Global Species Richness & Biodiversity - BIOB50 PDF
Document Details
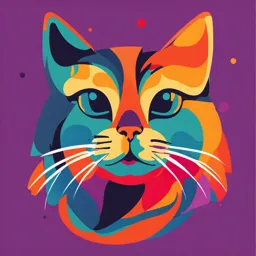
Uploaded by JollyBoltzmann7380
University of Toronto
Tags
Related
- Extinction of Species PDF
- Endangered Species: The Polar Bear PDF
- Biogeography: Landscape Ecology and Global Biodiversity PDF
- Global Fisheries for Tuna and Other Large Pelagic Species PDF
- Addison Learn - Unit 9 Global Change Exam Review PDF
- BIOB50H3F Ecology Biodiversity & Biogeography Fall 2024 Lecture 10 PDF
Summary
This document discusses global species richness, challenges in estimating species richness, species-abundance relationships, quantifying diversity using indices like the Shannon-Wiener Diversity Index, phylogenetic diversity, and functional diversity. It also examines species richness across different ecosystems and geographic areas.
Full Transcript
Week 10 | Day 1 | BIOB50 Global Species Richness On this plane we have ~5 million to several billions species: 52.6% - Insects (1,000,000) 18.9% - Other invertebrates (360,000) 16.3% - Plants (310,000) 5.2% - Fungi (100,000) 3.5% - Unicellular organisms (70,000) 3.4% - Chord...
Week 10 | Day 1 | BIOB50 Global Species Richness On this plane we have ~5 million to several billions species: 52.6% - Insects (1,000,000) 18.9% - Other invertebrates (360,000) 16.3% - Plants (310,000) 5.2% - Fungi (100,000) 3.5% - Unicellular organisms (70,000) 3.4% - Chordates (60,000) Challenges of Estimating Global Species Richness 1) Reliance on physical + morphological characteristics Historically we used physical/morphological characteristics to define species Morphs of a single species may be mistaken and described as different species (eg. we’re not different species because we’re different races) Hard to determine sub-species 2) Biases exist with which species are studied We put in different levels of effort depending on what things we like to research ○ Eg. it’s more rare to find a new animal compared to a fungus (because finding a new species of animal is rarer, and would more likely be on the news etc.) Also applies to specific ecosystems ○ Studying a rainforest? Great! Go be Tarzan. Deep sea? That’s expensive. People don’t wanna be like the OceanGate billionaire. 3) Many species hard to find Researchers are constricted by logistic, time, and funding constraints 4) Many species are going extinct before they are described We are in the middle of the 6th mass extinction Determining which species are present in a community can be challenging; recording all species in a community is typically impossible Sampling techniques for determining community composition are similar to those discussed in Lecture 4 for populations, such as sampling plots, line transects, and camera traps Species-Abundance Relationships Rarity imposes challenges for sampling, as rare species are rarely sampled and may, thus, be missed when documenting community composition ○ Eg. If you knew nothing about Toronto and went out sampling, you may think there’s only raccoons here, but we know that there’s also coyotes, skunks, etc, but how often do you see those? In most communities, a few species account for most individuals; most species can be considered rare Given that we do not know the true state of a community, how do we know when our sampling efforts have captured a community’s species richness and composition adequately? Species accumulation curve: describe how the number of species that were identified increases with the number of individuals sampled ○ Species accumulation curves are initially steep but then level off once most species have been identified (the more you sample, the less likely it is that you see something new). Leveling off of the species accumulation curve can indicate adequate representation of the community in the samples Hierarchical sampling designs: collecting data at multiple spatial scales at randomly chosen locations ○ Useful because we can’t sample every area of an ecosystem either ○ Allows understanding how community composition might vary across different areas Species-Area Relationships Species-area relationships: suggest that the number of species that are found will increase with the area that is sampled ○ Steeply first, and then more slowly as the probability increases that sampled species have already been observed in previous areas Species-area relationships can be described using a power function: 𝑧 𝑆 = 𝑐𝐴 Log-log version: 𝑙𝑜𝑠 𝑆 = 𝑙𝑜𝑔 𝑐 + 𝑧 𝑙𝑜𝑔 𝐴 Where: A = area S = number of species in the area c = average number of species per unit area z = slope of log-log transformed species-area relationship (aka informing on how quickly new species area accumulated when new areas are added) Quantifying Diversity Species richness: the number of species in a community Species evenness: describes how evenly individuals are spread among species (by considering the relative abundances of each species compared to one another) ← Both communities have the same species richness. However, community B has a higher species evenness, and therefore, more diverse Shannon-Wiener Diversity Index Shannon-Wiener diversity index: a summary metric for species diversity that incorporates both species richness and species evenness (aka a measurement tool for species diversity) Where: S = number of species in the community pi = proportion of individuals that belong to the ith species Properties of the Shannon-Wiener Diversity index: ○ For a community that contains exactly one species, H=0. ○ For a fixed species richness (S), increasing evenness increases H. ○ For a fixed evenness (e.g., pi = 1/S for all species i), increasing species richness (S) increases H. ○ A higher H indicates a higher diversity, accounting for both richness and evenness. ○ A higher H indicates a higher diversity, but it does not inform on whether this is because of high richness or evenness ← Work through this example I promise doing this table is easier than memorizing that above formula (more examples on slide 28) Rank Abundance Curves Rank abundance curves: used to understand whether differences in diversity are due to differences in species richness, evenness, or both ○ It plots the proportional abundance of each species (pi) relative to the others in rank order ○ Steeper curve = more unequal community ○ Longer length of curve = more species-rich Always note: two communities could have identical species richness and evenness but completely different species compositions and functions Seven Forms of Raity Defined By Three Axes Defining Biodiversity Three definitions/categories of biodiversity: 1. The total number of species on Earth (global species richness) 2. The evolutionary diversity represented by these species 3. The diverse communities and ecosystems that these species build Phylogenetic Phylogenetic trees: graphically depict the evolutionary relationships among a set of species ○ Length of branches typically represent the time since evolutionary divergence from the previous node Phylogenetic diversity (PD): a way to measure how different species are from each other in terms of their evolutionary history ○ Can be measured by adding up all branch lengths within a phylogeny to create a single number that can be compared across locations and through time ○ A set of species that are evolutionarily very different from one another will have a higher PD score that a set of closely related species ○ How is this helpful? Eg. if you want to see how thermally sensitive a parasite is, but don’t know that much information, you can look at their evolutionary history and make inferences on how sensitive they are Functional Diversity Functional traits: characteristics of species that describe their ecological roles in a community ○ Eg. Functional traits of plants are often defined based on their morphology (e.g., woodiness, leaf characteristics, height) and on how they contribute to forest canopy structure Functional diversity dendrograms: graphically depict the relationships among a set of species in terms of their functional traits ○ The total length of all the branches in the tree can be used to measure the community's functional diversity (FD) Functional richness: How many different traits are present in the community Functional evenness: How evenly the species are distributed across these traits Diversity Across Ecosystems: Alpha, Beta, & Gamma Diversity Species richness across different habitats can be described using α-, β-, and γ-diversity γ-diversity: species richness across a region made up of smaller localities ○ The average number of species α-diversity: the average number of species found within small, local-scale habitats in a region β-diversity: a measure of heterogeneity of community composition among localities. β-diversity can be defined in many ways; one of the most common definitions is β = γ / α Walking through the image: → ○ Look at the Empire Islands The letters represent different species ○ A and B comparison shows that there’s generally same amount of species (except on Maul island, where there is no A), but overall, mostly the same ○ On the alliance islands, they have lower numbers of species and they have different species among them From a conservation perspective, that matters because if you were a conservation manager, you can put your money towards saving the Kylo island because all species from the Empire Islands can be found on Kylo ○ In this context: γ-diversity for Empire islands would be 7 (7 species) and Alliance would be 12 (12 species) α-diversity would be the average number of species on Empire/Alliance island, so for empire = (6 + 5 + 7)/3 = 6, and alliance = (3 + 4 + 5)/3 = 4 β-diversity (which is γ/α) would be low for Empire islands because you have same species everywhere, and high for Alliance islands because you have very different species everywhere Geographic Diversity There are not the same number of species everywhere Trends in phylogenetic diversity tend to be similar to those in species richness. Differences between the two (e.g., areas with comparatively low species richness but high phylogenetic diversity) can indicate hotspots of evolutionary diversity (e.g., due to long and unique evolutionary histories) Latitudinal Gradients of Diversity: Hypotheses Many hypotheses have been proposed for explaining the observed latitudinal gradients in diversity, including differences between lower and higher latitudes in (A) diversification rate, (B) diversification time, and (C) primary productivity 3 hypothesis: 1. Species diversity is largest in the tropics because the tropics have a higher species diversification rate than higher-latitude areas Large, thermally stable areas result in larger population sizes and larger population ranges, which decreases each species’ extinction risk and increases the rates of speciation 2. Species diversity is larger in the tropics than in higher-latitude areas because the tropics have been climatically stable for longer periods of time, thus giving species more time to evolve It has been hypothesized that many species that are found elsewhere also originated in the tropics and then dispersed from there 3. Species diversity is larger in terrestrial tropical systems than in temperate ones, because terrestrial productivity is generally highest in the tropics High productivity promotes higher carrying capacities and thus larger population sizes and decreased extinction risks Regional Geographic Patterns Of Biodiversity More species when: ○ The mass is bigger ○ It’s closer to mainland Equilibrium Theory of Island Biogeography Equilibrium theory of island biogeography: the number of species on an island depends on how immigration rates and extinction rates balance against one another Rates of immigration: Ecological timescales are much faster than evolutionary timescales (aka rate of new species arrivals on an island is primarily driven by immigration from somewhere else, rather than by speciation on the island) The rate of increase at which new species arrive slows when the island’s species richness is high (aka it’s harder to find new types of immigrants when it’s already so diverse) The remoteness of an island influences immigration rates ○ Higher immigration rates for islands that are closer to a source of species, such as the mainland Rats of extinction: Higher species richness results in higher extinction rates because: ○ More species can go extinct when more species are present ○ Higher species richness leads to increased competition for limited resources, leading to smaller population sizes and higher extinction probabilities for each species Equilibrium + other notes: The equilibrium number of species on an island occurs where immigration and extinction rates balance exactly The model predicts that species richness will be highest on large islands near to the mainland, and smallest on small islands far from the mainland Turnover rates (the rates at which newly colonizing species replace newly extinct species) are predicted to be largest on small islands near the mainland, and smallest on large islands far from the mainland This theorem doesn’t apply to islands only, they can also be in island-like habitats (eg. going from one mountain peak to another is like crossing an ocean) Sample Short Answer Question 1. a. Using the Equilibrium Theory of Island Biogeography, explain why a large island that is near to the mainland will likely have a larger number of species present than a small island that is farther away from the mainland. b. Will a small island that is near to the mainland always have a larger species richness than a large island that is far from the mainland? Why / why not?