Week 11 Renal Physiology Lecture Slides PDF
Document Details
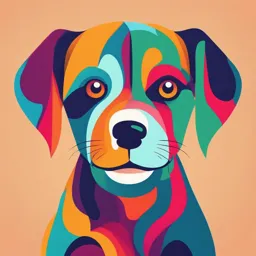
Uploaded by EnoughLion
Charles Sturt University
Tags
Summary
Lecture slides on renal physiology, covering topics such as renal anatomy and its function, renal conditions, and processes of urine formation. The slides detail the structure of the kidneys, blood vessels, nerves, and other anatomical components, making it an ideal resource for undergraduates.
Full Transcript
EHR522 EXERCISE FOR METABOLIC & RENAL CONDITIONS WEEK 11 – RENAL PHYSIOLOGY RENAL PHYSIOLOGY • Every day the kidneys filter nearly 200 litres of fluid from the bloodstream • This process allows toxins, metabolic wastes and excess ions to leave the body in urine while returning needed substances t...
EHR522 EXERCISE FOR METABOLIC & RENAL CONDITIONS WEEK 11 – RENAL PHYSIOLOGY RENAL PHYSIOLOGY • Every day the kidneys filter nearly 200 litres of fluid from the bloodstream • This process allows toxins, metabolic wastes and excess ions to leave the body in urine while returning needed substances to the blood 2 RENAL PHYSIOLOGY • The kidneys maintain the body’s internal environment by − Regulating the total volume of water in the body and the total concentration of solutes in that water (osmolality) − Regulating the concentrations of various ions in the extracellular fluid (even relatively small changes in some ion concentrations such as K+ can be fatal) − Ensuring long-term acid-base balance − Excreting metabolic wastes and foreign substances such as drugs or toxins − Producing erythropoietin and renin, important molecules for regulating red blood cell production and blood pressure, respectively − Converting vitamin D to its active form − Carrying out gluconeogenesis during prolonged fasting 3 KIDNEYS – LOCATION AND EXTERNAL ANATOMY • The bean-shaped kidneys lie in a retroperitoneal position • Extending approximately from T12 to L3, the kidneys receive some protection from the lower part of the rib cage • The right kidney is crowded by the liver and lies slightly lower than the left • The ureter, renal blood vessels, lymphatics and nerves all join each kidney at the hilum and occupy the sinus • Atop each kidney is an adrenal (or suprarenal) gland, an endocrine gland that is functional unrelated to the kidney 4 KIDNEYS – LOCATION AND EXTERNAL ANATOMY • Three layers of supportive tissue surround each kidney. From superficial to deep, these are: − The renal fascia, an outer layer of dense fibrous connective tissue that anchors the kidney and the adrenal gland to surrounding structures − The perirenal fat capsule, a fatty mass that surrounds the kidney and cushions it against blows − The fibrous capsule, a transparent capsule that prevents infections in surrounding regions from spreading to the kidney 5 KIDNEYS – INTERNAL GROSS ANATOMY • A frontal section through a kidney reveals three distinct regions: − Cortex − Medulla − Pelvis • The renal calyces collect urine, which drains continuously from the papillae, and empty it into the renal pelvis • The urine then flows through the renal pelvis and into the ureter, which moves it to the bladder to be stored • The walls of the calyces, pelvis and ureter contain smooth muscle that contracts rhythmically to propel urine by peristalsis 6 KIDNEYS – BLOOD AND NERVE SUPPLY • Under normal resting conditions, the large renal arteries deliver one-fourth of the total cardiac output (about 1200mL) to the kidneys each minute • The renal arteries exit at right angles from the abdominal aorta, and the right renal artery is longer than the left because the aorta lies to the left of the midline • As each renal artery approaches a kidney, it divides into five segmental arteries • Within the renal sinus, each segmental artery branches further to form interlobar arteries 7 KIDNEYS – BLOOD AND NERVE SUPPLY • At the cortex-medulla junction, the interlobar arteries branch into the arcuate arteries that arch over the bases of the medullary pyramids • Small cortical radiate arteries (also called interlobar arteries) radiate outward from the arcuate arteries to supply the cortical tissue • Afferent arterioles branching from the cortical radiates arteries begin a complex arrangement of microscopic blood vessels. These vessels are key elements of kidney function 8 KIDNEYS – BLOOD AND NERVE SUPPLY • Veins pretty much trace the pathway of the arterial supply in reverse • Blood leaving the renal cortex drains sequentially into the cortical radiate, arcuate, interlobar and finally renal veins (there are no segmental veins) • The renal veins exit from the kidneys and empty into the inferior vena cava. Because the inferior vena cava lies to the right of the vertebral column, the left renal vein is about twice as long as the right 9 KIDNEYS – BLOOD AND NERVE SUPPLY • The renal plexus, a variable network of autonomic nerve fibres and ganglia, provides the nerve supply of the kidney and its ureter • Sympathetic vasomotor fibres regulate renal blood flow by adjusting the diameter of renal arterioles and also influence the formation of urine by the nephron 10 NEPHRONS • Nephrons are the structural and functional units of the kidneys • Each kidney contains over one million of these tiny bloodprocessing units, which carry out the processes that form urine • In addition, there are thousands of collecting ducts, each of which collects fluid from several nephrons and conveys it to the renal pelvis • Each nephron consists of a renal corpuscle and a renal tubule • All of the renal corpuscles are located in the renal cortex, while the renal tubules begin in the cortex and then pass into the medulla before returning to the cortex 11 RENAL CORPUSCLE • Each renal corpuscle consists of a tuft of capillaries called a glomerulus and a cup-shaped hollow structure called the glomerular capsule (or Bowman’s capsule) • The glomerular capsule completely surrounds the glomerulus and is continuous with its renal tubule • Glomerulus – The endothelium of the glomerular capillaries is fenestrated (penetrated by many pores), which makes these capillaries exceptionally porous. This property allows large amounts of solute-rich, but virtually protein-free, fluid to pass from the blood into the glomerular capsule. This plasmaderived fluid or filtrate is the raw material that the renal tubules process to form urine 12 RENAL CORPUSCLE • Glomerular Capsule − Parietal layer – This layer contributes to the capsule structure, but plays no part in forming filtrate − Visceral layer – Clings to the glomerular capillaries and contains filtration slits. Through these slits, filtrate enters the capsule space inside the glomerular capsule 13 RENAL TUBULE AND COLLECTING DUCT • The renal tubule is about 3cm long and has three major parts • It leaves the glomerular capsule as the elaborately coiled proximal convoluted tubule, drops into a hairpin loop called the nephron loop, and then winds and twists again as the distal convoluted tubule before emptying into the collecting duct • The terms proximal and distal indicate the relationship of the convoluted tubules to the renal corpuscle • The meandering nature of the renal tubule increases its length and enhances its filtrate processing capabilities 14 CLASSES OF NEPHRONS • Nephrons are generally divided into two major groups, cortical and juxtamedullary − Cortical Nephrons – Account for 85% of the nephrons in the kidneys. Except for small parts of their nephron loops that dip into the outer medulla, they are located entirely in the cortex − Juxtamedullary Nephrons – Originate close to the cortexmedulla junction, and they play an important role in the kidneys’ ability to produce concentrated urine. They have long nephron loops that deeply invade the medulla 15 NEPHRON CAPILLARY BEDS • The renal tubule of every nephron is closely associated with two capillary beds - The glomerulus - The peritubular capillaries • In addition, juxtamedullary nephrons are associated with special capillaries called the vasa recta 16 GLOMERULUS • The glomerulus, in which the capillaries run in parallel is specialised for filtration. It differs from all other capillary beds in the body in that it is both fed and drained by arterioles – the afferent arteriole and efferent arteriole, respectively • Filtration produces a large amount of fluid, most (99%) of which is reabsorbed by the renal tubule cells and returned to the blood in the peritubular capillary beds • The afferent arterioles arise from the cortical radiate arteries that run through the renal cortex. The efferent arterioles feed into either the peritubular capillaries or the vasa recta 17 PERITUBULAR CAPILLARIES • The peritubular capillaries cling closely to adjacent renal tubules and empty into nearby venules. • Because they arise from the high-resistance efferent arterioles, they only experience low pressure. As a result, these low pressure, porous capillaries readily absorb solutes and water from the tubule cells as these substances are reclaimed from the filtrate • Renal tubules are closely packed together, so the peritubular capillaries of each nephron absorb substances from several adjacent nephrons 18 VASA RECTA • Efferent arterioles serving the juxtamedullary nephrons tend not to break up into meandering peritubular capillaries. Instead they form bundles of long straight vessels called vasa recta that extend deep into the medulla paralleling the longest nephron loops • The thin-walled vasa recta play an important role in forming concentrated urine • In summary, nephrons have two functionally different capillary beds - The first capillary bed (the glomerulus) produces the filtrate - The second (a combination of peritubular capillaries and vasa recta) reclaims most of that filtrate 19 JUXTAGLOMERULAR COMPLEX (JGC) • Each nephron has a juxtaglomerular complex (JGC), a region where the most distal portion of the nephron loop lies against the afferent arteriole feeding the glomerulus • The JGC includes cells that help regulate the rate of filtrate formation and systemic blood pressure 20 MECHANISMS OF URINE FORMATION • Urine formation and the adjustment of blood composition involve three processes 1. Glomerular filtration – Takes place in the renal corpuscle and produces a cell- and protein-free filtrate 2. Tubular reabsorption – Is the process of selectively moving substances from the filtrate back into the blood. It takes place in the renal tubules and collecting ducts. Tubular reabsorption reclaims almost everything filtered – all of the glucose and amino acids, and some 99% of the water, salt and other components. Anything that is not reabsorbed becomes urine 3. Tubular secretion – Is the process of selectively moving substances from the blood into filtrate. Like tubular reabsorption, it occurs along the length of the tubule and collecting duct 21 MECHANISMS OF URINE FORMATION • Of the approximately 1200mL of blood that passes through the glomeruli each minute, some 650mL is plasma, and about one-fifth of this (120 – 125mL) is forced into the glomerular capsules as filtrate • Filtrate and urine are quite different. Filtrate contains everything found in blood plasma except proteins. Urine contains unneeded substances such as excess salts and metabolic wastes • The kidneys process about 180L of blood-derived fluid daily. Of this amount, less than 1% (1.5L) typically leaves the body as urine; the rest returns to the circulation 22 URINE FORMATION – STEP 1: GLOMERULAR FILTRATION • Glomerular filtration is a passive process in which hydrostatic pressure forces fluids and solutes through a membrane • The filtration membrane lies between the blood and the interior of the glomerular capsule. It is a porous membrane that allows free passage of water and solutes smaller than plasma protein • Molecules smaller than 3nm in diameter – such as water, glucose, amino acids and nitrogenous wastes – pass freely from the blood into the glomerular capsule. As a result, these substances usually have similar concentrations in the blood and the glomerular filtrate 23 URINE FORMATION – STEP 1: GLOMERULAR FILTRATION • Larger molecules pass with greater difficulty, and those larger than 5nm are generally barred from entering the tubule • Keeping the plasma proteins in the capillaries maintains the colloid osmotic (oncotic) pressure of the glomerular blood, preventing the loss of all its water to the capsular space • The presence of proteins or blood cells in the urine usually indicates a problem with the filtration membrane 24 PRESSURES THAT AFFECT FILTRATION • Outward pressures promote formation - The hydrostatic pressure is glomerular capillaries (HPgc) is essentially glomerular blood pressure. It is the chief force pushing water and solutes out of the blood and across the filtration membrane • Two inward pressure inhibit filtrate formation by opposing HPgc - The hydrostatic pressure in the capsular space (HPcs) is the pressure exerted by filtrate in the glomerular capsule - The colloid osmotic pressure in glomerular capillaries (OPgc) is the pressure exerted by the proteins in the blood • The above pressures determine the net filtration pressure (NFP). NFP largely determines the glomerular filtration rate 25 GLOMERULAR FILTRATION RATE (GFR) • The glomerular filtration rate (GFR) is the volume of filtrate formed each minute by the combined activity of all two million glomeruli of the kidneys. GFR is directly proportional to each of the following factors 1. Net filtration pressure (NFP) – Is the main controllable factor. Of the pressures determining NFP, the most important is hydrostatic pressure in the glomerulus. This pressure can be controlled by changing the diameter of the afferent (and sometimes the efferent) arterioles 2. Total surface are available for filtration – Glomerular capillaries have a huge surface area (collectively equal to the surface area of the skin) 3. Filtration membrane permeability – Glomerular capillaries are thousands of times more permeable than other capillaries because of their fenestrations 26 GLOMERULAR FILTRATION RATE (GFR) • The huge surface area and high permeability of the filtration membrane explain how the relatively modest 10 mmHg NFP can produce large amounts of filtrate • The adult kidneys produce about 180L of filtrate daily. This 180L of filtrate per day translates to the normal GFR of 120 – 125 mL/min 27 REGULATION OF GLOMERULAR FILTRATION • GFR is tightly regulated to serve two crucial and sometimes opposing needs • The kidneys need a relatively constant GFR to make filtrate and do their job maintaining extracellular homeostasis • On the other hand, the body as a whole needs a constant blood pressure, and this is closely tied to GFR in the following way: assuming nothing else changes, an increase in GFR increases urine output, which reduces blood volume and blood pressure. The opposite holds true for a decrease in GFR 28 REGULATION OF GLOMERULAR FILTRATION • Two types of controls serve these two different needs - Intrinsic controls (renal autoregulation) – Act locally within the kidney to maintain GFR - Extrinsic controls – By the nervous and endocrine systems maintain blood pressure • In extreme changes of blood pressure (mean arterial pressure less than 80 or greater 180 mmHg), the extrinsic controls take precedence over intrinsic controls in an effort to prevent damage to the brain and other crucial organs • GFR can be controlled by changing a single variable – glomerular hydrostatic pressure. All major control mechanisms act primarily to change this one variable. If the glomerular hydrostatic pressure rises, NFP rises and so does GFR. If the glomerular hydrostatic pressure falls by as little as 18%, GFR drops to zero 29 INTRINSIC CONTROLS: RENAL AUTOREGULATION • By adjusting its own resistance to blood flow, a process called renal autoregulation, the kidney can maintain a nearly constant GFR despite fluctuations in systemic arterial blood pressure • Renal autoregulation uses two different mechanisms - Myogenic mechanism - Tubuloglomerular feedback mechanism 30 MYOGENIC MECHANISM • The myogenic mechanism reflects a property of vascular smooth muscle – it contracts when stretched and relaxes when not stretched • Rising systemic blood pressure stretches vascular smooth muscle in arteriolar walls, causing the afferent arterioles to constrict • This constriction restricts blood flow into the glomerulus and prevents glomerular blood pressure from rising to damaging levels • Declining systemic blood pressure causes dilation of afferent arterioles and raises glomerular hydrostatic pressure • Both responses help maintain normal NFP and GFR 31 TUBULOGLOMERULAR FEEDBACK MECHANISM • Autoregulation by the flow-dependent tubuloglomerular feedback mechanism is “directed” by the macula densa cells of the juxtaglomerular complex. These cells, located in the walls of the ascending limb of the nephron loop, respond to filtrate NaCl concentration (which varies directly with filtrate flow rate). • When GFR increases, there is not enough time for reabsorption and the concentration of NaCl in filtrate remains high. The macula densa cells respond to high levels of NaCl in filtrate by releasing vasoconstrictor chemicals (ATP and others) that cause intense constriction of the afferent arteriole, reducing blood flow into the glomerulus 32 TUBULOGLOMERULAR FEEDBACK MECHANISM • This drop in blood flow decreases the NFP and GFR, slowing the flow of filtrate and allowing more time for filtrate processing (NaCl reabsorption) • On the other hand, the low NaCl concentration of slow flowing filtrate inhibits ATP release from macula densa cells, causing vasodilation of the afferent arterioles. This allows more blood flow into the glomerulus, thus increasing NFP and GFR 33 REGULATION OF GLOMERULAR FILTRATION • Autoregulatory mechanisms maintain a relatively constant GFR over a mean arterial pressure range of about 80 – 180 mmHg • Consequently, normal day-to-day changes in our blood pressure (such as during exercise, sleep or changes in posture) do not cause large changes in water or solute excretion • However, the intrinsic controls cannot handle extremely low systemic blood pressure, such as might result from serious haemorrhage (hypovolaemic shock). Once mean arterial pressure drops below 80 mmHg, autoregulation ceases and extrinsic controls take over 34 EXTRINSIC CONTROLS: NEURAL AND HORMONAL MECHANISMS • The purpose of extrinsic controls regulating the GFR is to maintain systemic blood pressure 1. Sympathetic nervous system controls – Neural renal controls serve the needs of the body as a whole – sometimes to the detriment of the kidneys. When the volume of the extracellular fluid is normal and the sympathetic nervous system is at rest, the renal blood vessels are dilated and renal autoregulation mechanisms prevail. However, when the extracellular fluid volume is extremely low (as in hypovolaemic shock during severe haemorrhage) it is necessary to shunt blood to vital organs and neural controls may override autoregulatory mechanisms. This could reduce renal blood flow to the point of damaging the kidneys. When blood pressure falls, norepinephrine released by sympathetic nerve fibres (and epinephrine released by the adrenal medulla) causes vascular smooth muscle to constrict, increasing peripheral resistance and bringing blood pressure back up toward normal. As part of this reflex, the afferent arterioles also constrict. Constriction of the afferent arterioles decreases GFR and so helps to restore blood volume and blood pressure to normal 35 EXTRINSIC CONTROLS: NEURAL AND HORMONAL MECHANISMS 2. Renin-angiotensin-aldosterone mechanism – This is the body’s main mechanism for increasing blood pressure. |Low blood pressure causes the granular cells of the juxtaglomerular complex to release renin 36 REGULATION OF GLOMERULAR FILTRATION 37 URINE FORMATION – STEP 2: TUBULAR REABSORPTION • Tubular reabsorption is a selective transepithelial process that begins as soon as the filtrate enters the proximal tubules • Given healthy kidneys, virtually all organic nutrients such as glucose and amino acids are completely reabsorbed to maintain or restore normal plasma concentrations. On the other hand, the reabsorption of water and many ions is continuously regulated and adjusted in response to hormonal signals 38 URINE FORMATION – STEP 2: TUBULAR REABSORPTION • Tubular reabsorption of sodium – Sodium reabsorption is almost always active and via the transcellular route • Tubular reabsorption of nutrients, water and ions – The reabsorption of sodium by primary active transport provides the energy and the means for reabsorbing almost every other substance, including water 39 TRANSPORT MAXIMUM • The transcellular transport systems for the various solutes are quite specific and limited • There is a transport maximum (Tm) for nearly every substance that is reabsorbed using a transport protein in the membrane • The Tm (reported in mg/min) reflects the number of transport proteins in the renal tubules available to ferry a particular substance • In general, there are plenty of transporters (and therefore high Tm values) for substances such as glucose that need to be retained, and few or no transporters for substances of no use to the body 40 TRANSPORT MAXIMUM • When transporters are saturated – that is, all bound to the substance they transport – the excess is excreted in urine • This is what happens in individuals who become hyperglycaemic because of uncontrolled diabetes mellitus • As plasma levels of glucose approach 10 mmol/L, the glucose Tm is exceeded and large amounts of glucose may be lost in the urine even though the renal tubules are still functioning normally 41 REABSORPTIVE CAPABILITIES OF THE RENAL TUBULES AND COLLECTING DUCTS • Proximal Convoluted Tubule (PCT) – The entire renal tubule is involved in reabsorption to some degree, but the PCT cells are by far the most active “reabsorbers” and the events just described occur mainly in this tubular segment. Normally, the PCT reabsorbs all of the glucose and amino acids in the filtrate and 65% of the sodium and water. |The bulk of the electrolytes are reabsorbed by the time the filtrate reaches the nephron loop. Nearly all of the uric acid and about half of the urea are reabsorbed in the proximal tubule, but both are later secreted back into the filtrate 42 REABSORPTIVE CAPABILITIES OF THE RENAL TUBULES AND COLLECTING DUCTS • Nephron Loop – Beyond the PCT, the permeability of the tubule epithelium changes dramatically. Water can leave the descending limb of the nephron loop but not the ascending limb, where aquaporins are scarce or absent in the tubule cell membranes. The opposite is true for solutes. Virtually no solute reabsorption occurs in the descending limb, but solutes are reabsorbed both actively and passively in the ascending limb 43 REABSORPTIVE CAPABILITIES OF THE RENAL TUBULES AND COLLECTING DUCTS • Distal Convoluted Tubule (DCT) and Collecting Duct – While reabsorption in the PCT and nephron loop does not vary with the body’s needs, reabsorption in the DCT and collecting duct is fine-tuned by hormones. Because most of the filtered water and solutes have been reabsorbed by the time the DCT is reached, only a small amount of filtered load is subject to this fine tuning (e.g. about 10% of the originally filtered NaCl and 25% of the water) 44 REABSORPTIVE CAPABILITIES OF THE RENAL TUBULES AND COLLECTING DUCTS • Anti-Diuretic Hormone (ADH) – ADH inhibits diuresis, or urine output. ADH makes the principal cells of the collecting ducts more permeable to water by causing aquaporins to be inserted into their apical membranes. When the body is overhydrated, extracellular fluid osmolality decreases, decreasing ADH secretion by the posterior pituitary and making the collecting ducts relatively impermeable to water. ADH also increases urea reabsorption by the collecting ducts 45 REABSORPTIVE CAPABILITIES OF THE RENAL TUBULES AND COLLECTING DUCTS • Aldosterone – Aldosterone fine-tunes reabsorption of the remaining sodium. Decreased blood volume or blood pressure, or high extracellular potassium concentration (hyperkalaemia), can cause the adrenal cortex to release aldosterone to the blood. Aldosterone targets the principal cells of the collecting duct and cells of the distal portion of the DCT. As a result, little or no sodium leaves the body in urine. Physiologically, aldosterone’s role is to increase blood volume, thereby blood pressure, by enhancing sodium reabsorption. In general, water follows sodium if aquaporins are present 46 REABSORPTIVE CAPABILITIES OF THE RENAL TUBULES AND COLLECTING DUCTS • Atrial Natriuretic Peptide (ANP) – In contrast to aldosterone, which acts to conserve sodium, ANP reduces blood sodium thereby decreasing blood volume and blood pressure. Released by cardiac atrial cells when blood volume or blood pressure is elevated, ANP exerts several effects that lower blood sodium content, including direct inhibition of sodium reabsorption at the collecting ducts • Parathyroid Hormone (PTH) – Acting primarily at the DCT, PTH increases the reabsorption of calcium 47 URINE FORMATION – STEP 3: TUBULAR SECRETION • Tubular secretion is essentially reabsorption in reverse • Tubular secretion moves selected substances (such as hydrogen, potassium, ammonium, creatinine and certain organic acids and bases) from the peritubular capillaries through the tubule cells into the filtrate. Also, some substances (such as bicarbonate) that are synthesised in the tubule cells are secreted • The urine eventually excreted contains both filtered and secreted substances. With one major exception (potassium), the PCT is the main site of excretion 48 URINE FORMATION – STEP 3: TUBULAR SECRETION Tubular secretion is important for • Disposing of substances, such as certain drugs and metabolites that are tightly bound to plasma proteins. Because plasma proteins are generally not filtered, the substances they bind are not filtered and so must be secreted • Eliminating undesirable substances or end products that have been reabsorbed by passive processes. Urea and uric acid, two nitrogenous wastes, are both handled in this way • Ridding the body of excess potassium • Controlling blood pH. When blood pH drops toward the acidic end of its homeostatic range, the renal tubule cells actively secrete more hydrogen ions into the filtrate and retain and generate more bicarbonate (a base). As a result, blood pH rises and the urine drains off the excess hydrogen ions. Conversely, when blood pH approaches the alkaline end of it range, chloride I reabsorbed instead of bicarbonate, which is allowed to leave the body in urine 49 REGULATION OF URINE CONCENTRATION AND VOLUME • The kidneys make adjustments to keep the solute concentration of body fluids constant at about 300 mOsm, the normal osmotic concentration of blood plasma. Maintaining constant osmolality of extracellular fluid is crucial for preventing cells, particularly in the brain, from shrinking or swelling from the osmotic movement of water • The kidneys keep the solute load of body fluids constant by regulating urine concentration and volume. When you dehydrate, your kidneys produce a small volume of concentrated urine. When you overhydrate, your kidneys produce a large volume of dilute urine. The kidneys accomplish this feat using countercurrent mechanisms 50 REGULATION OF URINE CONCENTRATION AND VOLUME • Two types of countercurrent mechanisms determine urine concentration and volume • Countercurrent multiplier – Is the interaction between the flow of filtrate through the ascending and descending limbs of the long nephron loops of juxtamedullary nephrons • Countercurrent exchanger – Is the flow of blood through the ascending and descending portions of the vasa recta • These countercurrent mechanisms establish and maintain an osmotic gradient extending from the cortex through the depths of the medulla. This gradient – the medullary osmotic gradient – allows the kidneys to vary urine concentration dramatically 51 REGULATION OF URINE CONCENTRATION AND VOLUME 52 REGULATION OF URINE CONCENTRATION AND VOLUME 53 REGULATION OF URINE CONCENTRATION AND VOLUME 54 REGULATION OF URINE CONCENTRATION AND VOLUME 55 THE COUNTERCURRENT MULTIPLIER • The countercurrent multiplier depends on actively transporting solutes out of the ascending limb • Although the two limbs of the nephron limb are not in direct contact with each other, they are close enough to influence each other’s exchanges with the interstitial fluid they share • The more NaCl the ascending limb extrudes, the more water diffuses out of the descending limb and the saltier the filtrate in the descending limb becomes. The ascending limb then uses the increasingly “salty” filtrate left behind in the descending limb to raise the osmolality of the medullary interstitial fluid even further. This establishes a positive feedback cycle that produces the high osmolality of the fluids in the descending limb and interstitial fluid 56 THE COUNTERCURRENT EXCHANGER • The vasa recta act as countercurrent exchangers • Countercurrent exchange does not create the medullary gradient, but preserves it 1. By preventing rapid removal of salt from the medullary interstitial space 2. By removing reabsorbed water • As a result, blood leaving and re-entering the cortex via the vasa recta has nearly the same solute concentration • Water picked up by the ascending vasa recta includes not only water lost from the descending vasa recta, but also water reabsorbed from the nephron loop and collecting duct. As a result, the volume of blood at the end of the vasa recta is greater than at the beginning 57 FORMATION OF DILUTE OR CONCENTRATED URINE 58 FORMATION OF DILUTE OR CONCENTRATED URINE • Without the medullary osmotic gradient you would not be able to raise the concentration of urine above 300 mOsm – the osmolality of interstitial fluid. As a result, you would not be able to conserve water when you are dehydrated • When we are overhydrated, ADH production decreases and the osmolality of urine falls as low as 100 mOsm. If aldosterone is present, the DCT and collecting duct cells can remove sodium and selected other ions from the filtrate, making the urine that enters the renal pelvis even more dilute. The osmolality of urine can plunge as low as 50 mOsm, about one-sixth the concentration of glomerular filtrate or blood plasma 59 FORMATION OF DILUTE OR CONCENTRATED URINE • When we are dehydrated, the posterior pituitary releases large amounts of ADH and the solute concentration of urine may rise as high as 1200 mOsm, the concentration of interstitial fluid in the deepest part of the medulla. • With maximal ADH secretion, up to 99% of the water in filtrate is reabsorbed and returned to the blood, and only half a litre per day of highly concentrated urine is excreted. The ability of our kidneys to produce such concentrated urine is critically tied to our ability to survive without water 60 FORMATION OF DILUTE OR CONCENTRATED URINE 61 UREA RECYCLING AND THE MEDULLARY OSMOTIC GRADIENT • We usually think of urea as simply a metabolic waste product, but conserving water is important that the kidneys actually use urea to help form the medullary gradient 1. Urea enters the filtrate by facilitated diffusion in the ascending thin limb of the nephron loop 2. As the filtrate moves on, the cortical collecting duct usually reabsorbs water, leaving urea behind 3. When filtrate reaches the portion of the collecting duct in the deep medullary region, urea, now highly concentrated moves by facilitated diffusion out of the tubule into the interstitial fluid of the medulla. These movements of urea form a pool of urea that recycles back into the ascending thin limb of the nephron loop and contributes substantially to the high osmolality in the medulla 62 UREA RECYCLING AND THE MEDULLARY OSMOTIC GRADIENT • ADH enhances urea transport out of the medullary collecting duct. When ADH is present, it increases urea recycling and strengthens the medullary osmotic gradient allowing more concentrated urine to be formed 63 DIURETICS • Alcohol, essentially a sedative, encourages diuresis by inhibiting release of ADH • Other diuretics increase urine flow by inhibiting sodium reabsorption and the obligatory water reabsorption that normally follows • Examples include many drugs prescribed for hypertension or the oedema of congestive heart failure • Most diuretics inhibit sodium-associated symporters. “Loop diuretics” such as furosemide (Lasix) are powerful because they inhibit formation of the medullary gradient by acting at the ascending limb of the nephron loop. Thiazides are less potent and act at the DCT 64 RENAL CLEARANCE • Renal clearance refers to the volume of plasma from which the kidneys clear (completely remove) a particular substance in a given time, usually expressed as a one minute interval • Renal clearance tests are done to determine the GFR, which allows for the detection of glomerular damage and follow the progress of renal disease 65 RENAL CLEARANCE • The renal clearance rate (C) of any substance, in mL/min, is calculated from the equation C = UV/P • Where • U = Concentration of the substance in urine (mg/mL) • V = Flow rate of urine formation (mL/min) • P = Concentration of substance in plasma • Because it is freely filtered and neither reabsorbed nor secreted by the kidneys, insulin is the standard used to determine GFR 66 RENAL CLEARANCE • The clearance value tells us about the net handling of a substance by the kidneys. There are three possible cases • A clearance value of less than that of insulin means that the substance is reabsorbed. For example, urea has a C of 70 mL/min, meaning that of the 125 mL of glomerular filtrate formed each minute, approximately 70 mL is completely cleared of urea, while the urea in the remaining 55 mL is recovered and returned to the plasma. If the C is zero, such as for glucose in healthy individuals, reabsorption is complete or the substance is not filtered • If the C is equal to that of insulin, there is no net reabsorption or secretion • If the C is greater than that of insulin, the tubule cells are secreting the substance into the filtrate. This is the case with most drug metabolites. Knowing a drug’s renal clearance value is essential because if it is high, the drug dosage must also be high and administered frequently to maintain a therapeutic level 67 RENAL CLEARANCE • Creatinine, which has a C of 140 mL/min, is freely filtered but also secreted in small amounts. It is often used nevertheless to give a “quick and dirty” estimate of GFR because it does not need to be intravenously infused into the patient as does insulin 68 RENAL DISEASE • Chronic renal disease, defined as a GFR of less than 60 mL/min for at least three months, often develops silently and insidiously over many years. Filtrate formation decreases gradually, nitrogenous wastes accumulate in the blood and blood pH drifts toward the acidic range • The leading cause of chronic renal disease is diabetes mellitus (44% of new cases), with hypertension a close second (28% of new cases). Other causes include repeated kidney infections, physical trauma and chemical poisoning by heavy metals 69 RENAL DISEASE • In renal failure (GFR < 15 mL/min), filtrate formation decreases or stops completely. The clinical syndrome associated with renal failure is called uraemia (literally “urine in the blood”) and includes fatigue, anorexia, nausea, mental changes and muscle cramps 70 URINE – COLOUR AND TRANSPARENCY • Freshly voided urine is clear and pale to deep yellow. Its yellow colour is due to urochrome, a pigment that results when the body destroys haemoglobin. The more concentrated the urine, the deeper the colour • An abnormal colour (such as pink, brown or a smoky tinge) may result from eating certain foods (beetroot or rhubarb) of from the presence of bile pigments or blood in the urine. Additionally, some commonly prescribed drugs and vitamin supplements alter the colour of urine • Cloudy urine may indicate a urinary tract infection 71 URINE – CHEMICAL COMPOSITION • Water accounts for about 95% of urine volume; the remaining 5% consists of solutes • The largest component of urine by weight, apart from water, is urea, which is derived from the normal breakdown of amino acids • Other nitrogenous wastes in urine include uric acid (an end product of nucleic acid metabolism) and creatinine (a metabolite of creatine phosphate, which is found in large amounts in skeletal muscle tissue where it stores energy to regenerate ATP) 72 URINE – CHEMICAL COMPOSITION Normal solute constituents of urine, in order of descending concentration are • Urea • Sodium • Potassium • Phosphate • Sulphate • Creatinine • Uric acid 73 URINE – CHEMICAL COMPOSITION • Much smaller but highly variable amounts of calcium, magnesium and bicarbonate are also present • Unusually high concentrations of any solute, or the presence of abnormal substances such as blood proteins, WBCs (pus) or bile pigments, may indicate pathology 74 URINE TRANSPORT, STORAGE AND ELIMINATION • The kidneys form urine continuously and the ureters transport it to the bladder • It is usually stored in the bladder until its release through the urethra is convenient, a process called micturition • The ureters are slender tubes that convey urine from the kidneys to the bladder • Each ureter begins at the level of L2 as a continuation of the renal pelvis. From there, it descends behind the peritoneum and runs obliquely through the posterior bladder wall • This arrangement prevents backflow of urine because any increase in bladder pressure compresses and closes the distal ends of the ureters 75 URINE TRANSPORT, STORAGE AND ELIMINATION • The ureter plays an active role in transporting urine • Incoming urine distends the ureter and stimulates its muscularis to contract, propelling urine into the bladder – urine does not reach the bladder through gravity alone • The strength and frequency of peristaltic waves are adjusted to the rate of urine formation 76 URINE TRANSPORT, STORAGE AND ELIMINATION • On occasion, calcium, magnesium or uric acid salts in urine may crystallise and precipitate in the renal pelvis, forming renal calculi, or kidney stones • Most calculi are under 5mm in diameter and pass through the urinary tract without causing problems. However, larger calculi can obstruct a ureter and block urine drainage. Increasing pressure in the kidney causes excruciating pain 77 ABNORMAL URINARY CONSTITUENTS 78 URINARY BLADDER ANATOMY • The bladder is located retroperitoneally on the pelvic floor just posterior to the pubic symphysis • The prostate (part of the male reproductive system) lies inferior to the bladder neck, which empties into the urethra • In females, the bladder is anterior to the vagina and uterus • The interior of the bladder has openings for both ureters and the urethra. The smooth, triangular region of the bladder base outlined by these three openings is the trigone, important clinically because infections tend to persist in this region 79 URINE STORAGE CAPACITY • The bladder is very distensible and uniquely suited for its function of urine storage • When empty, the bladder collapses into its basic pyramidal shape and it walls are thick and thrown into folds (rugae) • As urine accumulates, the bladder expands, becomes pear-shaped, and rises superiorly in the abdominal cavity. The muscular wall stretches and thins, and rugae disappear. These changes allow the bladder to store more urine without a significant rise in internal pressure 80 URETHRA • The urethra is a thin-walled muscular tube that drains urine from the bladder and conveys it out of the body • At the bladder-urethra junction, the detrusor smooth muscle thickens to form the internal urethral sphincter. This involuntary sphincter, controlled by the autonomic nervous system, keeps the urethra closed when urine is not being passed and prevents leaking between voiding • The external urethral sphincter surrounds the urethra as it passes through the urogenital diaphragm. This sphincter is formed of skeletal muscle and is voluntarily controlled. The levator ani muscle of the pelvic floor also serves as a voluntary constrictor of the urethra 81 URETHRA • The length and functions of the urethra differ in the two sexes. In females the urethra is only 3 – 4 cm long and fibrous connective tissue binds it tightly to the anterior vaginal wall. Its external opening, the external urethral orifice, lies anterior to the vagina opening and posterior to the clitoris 82 URETHRA • In males the urethra is approximately 20 cm long and has three regions • The prostatic urethra, about 2.5cm long, runs within the prostate • The intermediate part of the urethra (or membranous urethra), which runs through the urogenital diaphragm, extends about 2cm from the prostate to the beginning of the penis • The spongy urethra, about 15cm long, passes through the penis and opens at its tip via the external urethral orifice • The male urethra has a double function: it carries semen as well as urine out of the body 83 POLYCYSTIC KIDNEY DISEASE (PKD) • PKD is a group of disorders characterised by the presence of many fluid-filled cysts in the kidneys. These interfere with renal function, ultimately leading to renal failure. These disorders can be grouped into two general forms: • Autosomal Dominant PKD – The less severe form, is much more common, affecting 1 in 500 people. The cysts develop so gradually that they produce no symptoms until about 40 years of age. Then both kidneys begin to enlarge as blister-like cysts containing fluid accumulate. The damage caused by these cysts progresses slowly, and many victims live without problems until their 60’s. Ultimately, however, the kidneys become “knobby” and grossly enlarged, reaching a mass of up to 14kg each • Autosomal Recessive PKD – Is more severe and much less common, affecting 1 in 20,000 people. Almost half of newborns with recessive PKD die just after birth, and survivors generally develop renal failure in early childhood 84 85 85