorsal to the pons and medulla (and to the intervening fourth ventricle). • It protrudes under the occipital lobes of the cerebral hemispheres, from which it is separated by the transverse cerebral fissure. • By processing inputs received from the cerebral motor cortex, various brain stem nuclei, and sensory receptors, the cerebellum provides the precise timing and appropriate patterns of skeletal muscle contraction for smooth, coordinated movements and agility needed for our daily living-driving, typing, and playing the tuba. • Cerebellar activity occurs subconsciously -we have no awareness of it. Cerebellar Anatomy • The cerebellum is bilaterally symmetrical. The wormlike vermis connects its two apple-sized cerebellar hemispheres medially. • Its surface is heavily convoluted, with fine, transversely oriented pleatlike gyri known as folia "leaves". • Deep fissures subdivide each hemisphere into Anterior, Posterior, and Flocculonodular Lobes. • The small propeller-shaped flocculonodular lobes, situated deep to the vermis and posterior lobe, cannot be seen in a surface view. • Like the cerebrum, the cerebellum has a thin outer cortex of gray matter, internal white matter, and small, deeply situated, paired masses of gray matter, the most familiar of which are the Dentate Nuclei. • Several types of neurons populate the cerebellar cortex, including Purkinje cells • These large cells, with their extensively branched dendrites, are the only cortical neurons that send axons through the white matter to synapse with the central nuclei of the cerebellum. • The distinctive pattern of white matter in the cerebellum resembles a branching tree, a pattern fancifully called the Arbor Vitae The anterior and posterior lobes of the cerebellum Coordinate body movements, have three sensory maps of the entire body as indicated by the homunculi. The part of the cerebellar cortex that receives sensory input from a body region influences motor output to that region. • The medial portions influence the motor activities of the trunk and girdle muscles. • The intermediate parts of each hemisphere influence the distal parts of the limbs and skilled movements. • The lateral most parts of each hemisphere integrate information from the association areas of the cerebral cortex and appear to play a role in planning movements rather than executing them. • The flocculonodular lobes receive inputs from the equilibrium apparatus of the inner ears, and adjust posture to maintain balance. The cerebellum Cerebellar Processing-fine-tunes motor activity as follows: 1. The motor areas of the cerebral cortex, via relay nuclei in the brain stem, notify the cerebellum of their intent to initiate voluntary muscle contractions. 2. At the same time, the cerebellum receives information from proprioceptors throughout the body (regarding tension in the muscles and tendons, and joint position) and from visual and equilibrium pathways. This information enables the cerebellum to evaluate body position and momentum-where the body is and where it is going. 3. The cerebellar cortex calculates the best way to coordinate the force, direction, and extent of muscle contraction to prevent overshoot, maintain posture, and ensure smooth, coordinated movements. 4. Then, via the superior peduncles, the cerebellum dispatches to the cerebral motor cortex its "blueprint" for coordinating movement. Cerebellar fibers also send information to brain stem nuclei, which in turn influence motor neurons of the spinal cord. *** Cerebellar injury results in loss of muscle tone and clumsy, uncertain movements. The spinal cord • It enclosed in the vertebral column, extends from the Foramen Magnum of the skull to The First or Second Lumbar Vertebra, just inferior to the ribs. About 42 cm long and 1.8 cm thick, the glistening white spinal cord provides a two-way conduction pathway to and from the brain. • It is a major reflex center: Spinal reflexes are initiated and completed at the spinal cord level. • Like the brain, the spinal cord is protected by Bone, Meninges, And Cerebrospinal Fluid. The single-layered Spinal Dura Mater is not attached to the bony walls of the vertebral column. Between the bony vertebrae and the spinal dura mater is an Epidural Space filled with a soft padding of fat and a network of veins. • Cerebrospinal fluid fills the Subarachnoid Space between the Arachnoid and Pia Mater Meninges. • Inferiorly, the dural and arachnoid membranes extend to the level of S2, well beyond the end of the spinal cord. The spinal cord typically ends between L1 and L2. For this reason, the subarachnoid space within the meningeal sac inferior to that point provides an ideal spot for removing Cerebrospinal Fluid for Testing, a procedure called a Lumbar Puncture • Because the spinal cord is absent there and the delicate nerve roots drift away from the point of needle insertion, there is little or no danger of damaging the cord (or spinal roots) beyond L3. Foramen Magnum It enclosed in the vertebral column, extends from the Foramen Magnum of the skull to The First or Second Lumbar Spinal cord segments. Diagram of the spinal cord in sagittal view showing the position of spinal cord segments and the spinal nerves. The vertebral bodies, shown on the right, are numbered. Those in the lumbar and sacral regions are displaced anteriorly to illustrate the emergence of the spinal nerves. Gross structure of the spinal cord, dorsal view The spinal cord • Inferiorly, the spinal cord terminates in a tapering cone-shaped structure called the Conus Medullaris. The Filum Terminate, a fibrous extension of the conus covered by pia mater, extends inferiorly from the Conus Medullaris to the coccyx, where it anchors the spinal cord so it is not pushed by body movements. • Furthermore, saw-toothed nerves emerge from the spinal cord. While each nerve pair defines a segment of the cord, the spinal cord is, in fact, continuous throughout its length and its internal structure changes gradually. Each nerve exits from the vertebral column by passing adjacent to its corresponding vertebra via the intervertebral foramen, and travels to the body region it serves. • Because the cord does not reach the end of the vertebral column, the spinal cord segments are located superior to where their corresponding spinal nerves emerge through the intervertebral foramina. • The lumbar and sacral spinal nerve roots angle sharply downward and travel inferiorly through the vertebral canal for some distance before reaching their intervertebral foramina. • The collection of nerve roots at the inferior end of the vertebral canal is named the Cauda Equina. This strange arrangement reflects the fact that during fetal development, the vertebral column grows faster than the spinal cord, forcing the lower spinal nerve roots to "chase" their exit points inferiorly through the vertebral canal. The spinal cord-Cross-Sectional Anatomy The spinal cord is somewhat flattened from front to back and two grooves mark its surface: the wide Ventral (Anterior) Median Fissure and the narrower Dorsal (Posterior) Median Sulcus. These grooves run the length of the cord and partially divide it into right and left halves. The gray matter of the cord is located in its core, the white matter outside. The cerebrospinal fluid-filled central canal runs the length of the spinal cord. Gray Matter and Spinal Roots • In cross section the gray matter of the cord looks like the letter H or like a butterfly. It consists of mirror-image lateral gray masses connected by a crossbar of gray matter, the gray commissure, that encloses the central canal. • The two dorsal projections of the gray matter are the dorsal (posterior) horns, and the ventral pair are the ventral (anterior) horns. In 3-D, these horns form columns of gray matter that run the entire length of the spinal cord. • The thoracic and superior lumbar segments of the cord have an additional pair of gray matter columns, the small lateral horns. • All neurons whose cell bodies are in the spinal cord gray matter are Multipolar. The dorsal horns consist entirely of Interneurons. The ventral horns have some interneurons but mainly house cell bodies of somatic motor neurons. • These motor neurons send their axons out to the skeletal muscles (their effector organs) via the ventral rootlets that fuse together to become the ventral roots of the spinal cord. The amount of ventral gray matter present at a given level of the spinal cord reflects the amount of skeletal muscle innervated at that level. • As a result, the ventral horns are largest in the limb-innervating cervical and lumbar regions of the cord and are responsible for the cord enlargements seen in those regions. • The lateral horns consist mostly of the cell bodies of autonomic (sympathetic division) motor neurons that serve visceral organs. Their axons leave the cord via the ventral root along with those of the somatic motor neurons. • Because the ventral roots contain both somatic and autonomic efferent fibers, they serve both motor divisions of the peripheral nervous system. The spinal cord • Afferent fibers carrying impulses from peripheral sensory receptors form the dorsal roots of the spinal cord that fan out as the dorsal rootlets before they enter the spinal cord. • The cell bodies of the associated sensory neurons are found in an enlarged region of the dorsal root called the dorsal root ganglion or spinal ganglion. • After entering the cord, the axons of these neurons may take a number of routes. Some enter the dorsal white matter of the cord directly and travel to synapse at higher cord or brain levels. • Others synapse with interneurons in the dorsal horns of the spinal cord gray matter at their entry level. The dorsal and ventral roots are very short and fuse laterally to form the spinal nerves. • The spinal gray matter can be divided further according to its neurons' relative involvement in innervating the somatic and visceral regions of the body. Spinal gray matter has the following four zones : Somatic Sensory (SS), Visceral Sensory (VS), Visceral (Autonomic) Motor (VM), Somatic Motor (SM). Organization of the gray matter of the spinal cord. The gray matter of the spinal cord is divided into a sensory half dorsally and a motor half ventrally. Note that the dorsal and ventral roots are part of the PNS, not of the spinal cord. The spinal cord White Matter • The white matter of the spinal cord is composed of myelinated and nonmyelinated nerve fibers that allow communication between different parts of the spinal cord and between the cord and brain. These fibers run in three directions: 1. Ascending-up to higher centers (sensory inputs) 2. Descending-down to the cord from the brain or within the cord to lower levels (motor outputs) 3. Transverse-across from one side of the cord to the other (commissural fibers) **** Ascending and descending tracts make up most of the white matter. • The white matter on each side of the cord is divided into three white columns, or funiculi named according to their position as Dorsal (Posterior), Lateral, And Ventral (Anterior) Funiculi. • Each funiculus contains several fiber tracts, and each tract is made up of axons with similar destinations and functions. With a few exceptions, the names of the spinal tracts reveal both their origin and destination. Meninges • The brain is protected by bone, meninges, cerebrospinal fluid, and the blood brain barrier. • The Meninges are Three Connective Tissue Membranes that lie just external to the CNS organs. • The meninges: • Cover and protect the CNS • Protect blood vessels and enclose venous sinuses • Contain cerebrospinal fluid • Form partitions in the skull • From external to internal, the meninges are: the Dura Mater, Arachnoid Mater, and Pia Mater Dura Mater • The leathery dura mater is the strongest meninx. Where it surrounds the brain, it is a two-layered sheet of fibrous connective tissue. • The more superficial periosteal layer attaches to the inner surface of the skull (the periosteum). • There is No Dural Periosteal Layer surrounding the spinal cord. • The deeper meningeal layer forms the true external covering of the brain and continues caudally in the vertebral canal as the spinal dura mater. • The brain's two dural layers are fused together except in certain areas, where they separate to Enclose Dural Venous Sinuses that collect venous blood from the brain and direct it into the internal jugular veins of the neck. • ln several places, the meningeal dura mater extends inward to form Flat Partitions that subdivide the cranial cavity. These Dural septa, which limit excessive movement of the brain within the cranium, include the following: • Falx cerebri: A large sickle-shaped fold that dips into the longitudinal fissure between the cerebral hemispheres. Anteriorly, it attaches to the crista galli of the ethmoid bone. • Falx cerebelli: Continuing inferiorly from the posterior falx cerebri, this small midline partition runs along the vermis of the cerebellum. • Tentorium cerebelli: Resembling a tent over the cerebellum, this nearly horizontal Dural fold extends into the transverse fissure between the cerebral hemispheres and the cerebellum Arachnoid Mater • The middle meninx, the arachnoid mater forms a loose brain covering, never dipping into the sulci at the cerebral surface. • It is separated from the dura mater by a narrow serous cavity, the subdural space, which contains a film of fluid. • Beneath the arachnoid membrane is the wide subarachnoid space. Spider web-like extensions span this space and secure the arachnoid mater to the underlying pia mater. • The subarachnoid space is filled with cerebrospinal fluid and also contains the largest blood vessels serving the brain. Because the arachnoid mater is fine and elastic, these blood vessels are poorly protected. • Knob like projections of the arachnoid mater called Arachnoid Granulations protrude superiorly through the dura mater and into the superior sagittal. • These granulations absorb cerebrospinal fluid into the venous blood of the sinus. The pia mater • "gentle mother," is composed of delicate connective tissue and contains many tiny blood vessels. • It is the only meninx that clings tightly to the brain like plastic wrap, following its every convolution. • Small arteries entering the brain tissue carry ragged sheaths of pia mater inward with them for short distances. Cerebrospinal fluid (CSF) • Cerebrospinal fluid, found in and around the brain and spinal cord, forms a liquid cushion that gives buoyancy to CNS structures. By floating the jellylike brain, the CSF effectively reduces brain weight by 97% and prevents the delicate brain from crushing under its own weight. • CSF also protects the brain and spinal cord from blows and other trauma. Additionally, although the brain has a rich blood supply, CSF helps nourish the brain, and there is some evidence that it carries chemical signals (such as hormones and sleep- and appetite-inducing molecules) from one part of the brain to another. • CSF is a watery "broth" Similar In Composition To Blood Plasma, from which it is formed. However, it contains less protein than plasma and its ion concentrations are different. • For example, CSF contains more Na+, Cl-, and H+ than does blood plasma, and less Ca2 + and K+. • The choroid plexuses that hang from the roof of each ventricle form CSF. These plexuses are frondshaped clusters of broad, thin-walled capillaries enclosed first by Pia Mater and then by a layer of Ependymal Cells lining the ventricles. Cerebrospinal fluid (CSF) • These capillaries are fairly permeable, and tissue fluid filters continuously from the bloodstream. • However, the choroid plexus ependymal cells are joined by tight junctions, and they have ion pumps that allow them to modify this filtrate by actively transporting only certain ions across their membranes into the CSF pool. • This careful regulation of CSF composition is important because CSF mixes with the extracellular fluid bathing neurons and influences the composition of this fluid. • Ion pumping also sets up ionic gradients that cause water to diffuse into the ventricles. • ln adults, the total CSF volume of about 150 ml (about half a cup) is replaced every 8 hours or so. About 500 ml of CSF is formed daily. • The choroid plexuses also help cleanse the CSF by removing waste products and unnecessary solutes. Cerebrospinal fluid (CSF) • Once produced, CSF moves freely through the ventricles. • CSF enters the subarachnoid space via the lateral and median apertures in the walls of the fourth ventricle. • The long cilia of the ependymal cells lining the ventricles help keep the CSF in constant motion. • In the subarachnoid space, CSF bathes the outer surfaces of the brain and spinal cord and then returns to the blood in the dural sinuses via the arachnoid granulations. Blood Brain Barrier • The blood brain barrier is the protective mechanism that helps maintain the brain's stable environment. • Exceptionally Impermeable Tight Junctions between capillary endothelial cells are its major component. Surrounding the endothelial cells are the bulbous feet of astrocytes and smooth muscle-like cells called pericytes. • Together these supporting cells regulate and maintain the endothelial cells. In particular, they signal the endothelial cells to form tight junctions that are very tight ** If the brain were exposed to chemical variations, its neurons would fire uncontrollably, because some hormones and amino acids serve as neurotransmitters and certain ions (particularly K+) modify the threshold for neuronal firing. Blood Brain Barrier The presence of tight junctions prevents molecules from moving between the endothelial cells from the blood to the brain. Instead, everything must pass through the plasma membrane into an endothelial cell and out the other side. Simple diffusion allows lipid-soluble substances to pass freely. Fats, fatty acids, oxygen, carbon dioxide, and other lipid-soluble molecules diffuse easily through the plasma membranes. This explains why drugs that affect the brain (including alcohol, nicotine, and anesthetics) are lipid soluble. Specific transport mechanisms move substances important to the brain. Nutrients such as glucose, amino acids, and specific ions move passively by Facilitated Diffusion. Transcytosis moves larger substances into and out of the brain. Blood-borne metabolic wastes, proteins, certain toxins, and most drugs are denied entry. Small nonessential amino acids and potassium ions not only are prevented from entering the brain, but are also Actively Pumped from the brain across the capillary endothelium. Blood Brain Barrier • The basement membrane of the endothelial cells forms the last part of the blood brain barrier. It contains enzymes that destroy certain chemicals (such as Epinephrine And Norepinephrine) that would otherwise activate brain neurons. • The structure of the blood brain barrier is not completely uniform. In some brain areas surrounding the third and fourth ventricles, the barrier is entirely absent and the capillary endothelium is quite permeable, allowing blood-born molecules easy access to the neural tissue. One such region is the vomiting center of the brain stem, which monitors the blood for poisonous substances. • Another is in the hypothalamus, which regulates water balance, body temperature, and many other metabolic activities. Lack of blood brain barrier here is essential to allow the hypothalamus to sample the chemical composition of the blood. • The barrier is incomplete in newborn and premature infants, and potentially toxic substances can enter the CNS and cause problems not seen in adults. Injury to the brain, whatever the cause, may result in a localized breakdown of the blood brain barrier. Most likely, this breakdown reflects some change in the capillary endothelial cells or their tight junctions. The interconnected structures of the brain allow higher mental functions • Our ability to discuss "souls and synapses" is one of the things that makes us human. Language is such an important function of the brain that it involves practically all of the association cortex on the left side in one way or another. Pioneering studies of patients with aphasias (the loss of language abilities due to damage to specific areas of the brain) pointed to two critically important regions, Broca's area and Wernicke's area. Patients with lesions involving Broca's area can understand language but have difficulty speaking (and sometimes cannot write or type or use sign language). On the other hand, patients with lesions involving Wernicke's area are able to speak but produce a type of nonsense often referred to as "word salad.“ Broca's and Wernicke's areas together with the basal nuclei form a single language implementation system that analyzes incoming and produces outgoing word sounds and grammatical structures. The corresponding areas in the right or non-language- dominant hemisphere are involved in "Body Language"-the nonverbal emotional components of language. These areas allow the lilt or tone of our voice and our gestures to express our emotions when we speak, and permit us to comprehend the emotional content of what we hear Memory • Is the storage and retrieval of information. Memories are essential for learning and incorporating our experiences into behavior and are an integral part of our consciousness. • There are different kinds of memory: Declarative (Fact) Memory (names, faces, words, and dates), Procedural (Skills) Memory (piano playing), Motor Memory (riding a bike), and Emotional Memory (your pounding heart when you hear a rattlesnake nearby). • Declarative memory storage involves two distinct stages: Short-term Memory and Long-term Memory. • Short-term memory (STM), also called working memory, is the preliminary step, as well as the power that lets you look up a telephone number, dial it, and then never think of it again. STM is limited to seven or eight chunks of information, such as the digits of a telephone number or the sequence of words in an elaborate sentence. • Long-term memory (LTM) seems to have a limitless capacity. Although our STM cannot recall numbers much longer than a telephone number, we can remember the lyrics to many songs by committing them to LTM. However, long-term memories can be forgotten, and so our memory bank continually changes with time. Furthermore, our ability to store and retrieve information declines with aging. We do not remember or even consciously notice much of what is going on around us. As sensory inputs flood into our cerebral cortex, they are processed. • Some 5% of this information is selected for transfer to STM. STM serves as a temporary holding bin for data that we may or may not want to retain. Memory Information is then transferred from STM to LTM. Many factors can influence this transfer, including: • Emotional state. We learn best when we are alert, motivated, surprised, or aroused. For example, when we witness shocking events, transferal is almost immediate. Norepinephrine, a neurotransmitter involved in memory processing of emotionally charged events, is released when we are excited or "stressed out," which helps to explain this phenomenon. • Rehearsal. Rehearsing or repeating the material enhances memory. • Association. Tying "new" information to "old" information already stored in LTM appears to be important in remembering facts. • Automatic memory. Not all impressions that become part of LTM are consciously formed. A student concentrating on a lecturer's speech may record an automatic memory of the pattern of the lecturer's tie. Memory • Memories transferred to LTM take time to become permanent. • The process of Memory Consolidation apparently involves fitting new facts into the categories of knowledge already stored in the cerebral cortex. • The Hippocampus and Surrounding Temporal Cortical areas play a major role in memory consolidation by communicating with the thalamus and the prefrontal cortex. • Specific pieces of each memory are thought to be stored near regions of the brain that need them so new inputs can be quickly associated with the old. Accordingly, visual memories are stored in the occipital cortex, memories of music in the temporal cortex, and so on… Consciousness • Consciousness includes perception of sensations, voluntary initiation and control of movement, and capabilities associated with higher mental processing (memory, logic, judgment, perseverance, and so on). • Clinically, consciousness is defined on a Continuum that grades behavior in response to stimuli as (1) alertness, (2) drowsiness or lethargy (which proceeds to sleep), (3) stupor, and (4) coma. • Alertness is the highest state of consciousness and cortical activity, and coma the most depressed. • Consciousness is difficult to define. A sleeping person obviously lacks something that he or she has when awake, and we call this "something" consciousness. Current suppositions about consciousness are as follows: • Consciousness involves simultaneous activity of large areas of the cerebral cortex. • It is superimposed on other types of neural activity. At any time, specific neurons and neuronal pools are involved both in localized activities (such as motor control) and in cognition. • It is holistic and totally interconnected. Information for "thought" can be claimed from many locations in the cerebrum simultaneously. For example, retrieval of a specific memory can be triggered by several routes-a smell, a place, a particular person, and so on. Brain injuries and disorders have devastating consequences Traumatic Brain Injuries: • Brain damage is caused not only by localized injury at the site of the blow, but also by the ricocheting brain hitting the opposite end of the skull. • A concussion is an alteration in brain function, usually temporary, following a blow to the head. • The victim may be dizzy or lose consciousness. multiple concussions over time produce cumulative damage. • Permanents neurological damage, a condition called a contusion. Following a head injury, death may result from subdural or subarachnoid hemorrhage (bleeding from ruptured vessels into those spaces). • Blood accumulating in the skull increases intracranial pressure and compresses brain tissue. If the pressure forces the brain stem inferiorly through the foramen magnum, control of blood pressure, heart rate, and respiration is lost. • Another consequence of traumatic head injury is cerebral edema, swelling of the brain Cerebrovascular Accidents (CVAs) also called strokes. • Occur when blood circulation to a brain area is blocked and brain tissue dies of ischemia. The most common cause of CVA is a blood clot that blocks a cerebral artery or form on the roughened interior wall of a brain artery narrowed by atherosclerosis • Many who survive a CVA are paralyzed on one side of the body (hemiplegia). • exhibit sensory deficits or have difficulty understanding or vocalizing speech. • If motor function is impaired, physical therapy should start as soon as possible to prevent muscle contractures • Temporary episodes of reversible cerebral ischemia, called Transient Ischemic Attacks (TIAs) characterized by temporary numbness, paralysis, or impaired speech. • Glutamate plays a key role in learning and memory, as well as other critical brain functions. After brain injury, neurons totally deprived of oxygen begin to disintegrate, unleashing the cellular equivalent of a tidal wave of glutamate. Under these conditions, glutamate acts as an excitotoxin, literally exciting surrounding cells to death. • At present, the most successful treatment for stroke is tissue plasminogen activator (tPA), which dissolves blood clots in the brain. Degenerative Brain Disorders Alzheimer's disease (AD): • a progressive degenerative disease of the brain, ultimately results in dementia (mental deterioration). • AD patients exhibit memory loss (particularly for recent events), shortened attention span, disorientation, and eventual language loss. Over a period of several years, formerly good-natured people may become irritable, moody, and confused. • Examinations of brain tissue reveal Senile Plaques littering the brain like shrapnel between the neurons. The plaques consist of extracellular aggregations of Beta-Amyloid Peptide, which is cut from a normal membrane precursor protein (APP) by enzymes. • One form of Alzheimer's disease is caused by an inherited mutation in the gene for APP, which suggests that too much betaamyloid may be toxic. • Current clinical trials focus on using the immune system to clear away beta-amyloid peptide. Another hallmark of Alzheimer's disease is the presence of Neurofibrillary Tangles inside neurons. These tangles involve a protein called Tau, which functions like railroad ties to bind microtubule "tracks" together. • In the brains of AD patients, tau abandons its microtubule-stabilizing role and grabs on to other tau molecules, forming spaghetti-like neurofibrillary tangles, which kill the neurons by disrupting their transport mechanisms. Both plaques and tangles come about because the proteins that comprise them have misfolded. • These misfolded proteins clump together, and also catalyze the misfolding of normally folded copies of the same proteins. Evidence suggests that this protein misfolding spreads in a predictable way from one region of the brain to another. Alzheimer's disease (AD): • This helps explain both the different types of dementia and their progression, as neurons in more and more brain regions die. • As the brain cells die, their functions are lost and the brain shrinks. Particularly vulnerable brain areas include the hippocampus, the basal forebrain and association areas of the cortex, all regions involved in thinking and memory • Neurons that use the neurotransmitter acetylcholine are particularly vulnerable, and their loss is associated with a shortage of this neurotransmitter. • Drugs that inhibit breakdown of acetylcholine slightly enhance cognitive function in AD patients. Parkinson's disease • Results from a degeneration of the dopamine-releasing neurons of the Substantia Nigra. As those neurons deteriorate, the dopamine-deprived basal nuclei they target become overactive. • Afflicted individuals have a persistent tremor at rest, a forward-bent walking posture and shuffling gait, and a stiff facial expression. They are slow initiating and executing movement. • Parkinson's disease becomes more common with age. Its cause is still unknown, but multiple factors may interact to destroy dopamine-releasing neurons. Recent evidence points to abnormalities in certain mitochondrial proteins and protein degradation pathways. The drug L-DOPA helps to alleviate some symptoms. • L-DOPA passes through the blood brain barrier and is then converted into dopamine. However, as more and more neurons die off, L-dopa becomes ineffective. Mixing L-dopa with drugs that inhibit the breakdown of dopamine can prolong its effectiveness. Early in the disease, these drugs alone slow the neurologi