Week 1-Introduction to Biochemistry PDF
Document Details
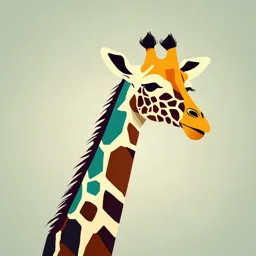
Uploaded by AffirmativeJasper2611
Fenerbahçe Üniversitesi
2023
Derya Dilek Kancağı
Tags
Summary
This document provides an introduction to biochemistry, describing its historical context, fundamental principles, including central concepts like the chemical composition of living matter, and the biochemical processes underlying life activities. It explores the evolution of biomolecules through early biochemical processes.
Full Transcript
INTRODUCTION TO BIOCHEMISTRY Assist. Prof. Dr. Derya DİLEK KANÇAĞI Room Number: 511 E-mail: [email protected] Office Hour: Wednesday 13.00-15.00 Biochemistry • The term “biochemistry” was coined in the 1870s. (the term Biology in 1800) • Biochemistry played a significant role in the advan...
INTRODUCTION TO BIOCHEMISTRY Assist. Prof. Dr. Derya DİLEK KANÇAĞI Room Number: 511 E-mail: [email protected] Office Hour: Wednesday 13.00-15.00 Biochemistry • The term “biochemistry” was coined in the 1870s. (the term Biology in 1800) • Biochemistry played a significant role in the advancement of chemistry and made an important contribution to the modernization of biology. By the mid-twentieth century, biochemistry was recognized widely as a discipline distinct from other branches of chemistry and biology. • Biochemistry in broad terms is the study of the chemical composition of the living matter and the biochemical processes that underlie life activities during growth and maintenance. Biochemistry= describes the structures, mechanisms, and chemical processes shared by all organisms SCIENCE… Although biochemistry provides important insights and practical applications in medicine, agriculture, nutrition, and industry, its ultimate concern is with the wonder of life itself. Timeline on history of biochemistry 1828 Friedrich Wöhler published a paper about the synthesis of urea, proving that organic compounds can be created artificially. 1829 In 1833, diastase (a mixtureof amylases) was the first enzyme to be discovered, quickly followed by other hydrolytic enzymes such as pepsin and invertase, but the term enzyme was only coined in 1877 by Wilhelm Kühne. 1883 Discovered in 1883, laccase is one of the first enzymes ever described. 1896 Eduard Buchner contributed the first demonstration of a complex biochemical process outside of a cell: alcoholic fermentation in cell extracts of yeast. 1953 James Watson and Francis Crick discovered the double helical structure of the DNA molecule. 1953 Hans Adolf Krebs discovers the biochemical steps of the Krebs cycle in carbohydrate metabolism. 1957 Daniel Bovet receives the Nobel Prize for the development of antihistamines. 1959 Severo Ochoa and Arthur Kornberg receive Nobel Prize for discoveries on the synthesis of DNA and RNA. 1970 Hamilton Smith reports the discovery of the first restriction enzyme. Timeline on history of biochemistry 1971 Theodor Diener demonstrates the fundamental differences between viroids and viruses. 1975 Cesar Milsein, Georges Kohler, and Niels Kai Jerne develop the technique for making monoclonal antibodies. 1977 Rosalyn S. Yalow, R.C.I. Guillemin and A.V. Schally receive the Nobel Prize for developing the radioimmunossay (RIA) techniques; and using RIA to analyze peptide hormones in the brain. 1984 Cesar Milstein, Georges J.F. Koehler and Niels Jerne receive the Nobel Prize for developing a method for the production of large quantities of monoclonal antibodies. 1995 The Food and Drug Administration approves the first protease inhibitor, a major weapon against the progression of AIDS. 1997 The first complete nucleotide sequence of all of the chromosomes of a eukaryote is reported (yeast). 1999 First evidence of dopamine release from human fetal ventral mesencephalic (hfVM) grafts. 2000 Porcine fetal ventral mesencephalic (FVM) transplantation clinical trial. 2001 NIH-funded hfVM transplant clinical trials with negative results. 2003 Carotid body clinical trial. 2005 Human fetal or adult retinal pigment epithelial (hRPE) cells linked to microcarrier clinical trial. 2009 Start of TRANSEURO clinical trial. © 2021 Macmillan Learning Central Principles in Biochemistry Principle 1 Principle 2 • Cells are the fundamental unit of life. • Cells use a relatively small set of carbonbased metabolites to create polymeric machines, supramolecular structures, and information repositories. • Although they vary in complexity and can be highly specialized for their environment or function within a multicellular organism, they share remarkable similarities. • The chemical structure of these components defines their cellular function. The collection of molecules carries out a program, the end result of which is reproduction of the program and self-perpetuation of that collection of molecules—in short, life. Central Principles in Biochemistry Principle 3 Principle 4 • Living organisms exist in a dynamic steady state, never at equilibrium with their surroundings. • Cells have the capacity for precise selfreplication and self-assembly using chemical information stored in the genome. • Following the laws of thermodynamics, living organisms extract energy from their surroundings and employ it to maintain homeostasis and do useful work. Essentially all of the energy obtained by a cell comes from the flow of electrons, driven by sunlight or by metabolic redox reactions. • A single bacterial cell placed in a sterile nutrient medium can give rise to a billion identical “daughter” cells in 24 hours. Each cell is a faithful copy of the original, its construction directed entirely by information contained in the genetic material of the original cell. On a larger scale, the progeny of vertebrate animals share a striking resemblance to their parents, also the result of their inheritance of parental genes. Central Principles in Biochemistry Principle 5 WHAT IS NEXT? • Living organisms change over time by gradual evolution. • Cellular Foundations • The result of eons of evolution is an enormous diversity of life forms, fundamentally related through their shared ancestry, which can be seen at the molecular level in the similarity of gene sequences and protein structures. • Chemical Foundations • Physical Foundations • Genetic Foundations • Evolutianary Foundations Cellular Foundations Cells are the fundamental unit of life. Cells are the fundamental unit of life. Plasma membrane = defines the periphery of the cell *Composed of lipid and protein molecules *Thin, flexible, hydrophobic barrier around the cell *Contains embedded transport proteins, receptor proteins, and membrane enzymes Cytoplasm = internal volume enclosed by the plasma membrane *Composed of the cytosol (an aqueous solution) and a variety of suspended particles (such as mitochondria, chloroplasts, ribosomes, and proteasomes) Cytosol = highly concentrated solution *Contains enzymes, RNA, amino acids, metabolites, coenzymes, and inorganic ions nucleotides, Genome = complete set of genes, composed of DNA *Bacteria and archaea (formally grouped as prokaryotes) store their genome in a nucleoid *Eukaryotes store their genome in a double membraneenclosed nucleus Cellular Dimensions Are Limited by Diffusion • Cells are microscopic: – – • Animal and plant cells: 5 to 100 μm in diameter Unicellular microorganisms: 1 to 2 μm long Upper limit of cell size is likely set by the rate of transport and the need to deliver O2 to all parts of the cell – As size increases, surface-to-volume ratio decreases Their convoluted surfaces give them a much larger surface area than a sphere of the same diameter. The smallest cells, certain bacteria known as mycoplasms, are 300 nm in diameter and have a volume of about 10−14 mL. human lymphocytes Organisms Belong to Three Distinct Domains of Life • • • Bacteria = inhabit soils, surface waters, and the tissues of other living or decaying organisms Archaea = inhabit extreme environments Eukarya = all eukaryotic organisms – more closely related to archaea than bacteria The basis for this tree is the similarity in nucleotide sequences of the ribosomal RNAs of each group. Phylogeny of the three domains of life Archaea and Bacteria Subgroups Are Distinguished by Their Habitats aerobic = plentiful supply of O2; organisms transfer electrons from fuel to O2 for energy anaerobic = devoid of O2; organisms transfer electrons to nitrate, sulfate or CO2 for energy obligate anaerobes = die when exposed to O2 facultative anaerobes = can live with or without O2 Organisms Differ Widely in Their Sources of Energy and Biosynthetic Precursors • • • • Phototrophs = trap and use sunlight Chemotrophs = derive energy from oxidation* of a chemical fuel Autotrophs = can synthesize all their biomolecules directly from CO2 Heterotrophs = require some preformed organic nutrients made by other organisms *An oxidation-reduction (redox) reaction is a type of chemical reaction that involves a transfer of electrons between two species. Oxidation is the loss of electrons during a reaction by a molecule, atom or ion. Reduction is the gain of electron during a reaction by a molecule, atom or ion. Bacteria and Archaeal Cells Share Common Features but Differ in Important Ways • Cell envelope = composed of plasma membrane, outer membrane, and peptidoglycan (high molecular weight polymer) • Gram-positive bacteria: • Colored by Gram’s stain • Thick peptidoglycan layer outside plasma membrane • Lack an outer membrane • Gram-negative bacteria: • Outer membrane composed of a lipid bilayer • Archaea: • Layer of peptidoglycan or protein confers rigidity on their cell envelopes Eukaryotic Cells Have a Variety of Membranous Organelles, Which Can Be Isolated for Study • • • • • • Mitochondria = the site of most of the energy-extracting reactions of the cell Endoplasmic reticulum and golgi complexes = play central roles in the synthesis and processing of lipids and membrane proteins Peroxisomes = site of the oxidation of verylong-chain fatty acids and detoxification of reactive oxygen species Lysosomes = filled with digestive enzymes granules or droplets containing stored nutrients, such as starch and fat Vacuoles = store large quantities of organic acids Chloroplasts = where sunlight drives the synthesis of ATP (adenosine triphosphate) in the process of photosynthesis Subcellular Fractionation of Tissue • • • First step = gently disrupt cells or tissues by physical shear to rupture the plasma membrane Second step = centrifuge the homogenate Organelles differ in size and sediment at different rates These methods were used to establish, for example, that lysosomes contain degradative enzymes, mitochondria contain oxidative enzymes, and chloroplasts contain photosynthetic pigments. The isolation of an organelle enriched in a certain enzyme is often the first step in the purification of that enzyme. The Cytoplasm Is Organized by the Cytoskeleton and Is Highly Dynamic • Cytoskeleton = three-dimensional network of protein filaments in eukaryotic cells Actin filaments Microtubules Intermediate filaments – – – • Differing in width (from about 6 nm to 22 nm), composition, and specific function. All types provide structure and organization to the cytoplasm and shape to the cell. Actin filaments and microtubules also help to produce the motion of organelles or of the whole cell. • Filaments are not permanent structures; they undergo constant disassembly into their protein subunits and reassembly into filaments. Their locations in cells are not rigidly fixed but may change dramatically with mitosis, cytokinesis, amoeboid motion, or other changes in cell shape. The Structural Organization of the Cytoplasm • • Endomembrane system = segregates specific metabolic processes and provides surfaces on which certain enzyme-catalyzed reactions occur Exocytosis and endocytosis = mechanisms of transport (out of and into cells, respectively) – Involve membrane fusion and fission – Provide paths between the cytoplasm and the surrounding medium allowing the secretion of substances produced in the cell and uptake of extracellular materials. https://doi.org/10.1016/B978-0-12-386882-4.00002-5 Cells Build Supramolecular Structures • The monomeric subunits of proteins, nucleic acids, and polysaccharides are joined by covalent bonds. In supramolecular complexes, however, macromolecules are held together largely by noncovalent interactions-much weaker, individually, than covalent bonds. • Held together by noncovalent interactions (hydrogen bonds, ionic interactions, van der waals interactions, and the hydrophobic effect) • The large numbers of weak interactions between macromolecules in supramolecular complexes stabilize these assemblies, producing their unique structures. Structural hierarchy in the molecular organization of cells. In Vitro Studies May Overlook Important Interactions among Molecules • in vitro = “in glass” • in vivo = “in the living” • Molecules may behave differently in vivo and in vitro Studying isolated cellular components in vitro simplifies the experimental system, but such study may overlook important interactions that occur in the living cell. Chemical Foundations Cells use a relatively small set of carbon- based metabolites to create polymeric machines, supramolecular structures, and information repositories. Elements Essential to Animal Life and Health “What is true of E. coli is true of the elephant.” The current understanding that all organisms share a common evolutionary origin is based in part on this observed universality of chemical intermediates and transformations, often termed “biochemical unity.” • The four most abundant elements in living organisms, in terms of percentage of total number of atoms, are hydrogen, oxygen, nitrogen, and carbon, which together make up more than 99% of the mass of most cells. They are the lightest elements capable of efficiently forming one, two, three, and four bonds, respectively; in general, the lightest elements form the strongest bonds. Elements essential to animal life and health The trace elements represent a miniscule fraction of the weight of the human body, but all are essential to life, usually because they are essential to the function of specific proteins, including many enzymes. Biomolecules Are Compounds of Carbon with a Variety of Functional Groups • The chemistry of living organisms is organized around carbon. • Carbon can form covalent single (H), double (O and N), and triple bonds, particularly with other carbon atoms. Triple bonds are rare in biomolecules. • The ability of carbon atoms to form very stable single bonds with up to four other carbon atoms is very important. • Two carbon atoms also can share two (or three) electron pairs, thus forming double (or triple) bonds. Versatility of carbon bonding Geometry of Carbon Bonding • Carbon atoms have a characteristic tetrahedral arrangement of their four single bonds • Free rotation around each single bond • Limited rotation about the axis of a double bond Common Functional Groups of Biomolecules • Most biomolecules can be regarded as derivatives of hydrocarbons, with hydrogen atoms replaced by a variety of functional groups that confer specific chemical properties on the molecule, forming various families of organic compounds. • Typical of these are alcohols, which have one or more hydroxyl groups; amines, with amino groups; aldehydes and ketones, with carbonyl groups; and carboxylic acids, with carboxyl groups Many Biomolecules Are Polyfunctional … containing two or more types of functional groups, each with its own chemical characteristics and reactions. The chemical “personality” of a compound is determined by the chemistry of its functional groups and their disposition in three-dimensional space. Cells Contain a Universal Set of Small Molecules Evolution • • Central metabolites in the major pathways occurring in nearly every cell: – Common amino acids – Nucleotides – Sugars and their phosphorylated derivatives – Mono-, di-, and tricarboxylic acids Secondary metabolites = specific to the organism • Metabolome = entire collection of small molecules in a given cell under a specific set of conditions – Metabolomics = the systematic characterization of the metabolome under very specific conditions – Such as following administration of a drug, or a biological signal such as insulin Macromolecules Are the Major Constituents of Cells • • • Macromolecules= polymers with molecular weights above ~5,000 that are assembled from relatively simple precursors – – – Proteins Nucleic acids Polysaccharides composed of monomers with molecular weights of 500 or less. Oligomers = shorter polymers Informational macromolecules = name for proteins, nucleic acids, and some oligosaccharides, given their informationrich subunit sequences • Some oligosaccharides, also serve as informational molecules. Macromolecules Are the Major Constituents of Cells Protein Macromolecules • • • Proteins = long polymers of amino acids – Can function as enzymes, structural elements, signal receptors, transporters Proteome = sum of all the proteins functioning in a cell Proteomics = the systematic characterization of this protein complement under a specific set of conditions Nucleic Acid Macromolecules • • • Nucleic acids = DNA and RNA = polymers of nucleotides – Store and transmit genetic information – Some RNA molecules have structural and catalytic roles in supramolecular complexes Genome = entire sequence of a cell’s DNA or RNA Genomics = the characterization of the structure, function, evolution, and mapping of genomes Macromolecules Are the Major Constituents of Cells Polysaccharide Macromolecules Lipid Molecules • • • Polysaccharides = polymers of simple sugars – Energy-rich fuel stores – Rigid structural components of cell walls (in plants and bacteria) – Extracellular recognition elements that bind to proteins on other cells Glycome = entire complement of carbohydrate-containing molecules • Lipids = water-insoluble hydrocarbon derivatives – Structural components of membranes – Energy-rich fuel stores – Pigments – Intracellular signals Lipidome = the lipid containing molecules in a cell Building Blocks of Biochemistry Three-Dimensional Structure Is Described by Configuration and Conformation • • • Configuration = the fixed spatial arrangement of atoms Stereoisomers = molecules with the same chemical bonds and same chemical formula Stereospecific = requiring specific conformations in the interacting molecules – Describes typical interactions between biomolecules Configuration is conferred by the presence of either (1) double bonds, around which there is little or no freedom of rotation, or (2) chiral centers, around which substituent groups are arranged in a specific orientation. The identifying characteristic of stereoisomers is that they cannot be interconverted without the temporary breaking of one or more covalent bonds. Configurations of Geometric Isomers • Geometric isomers, or cis-trans isomers = differ in the arrangement of substituent groups with respect to the double bond • Each is a well-defined compound that can be separated from the other, and each has its own unique chemical properties. A binding site (on an enzyme, for example) that is complementary to one of these molecules would not be complementary to the other, which explains why the two compounds have distinct biological roles despite their similar chemical make up. • The visual pigment in the vertebrate eye, rhodopsin, contains retinal, a vitamin A–derived lipid. In the primary event of vision, light converts one isomer of retinal to another, triggering a neuronal signal to the brain. Chiral and Achiral Molecules • Chiral centers with four different substituents = asymmetric carbons • A molecule can have 2n stereoisomers, where n is the number of chiral carbons Enantiomers and Diastereomers • • Enantiomers = stereoisomers that are mirror images of each other Diastereomers = stereoisomers that are not mirror images of each other • • Enantiomers have nearly identical chemical reactivities, but differ in optical activity A racemic mixture (equimolar solution of two enantiomers) shows no optical rotation Naming Stereoisomers Using the RS System • Each group attached to a chiral carbon is assigned a priority, where: —OCH3 > —OH > —NH2 > —COOH > —CHO > —CH2OH > —CH3 > —H For compounds with more than one chiral center, the most useful system of nomenclature is the RS system. Another naming system for stereoisomers, the D and L system. Molecular Conformation • Conformation = the spatial arrangement of substituent groups that are free to assume different positions in space • When one or more of the hydrogen atoms on each carbon is replaced by a functional group that is either very large or electrically charged, freedom of rotation around the C—C bond is hindered. Interactions between Biomolecules Are Stereospecific • When biomolecules interact, the “fit” between them is often stereochemically correct; they are complementary. • In living organisms, chiral molecules are usually present in only one of their chiral forms. For example, the amino acids in proteins occur only as their L isomers; glucose occurs only as its D isomer. Complementary fit between a macromolecule and a small molecule Biological Systems Can Distinguish Stereoisomers • Stereospecificity = the ability to distinguish between stereoisomers • A property of enzymes and other proteins and a characteristic feature of biochemical interactions. • If the binding site on a protein is complementary to one isomer of a chiral compound, it will not be complementary to the other isomer Stereoisomers have different effects in humans. Physical Foundations Living organisms exist in a dynamic steady state, never at equilibrium with their surroundings. Living Organisms Exist in a Dynamic Steady State, Never at Equilibrium with Their Surroundings • Living cells and organisms must perform work to stay alive and to reproduce themselves. • One goal of biochemistry is to understand, in quantitative and chemical terms, the means by which energy is extracted, stored, and channeled into useful work in living cells. Thermodynamics… Although the characteristic composition of an organism changes little through time, the population of molecules within the organism is far from static. • • • Small molecules, macromolecules, and supramolecular complexes are continuously synthesized and broken down Living cells maintain themselves in a dynamic steady state distant from equilibrium Maintaining steady state requires the constant investment of energy when a cell can no longer obtain energy, it dies and begins to decay toward equilibrium with its surroundings. Organisms Transform Energy and Matter from Their Surroundings • • • System = all the constituent reactants and products, the solvent that contains them, and the immediate atmosphere, in short, everything within a defined region of space. Universe = system and its surroundings Types of systems: – Isolated = system exchanges neither matter nor energy with its surroundings – Closed system = system exchanges energy but not matter with its surroundings – Open system = system exchanges both energy and matter with its surroundings • A living organism is an open system; it exchanges both matter and energy with its surroundings. • Organisms obtain energy from their surroundings in two ways: • (1) they take up chemical fuels (such as glucose) from the environment and extract energy by oxidizing them (see Box 1-3, Case 2); or • (2) they absorb energy from sunlight. Energy Transformation in Living Organisms • First law of thermodynamics: in any physical or chemical change, the total amount of energy in the universe remains constant, although the form of the energy may change This means that while energy is “used” by a system, it is not “used up”; rather, it is converted from one form into another — from potential energy in chemical bonds, say, into kinetic energy of heat and motion. Some energy transformations in living Extracting Energy from the Surroundings • Photoautotrophs: • • • Chemotrophs: Oxidation-Reduction Reactions Autotrophs and heterotrophs participate in global cycles of O2 and CO2, driven by sunlight, making these two groups interdependent Oxidation-reduction reactions = one reactant is oxidized (loses electrons) as another is reduced (gains electrons) – Describes reactions involved in electron flow Creating and Maintaining Order Requires Work and Energy Second law of thermodynamics Free Energy, G • enthalpy, H = heat content, roughly reflecting the number and kinds of bonds free energy, G, of a closed system = H – TS, where H represents enthalpy, T represents absolute temperature, and S represents entropy • • Randomness in the universe is constantly increasing Entropy, S = represents the randomness or disorder of the components of a chemical system Any change in randomness of the system is expressed as entropy change, ΔS, which by convention has a positive value when randomness increases. • • • free-energy change, ∆G = ∆H − T∆S where ∆H is negative for a reaction that releases heat, and ∆S is positive for a reaction that increases the system’s randomness • Spontaneous reactions occur when ∆G is negative Coupling Reactions • • Energy-requiring (endergonic) reactions are often coupled to reactions that release free energy (exergonic) The breakage of phosphoanhydride bonds in ATP is highly exergonic Adenosine triphosphate (ATP) provides energy Energy Coupling Links Reactions in Biology • • • The central issue in bioenergetics (the study of energy transformations in living systems) is the means by which energy from fuel metabolism or light capture is coupled to a cell’s energy requiring reactions. Free-energy change, ∆G = amount of energy available to do work – Always less than the theoretical amount of energy released because some energy is dissipated as the heat of friction. reaction 1: endergonic; ∆G1 is positive reaction 2: exergonic; ∆G2 is negative In closed systems, chemical reactions proceed spontaneously until equilibrium is reached it is not the mere breakdown of ATP that provides energy to drive endergonic reactions; rather, it is the transfer of a phosphoryl group from ATP to another small molecule (glucose in the case above) that conserves some of the chemical potential originally in ATP. reaction 3: ∆G3 is negative Energy coupling in mechanical and chemical processes Keq and ∆G° Are Measures of a Reaction’s Tendency to Proceed Spontaneously • For the reaction, aA bB cC dD • ∆G (the actual free-energy change) for any chemical reaction is a function of the standard free-energy change, ∆G° • The equilibrium constant, Keq, is given by Ceq Deq K eq a b A B eq eq c d Where [A]eq is the concentration of A, [B]eq the concentration of B, and so on, when the system has reached equilibrium. where [A]i is the initial concentration of A, and so forth; R is the gas constant; and T is the absolute temperature Reactions Can Do No Work at Equilibrium Enzymes Promote Sequences of Chemical Reactions • Enzymes = greatly enhance reaction rates of specific chemical reactions without being consumed in the process • Each enzyme catalyzes a specific reaction, and each reaction in a cell is catalyzed by a different enzyme. • • • Transition state = higher free energy than reactant or product Activation energy, ∆G‡ = difference in energy between the reactant in its ground state and its transition state (an energy barrier) The binding of enzyme to the transition state is exergonic, and the energy released by this binding reduces the activation energy for the reaction and greatly increases the reaction rate. The multiplicity of enzymes, their specificity (the ability to discriminate between reactants), and their susceptibility to regulation give cells the capacity to lower activation barriers selectively. Energy changes during a chemical reaction Macromolecules thermodynamically stable than monomeric subunits slow breakdown without enzymes Catabolism and Anabolism • Pathways = sequences of consecutive reactions in which the product of one reaction becomes the reactant in the next • Catabolism = degradative, freeenergy-yielding reactions – – Drives ATP synthesis Produces the NAD(P)H reduced electron carriers • Anabolism = synthetic pathways that require the input of energy The central roles of ATP and NAD(P)H in metabolism Metabolism • Metabolism = overall network of enzyme-catalyzed pathways, both catabolic and anabolic • Unity of life = pathways of enzyme-catalyzed reactions that act on the main constituents of cells—proteins, fats, sugars, and nucleic acids—are nearly identical in all living organisms • ATP (as well as other energetically equivalent nucleoside triphosphates) is the connecting link between the catabolic and anabolic components of this network Metabolism Is Regulated to Achieve Balance and Economy • Feedback inhibition = keeps the production and utilization of each metabolic intermediate in balance • Systems biology = tasked with understanding complex interactions among intermediates and pathways in quantitative terms Genetic Foundations Cells have the capacity for precise self-replication and self-assembly using chemical information stored in the genome. Genetic Information Is Encoded in DNA The most remarkable property of living cells and organisms is their ability to reproduce themselves for countless generations with nearly perfect fidelity. This continuity of inherited traits implies constancy, over millions of years, in the structure of the molecules that contain the genetic information. • Deoxyribonucleic acid, DNA = sequence of the monomeric subunits (deoxyribonucleotides) – encode the instructions for forming all other cellular components – provide a template to produce identical DNA molecules • Genetic Continuity Is Vested in Single DNA Molecules • DNA of an E. coli cell is a single molecule containing 4.64 million nucleotide pairs • must be replicated perfectly to give rise to identical progeny by cell division The Structure of DNA Allows Its Replication and Repair with NearPerfect Fidelity • Deoxyribonucleotides = monomeric subunit that make up the DNA polymer • • Each deoxyribonucleotide in one strand pairs specifically with a complementary deoxyribonucleotide in the opposite strand Strands are held together by hydrogen bonds Complementarity between the two strands of DNA The Linear Sequence in DNA Encodes Proteins with ThreeDimensional Structures • Native conformation = precise three-dimensional structure of protein – Crucial to protein function a DNA to RNA to protein to enzyme (hexokinase). Evolutionary Foundations Living organisms change over time by gradual evolution. Changes in the Hereditary Instructions Allow Evolution • Mutation = changes in the nucleotide sequence of DNA • Changes the instructions for a cellular component • • • • Harmful or even lethal to the new organism or cell Can be beneficial Wild type = unmutated cells Natural selection - what is sometimes summarized as “survival of the fittest.” in the course of evolution, many mutations must have been erased or written over. But DNA molecules are the best source of biological history we have. Gene duplication and mutation: one path to generate new enzymatic activities. Biomolecules First Arose by Chemical Evolution • Miller and Urey experiments found that biomolecules may have been produced near hydrothermal vents at the bottom of the sea or by the action of lightning and high temperature on gaseous mixtures The components for the first cell may have been produced near hydrothermal vents at the bottom of the sea or by the action of lightning and high temperature on simple atmospheric moleculessuch as CO2 and NH3. Black smokers. Abiotic production of biomolecules. The Role of RNA in Prebiotic Evolution RNA (ribonucleic acid) = can act as catalysts in biologically significant reactions likely played a crucial role in prebiotic evolution, both as catalyst and as information repository RNA or Related Precursors May Have Been the First Genes and Catalysts • RNA or similar molecule may have been the first gene and the first catalyst • Alternatively, simple metabolic pathways may have evolved first, perhaps at the hot vents in the ocean floor The earliest cells may have been formed by the enclosure of a selfreplicating RNA molecule within a membrane-like lipid layer. The catalytic and genetic roles played by the early RNA genome were, over time, taken over by proteins and DNA, respectively. A possible “RNA world” scenario. Biological Evolution Began More Than Three and a Half Billion Years Ago • Lipid vesicles containing organic compounds and self-replicating RNA gave rise to protocells • Protocells with the greatest capacity for selfreplication became more numerous Landmarks in the evolution of life on Earth. The First Cell Probably Used Inorganic Fuels • Earliest cells probably obtained energy from inorganic fuels, such as ferrous sulfide and ferrous carbonate • Photosynthetic processes: – Arose from evolution – Pigments capture energy of light from the sun and reduce CO2 to organic compounds • Atmosphere became richer in O2 with the rise of O2-producing photosynthetic bacteria Eukaryotic Cells Evolved from Simpler Precursors in Several Stages • Three major changes led to the evolution of eukaryotes: • • • • Evolution of the chromosome Evolution of the nucleus Formation of endosymbiotic associations between early eukaryotic cells and aerobic or photosynthetic bacteria In multicellular organisms, differentiated cell types specialize in functions essential to the organism’s survival • Acquiring greater motility, efficiency, or reproductive success than their free-living singlecelled competitors. Eukaryotic cells acquired the capacity for photosynthesis and oxidative phosphorylation from endosymbiotic bacteria. In multicellular organisms, differentiated cell types specialize in one or more of the functions essential to the organism’s survival. Evolution of Eukaryotes through Endosymbiosis Early eukaryotic cells, which were incapable of photosynthesis or aerobic metabolism, enveloped aerobic bacteria or photosynthetic bacteria to form endosymbiotic associations that eventually became permanent. Some aerobic bacteria evolved into the mitochondria of modern eukaryotes, and some photosynthetic cyanobacteria became the plastids, such as the chloroplasts of green algae, the likely ancestors of modern plant cells. Evolution of eukaryotes through endosymbiosis. Molecular Anatomy Reveals Evolutionary Relationships • When two genes share readily detectable sequence similarities (nucleotide sequence in DNA or amino acid sequence in the proteins they encode), their sequences are said to be homologous and the proteins they encode are homologs. • Gene or protein sequence similarities between organisms can determine phylogenetic relationships Functional Genomics Shows the Allocations of Genes to Specific Cellular Processes • • Genes can be grouped according to the specific process (DNA synthesis, protein synthesis, generation of ATP, and so forth) in which they function – Can approximate the proportion of the genome dedicated to a specific process – Genes involved in regulation of cellular processes tend to increase with organism complexity Housekeeping genes = expressed under all conditions, not subject to much regulation Genomic Comparisons Have Increasing Importance in Medicine • • Large-scale sequencing studies have identified many genes in which mutations correlate with a medical condition The proteins these genes encode might become the target for drugs to treat a given condition