Week 1 - YR1 Lecture 1H - Blood Haemoglobin and Oxygen Transport - David Mahns 2019 PDF
Document Details
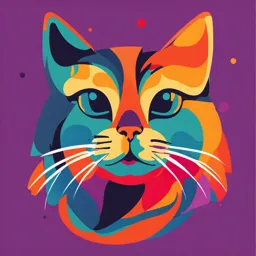
Uploaded by SoftFuturism
Western Sydney University
2019
David Mahns
Tags
Summary
This document contains lecture notes from Western Sydney University on the topic of blood, haemoglobin, and oxygen transport. The lecture notes cover the functions of blood, how oxygen is transported and blood components.
Full Transcript
Supporting cell activity: Respiratory organs and Haemoglobin Associate Professor David Mahns Director of Research Engagement School of Medicine Western Sydney University E: [email protected] Slides adapted, with thanks, from the original lecture delivered by Prof Neville Yeomans Dr Saad N...
Supporting cell activity: Respiratory organs and Haemoglobin Associate Professor David Mahns Director of Research Engagement School of Medicine Western Sydney University E: [email protected] Slides adapted, with thanks, from the original lecture delivered by Prof Neville Yeomans Dr Saad Nagi Blair Munford De Humani Corporis Fabrica Andreas Vesalius, 1543 1 Learning Objectives Understand why multicellular organisms face different challenges for survival than unicellular organisms Know the basic functions of the respiratory system, the relationship between structure and function, and its fundamental role in supporting cell activity elsewhere in the body Know the basic functions of the cardiovascular system in the transport of oxygen, and its fundamental role in supporting cell activity elsewhere in the body. 2 Learning Objectives Describe the main constituents and functions of blood Draw a normal oxyhaemoglobin dissociation curve and identify the normal arterial and mixed venous points. Explain how oxygen is transported to cells of the body via attachment to haemoglobin in red cells. Give normal values for haematocrit, haemoglobin, blood volume, arterial oxygen saturation and oxygen content. Identify that without a nucleus, red cells cannot make new enzymes or replace damaged haemoglobin and components of their membranes. 3 4 5 What do cells need to survive? Chemical building blocks – Carbohydrates, Protein, Lipids (Fats, oils, waxes, steroids), Nucleic Acids Energy sources – Molecules ‘burn’ to make energy – Oxygen http://www.fas.org 6 What’s inside a human cell? THE PLANS Mitochondria FACTORY Endoplasmic Reticulum Nucleus Golgi Apparatus 7 The mitochondrion First discovered in the nineteenth century, and was dubbed ‘bioblast’ Requires pyruvic acid (made from glucose in cytoplasm), free fatty acids or amino acids Needs O2 Makes the energy currency used in cells, ATP (Adenosine Triphosphate) Makes waste product (CO2) Image courtesy N. Yeomans 8 Why being one cell big is helpful Oxygen need only diffuse a short distance from the cell membrane to where it is needed Carbon dioxide need only diffuse a short distance to exit the cell Absorb small molecules from their environment Ingest ‘food’ by engulfing a smaller creature and digesting them in an internal vacuole 9 Mechanism for efficiently taking in O2 and excreting CO2 (Respiratory System) Tubes to carry gases in and out of body Numerous diffusing chambers (alveoli) with thin membranes, close to small blood vessels, so O2 can diffuse into the blood and CO2 can diffuse out A bellow mechanism to suck air in, and expel it with waste gases 10 How the bellows work During Inspiration, the volume of the chest cavity increases due to active – Elevation of the ribs and – Contraction (downward flattening) of diaphragm During Expiration, the volume of the chest cavity decreases due to passive – Relaxation (upward bulging) of diaphragm – Ribs return to normal position < 50 % -- Surface tension of water-air interface > 50 % 11 Cells making up the alveolus (functional unit of the lung) Fibroblast (wall) (surfactant) http://education.vetmed.vt.edu Type I cells, which are very thin squamous cells that form most of the wall of the alveolus and across which oxygen diffuses; Type II cells, which secrete surfactant; Macrophages, which enter the alveoli from the blood; Fibroblasts, which are found in the interstitium; Capillary (squamous) endothelial cells, which carry blood around the periphery of each alveolus. For clarity in the diagram, the thicknesses of the type I cells and the capillary endothelial cells are exaggerated by about 3X. The actual distance gas must diffuse between the alveolar airspace and blood for the most part is less than about 0.5 µm. 12 Surface Tension 13 Surfactant (a critical concept) In the same way that water forms a drop, alveoli without surfactant try to close. 14 15 16 17 Lung of a baby with hyaline membrane disease (not enough surfactant) Pathguy.com 18 Comparative chest x-rays CXR of a newborn baby with hyaline membrane disease (lung fields are hazy because alveoli are filled with exudate and cells) Normal CXR of a two hour old newborn 19 Images courtesy N. Yeomans and scielo.br The Circulation: an engineering rationale Larger organisms have both a reduced surface area to volume ratio & need to transport substances over greater distances. Diffusion is distance limited Some vital substances, e.g., Oxygen are poorly soluble in water.˙. Need a specialised transport system. The Circulation: an engineering design analysis REQUIREMENTS: 1. Pump - Heart 2. Energy source for pump - Intrinsic combustion of O2/nutrients 3. Pipes to/from appropriate points - arteries & veins to/from all organs 4. Interface/exchange systems - (A) Lung; - (B) Tissue capillaries 5. An appropriate fluid - BLOOD FUNCTIONS OF BLOOD Oxygen transport Nutrient transport Carbon dioxide transport Waste product transport Humeral messaging (hormone transport) Counter infection/immunological roles Systemic/self repair – i.e. coagulation The percentage of the blood that is made up of the red cells is called the haematocrit* Normal value is between 35 & 50% Components of blood The green topped tube contains mixed whole blood The white topped tube has been centrifuged into 3 layers: Top – plasma Middle - “buffy coat” of white cells & platelets. (In the tube it appears bigger than it really is as it also contains a separating gel) Bottom: red blood cells. They have a higher density due to their iron content, so settle to the bottom. BLOOD CONSTITUENTS A. PLASMA: Water & salts – i.e. ECF (isotonic* fluid) Proteins – roles include: – Transport – e.g. albumin (carries free fatty acids & other lipid soluble compounds) – Immunoglobulins – Coagulation (& fibrinolytic) factors – Oncotic pressure B. CELLULAR Erythrocytes* – a.k.a. red blood cells – OXYGEN CARRIERS Leukocytes* – a.k.a. white blood cells – Five types – Inflammatory & immune roles Platelets – Cellular fragments – Clot formers Vaguely relevant trivia The first commercial oral isotonic fluid was developed for the University of Florida’s college football team. Because of the Florida climate, the footballers rapidly got dehydrated at training. Physiologists developed a balanced solution of water, salts & glucose. The football team was called the Florida ‘Gators (short for alligators) so the drink was marketed as “GATORADE” Blood: The numbers Blood volume ≈ 5L or 70mls/kg ≈ 3L plasma (≈ 65g/L plasma proteins) ≈ 2L red cells (4-6 billion per ml) Haematocrit* ≈ 40% White cells: 3-10 million per ml Platelets: 150-400 million per ml – Vital role in haemostasis* & coagulation* The clotting cascade: THE BAD NEWS: You will have to know this in detail one day THE GOOD NEWS: Today is not that day Erythrocytes (Red Cells) : ≈ 25,000,000,000,000 in a 70kg adult Produced in bone marrow – Skull, vertebrae, sternum, ribs, pelvis in adult – Also femur & tibia in adolescent/child – Predominantly by liver & spleen in fetus By division from stem cells \ Stimulated by erythropoetin* Erythrocytes (Red blood cells) “Flying saucers of the bloodstream” Disc shaped: 8 μm (microns) x 2.5 μm Lifespan 100-120 days (normal cell) Destroyed by macrophages in spleen No nucleus, mitochondria or endoplasmic reticulum (ER) in mature RBCs This means Glycolytic* metabolism only Maintenance of discoid shape requires energy So why do it ? It maximises surface area & minimises distance to enhance diffusion of oxygen. Erythrocytes (Red blood cells) II "A bag of red goodies” Contains haemoglobin* (Hb) 25% of cytoplasmic volume is Hb This equates to ≈ 340g/L Oxyhaemoglobin has a red colour. Deoxyhaemoglobin has a blue-ish colour – cyanosis* “Haemoglobin: Smarter than your Heterotetramer: average protein” Adult form 2 Alpha chains Goblin fold (ά helix) Porphyrin ring 2+ Central Fe ion 2 Beta chains Goblin fold (ά helix) Porphyrin ring Central Fe ion Infants 2 α chains 2 γ chains Haemoglobin Oxygen carrying compound Contains iron within heme* molecule 4 heme + 4 peptide chains (2α, 2β) → 1x haemoglobin (Hb) Carries 1 molecule O2 per heme molecule i.e. 4 x O2 per Hb compound unit = 1.34 mls O2 per gram of Hb Oxygen & Haemoglobin O2 binding depends on pO2 - partial pressure* of oxygen Oxygen carriage not linear but sigmoid* This is the “Oxy-haemoglobin dissociation curve”* Oxygen & Haemoglobin ````` Arterial saturation Max 97-98% Mixed venous (at rest) (Venous blood returning to heart) - P02 40 mmHg - 75% Saturated ‘reserve’ capacity at rest P50 = 27 mmHg Haemoglobin again Variable O2 affinities Bohr effect* – shifting of O2 affinity with varying CO2 levels - ↑PC02 ↓ O2 affinity / Right shift in O2-Hb Curve CO2 transport Favours O2 unloading in peripheral tissue Haldane effect* – Deoxygenated haemoglobin has a higher affinity (~3.5 x) for CO2 than does oxyhaemoglobin. Favours CO2 loading in peripheral tissue Also has role in acid-base buffering Oxygen & Haemoglobin Bohr effect* Oxygen & Haemoglobin Increased CO2 carriage with decreased O2 Saturation “Decline & fall of the erythrocyte” No synthetic metabolic capability With aging: - increased fragility - decreased flexibility - tendency to spherocytosis Finally destroyed by reticulo-endothelial system (Spleen/liver) Lifespan approx 120 days i.e. ≈ 1% erythrocyte replacement/day. As erythrocytes get older they become less flexible, frailer, & tend to become spherical...... As people often do, too – but why is this? The ball requires energy input to generate a partial vacuum inside & produce this flattened shape. If this energy is lost...... the ball resumes a spherical shape – the natural conformation for a membrane – as exemplified by a soap bubble. This happens to red cells not only with age, but with metabolic disorders also. Because erythrocytes are the only cells that rely exclusively on anaerobic glucose metabolism, they are uniquely vulnerable to disorders of the glycolytic pathway – such as the G6PD deficiency. The fate of haemoglobin The old or misshapen erythrocyte ruptures usually in spleen Heamoglobin phagocytosed by macrophages Protein component lysed into amino acids & recycled into new proteins. Iron transferred by transferrin back to bone marrow & recycled into new Hb. Porphyrin (non iron portion of heme) metabolised by macrophages to bilirubin Bilirubin metabolism & excretion Macrophages produce 'free' bilirubin (yellowish pigment) Carried bound to albumin in the blood stream to the liver Conjugated with glucuronic acid in liver to water soluble bilirubin glucuronide “conjugated bilirubin” Conjugated bilirubin (greenish pigment) excreted by liver into the bile Oxygenation of blood Air goes in & out Blood goes round & round The lung is where they meet The most critical thing the circulation does is deliver oxygen to vital tissues Without the red blood cell & its haemoglobin this cannot happen, no matter how well the heart & lungs work Source of oxygen Atmospheric pressure 760 mmHg (100kPa) Oxygen is 21% of this (dry air) Humidified air has a water vapour pressure (PvH20) of 47 mmHg – or about 6% Oxygen is 21% of the remaining 713mm (760-47) = 150 mmHg This is the inspired oxygen partial pressure, PiO2 The alveolar gas equation To work out what the O2 is in the alveoli*: PAO2 = PiO2 – PACO2/R + F Or (simplified) PAO2 ≈ PiO2 – PaCO2/R This is what we breath in This is what we want to work out This is the CO2 added from blood But what the @#$% is this?! But who is “R”? R is the Respiratory Quotient* It is the Ratio: CO2 produced/O2 used and Reflects what fuel is being metabolised for glucose C6H12O6 + 6O2 => 6CO2 + 6H2O R = CO2 /O2 = 1 (e.g. a cyclist burning energy gels & muscle glycogen) For fats (CH2)n + 1½nO2 => nCO2 + nH2O R = CO2 /O2 = 1/1.5 = 0.67 somebody on a low or zero carbs (Atkins type) diet Vaguely relevant trivia Impressive early weight loss can be seen with the Atkins & similar fad diets: but this is mainly due to depletion of muscle - and to some extent liver – glycogen and its associated water content. On the scales at least, this produces a noticeable change... just not a worthwhile one. OK, back to “R” Under most normal circumstances the value of “R” is around 0.8 – and this value is accepted in most physiological calculations. So: Oxygen consumption in the “physiological” 70kg male is about 250mls/min Multiplied by “R” gives us his CO2 production. i.e. 250 x 0.8 = 200mls (or ~0.4 grams) CO2/min Back to alveolar gas: PAO2 ≈ PiO2 – PaCO2/R = 150 – 40/0.8 = 150 – 50 ≈ 100mm Hg So what? PaO2 (arterial oxygen pressure) should be very close to alveolar pressure (PAO2)... From the oxyhaemoglobin dissociation curve we can work out oxygen saturation. Then saturation times Hb content times 1.34 gives oxygen content (mls O2/dL or/L) And finally, content times cardiac output* gives us tissue oxygen delivery in mls/min. The O2-Hb dissociation curve again (Get used to this one – you’ll see a lot of it) Working it out SaO2 should be 98-99% i.e. ≈ 100% If Hb is 150g/L O2 content is 200 mls/L If cardiac output is 5 L/min, then tissue O2 delivery is 1000mls/min. Well above consumption @ 250mls/min Which is good Any time consumption exceeds supply (with money as well as oxygen)... you have a problem. (In one case – hypoxia; in the other – overdraft)x The Oxygen Cascade Air 153mm Hg Alveolar air 103 mm Hg Arterial blood 100 mmHg Capillary blood 40 mmHg Tissues 10-30 mm Hg Mitochondria* 3-5 mmHg O2 moves down its concentration gradient. Don’t tell me that this whole system exists just to get a lousy 5mm pressure of oxygen into the mitochondria?! OK, we won’t tell him, but nonetheless - it does. CO2 Transport Simpler than O2 Near linear relationship of pCO2 & content Carried as: – Dissolved CO2 – HCO3 (Bicarbonate) – most – Bound to Hb & plasma proteins – Carbamino compounds A little exercise: Can you work out the difference between arterial and venous CO2 content? Yes you can – you just may not realise it The Fick Principle states that if you know the amount of a substance added to (or removed from) a liquid & can measure the change in concentration, you can work out the volume (or flow). In fact if you know any two of these you can work out the third. Working through it: For our 70kg man we know that: CO2 production is ~ 200mls/min (From O2 consumption of 250mls/min x R of 0.8) Cardiac output is ~ 5 litres/min Can you work it out? And the answer is: 40mls CO2 per litre – more usually expressed as 4mls per decilitre (100mls) blood Did you get it right? YES Go to the top of the class NO Go back, have a think about it, try again The erythrocyte and CO2 transport Red blood cells contain the enzyme carbonic anhydrase*, which catalyses the conversion of CO2 to bicarbonate in the capillaries - & vice versa in the lungs: CO2 + H20 ↔ H2CO3 ↔ H+ + HCO3 Carbonic↑anhydrase This enables carriage of large amounts of total CO2 – approx 50mls/100mls in blood This is an important contributor to the body’s buffering* capacity So what can go wrong? Quite a lot of things actually But the upshot of all of them is failure to get oxygen to mitochondria – i.e. Hypoxia* Includes: Oxygen not getting to blood in the lungs “HYPOXIC HYPOXIA” Blood not able to carry enough oxygen “ANAEMIC HYPOXIA” Oxygenated blood not getting to the tissues ”STAGNANT HYPOXIA” Hypoxic “blue” hypoxia Causes include Central respiratory depression e.g. narcotic overdose, high quadraplegia Loss of airway e.g. coma, sleep apnoea, upper airway swellings Insufficient O2 in inspired gas mixture e.g. aircraft decompression at altitude Insufficient O2 getting into capillary blood. e.g. pulmonary oedema, pneumonia Anaemic “pale” hypoxia Causes include: Not enough haemoglobin e.g. iron deficiency, thalassaemia Abnormal haemoglobins – Congenital e.g. sickle cell anaemia – Or acquired e.g. met-haemoglobinaemia Haemoglobin “pre-occupied” e.g. carbon monoxide poisoning Haemolysis of normal erythrocytes e.g. transfusion reaction, auto-immune haemolysis Abnormal erythrocytes with haemolysis e.g. sickle cell crisis, spherocytosis. Stagnant “grey” hypoxia (shock) Causes include Hypovolaemic e.g. blood loss, burns, dehydration Cardiac e.g. cardiac arrest, tamponade, or failure Distributive (a) Septic e.g. meningococcal septicaemia (b) Neurogenic e.g. spinal injury, vagal syncope