The Seeing Brain - Chapter 7 PDF
Document Details
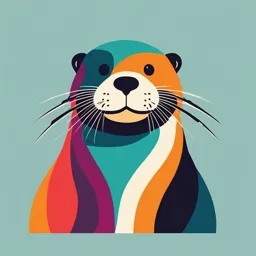
Uploaded by SteadiestTigerSEye6616
Tags
Summary
This chapter from a cognitive neuroscience textbook explores the fascinating process of vision. It describes how the brain actively constructs a visual representation of the world, going beyond a simple interpretation of retinal images. The chapter covers topics such as the geniculostriate route, receptive fields, and the primary visual cortex.
Full Transcript
CH A P TE R 7 The seeing brain CONTENTS From eye to brain 144 Cortical blindness and “blindsight” 149 Functional specialization of the visual cortex beyond V1 152 Recognizing objects...
CH A P TE R 7 The seeing brain CONTENTS From eye to brain 144 Cortical blindness and “blindsight” 149 Functional specialization of the visual cortex beyond V1 152 Recognizing objects 156 Recognizing faces 164 Vision imagined 171 Summary and key points of the chapter 172 Example essay questions 173 Recommended further reading 173 Students who are new to cognitive neuroscience might believe that the eyes do the seeing and the brain merely interprets the image on the retina. This is far from the truth. Although the eyes play an undeniably crucial role in vision, the brain is involved in actively constructing a visual representation of the world that is not a literal reproduction of the pattern of light falling on the eyes. For example, the brain divides a continuous pattern of light into discrete objects and surfaces, and translates the two-dimensional retinal image into a three-dimensional interactive model of the environment. In fact, the brain is biased to perceive objects when there is not necessarily an object there. Consider the Kanizsa illusion (Figure 7.1)—it is quite hard to perceive the stimulus as three corners as opposed to one triangle. The brain makes inferences during visual perception that go beyond the raw information given. Psychologists make a distinction between sensation and perception. Sensation refers to the effects of a stimulus on the sensory organs, whereas perception involves the elaboration and interpretation of 144 THE STUDENT’S GUIDE TO COGNITIVE NEUROSCIENCE that sensory stimulus based on, for example, knowledge of how objects are structured. This chapter will consider many examples of the constructive nature of the seeing brain, from the perception of visual attributes, such as color and motion, up to the recognition of objects and faces. F RO M EY E T O BRAIN Light is converted into neural signals by the retina, and there are several FIGURE 7.1: Do you routes by which this information is carried to the brain. Different routes automatically perceive a serve different functions. The dominant route for detailed, conscious visual white triangle that isn’t really perception in humans is the geniculostriate route. there? This is called the Kanizsa illusion. The retina K EY TERMS The retina is the internal surface of the eyes that contains specialized Sensation photoreceptors that convert (or transduce) light into neural signals. The The effects of a stimulus photoreceptors are made up of rod cells, which are specialized for low levels on the sensory organs. of light intensity, such as those found at night, and cone cells, which are more Perception active during daytime and are specialized for detecting different wavelengths The elaboration and in- of light (from which the brain can compute color). The highest concentration terpretation of a sensory of cones is at a point called the fovea, and the level of detail that can be stimulus based on, for perceived (or visual acuity) is greatest at this point. Rods are more evenly example, knowledge of distributed across the retina (but are not present at the fovea). how objects are struc- There is already a stage of neural computation that takes place at the tured. retina itself. Bipolar cells in the retina are a type of neuron that behave in one Retina of two ways: detecting light areas on dark backgrounds (ON) or detecting The internal surface of dark areas on light backgrounds (OFF). A higher level of processing, by the eyes that consists of multiple layers. Some retinal ganglion cells, has a more complex set of on and off properties. Most layers contain photore- retinal ganglion neurons have a particular characteristic response to light that ceptors that convert light is termed a center-surround receptive field. The term receptive field denotes to neural signals, and the region of space that elicits a response from a given neuron. One intriguing others consist of neurons feature of the receptive fields of these cells, and many others in the visual themselves. system, is that they do not respond to light as such (Barlow, 1953; Kuffler & Rod cells Barlow, 1953). Rather, they respond to differences in light across the center A type of photoreceptor specialized for low levels of light intensity, such as those found at night. EYE–BRAIN MYTH 1 Cone cells A type of photoreceptor Do not make the mistake of believing that the retina of the left eye specialized for high levels represents just the left side of space, and the retina of the right eye of light intensity, such as represents just the right side of space. (If you are still confused, those found during the day, and specialized for close one eye and keep it fixed—you should be able to see both the detection of different sides of space with a minor occlusion due to the nose.) Rather, the wavelengths. left side of the left eye and the left side of the right eye both contain Receptive field an image of objects on the right side of space. The right side of the The region of space that left eye and the right side of the right eye both contain an image of elicits a response from a objects on the left side of space. given neuron. The seeing brain 145 FIGURE 7.2: Receptive fields Light of two retinal ganglion cells. The cell in the upper part of Off On Off the figure responds when the center is illuminated “On” center (on-center, a) and when “Off” surround the surround is darkened a (off-surround, b). The cell in the lower part of the figure responds when the center is darkened (off-center, d) and when the surround is b illuminated (on-surround, e). Both cells give on- and off- responses when both center and surround are illuminated (c and f), but neither c response is as strong as when only center or surround is illuminated. From Hubel, 1963. “Off” center “On” surround d e f and surround of their receptive field as shown in Figure 7.2. Light falling in the center of the receptive field may excite the neuron, whereas light in the surrounding area may switch it off, but when the light is removed from this region the cell is excited again (on-center off-surround cells). Other retinal ganglion cells have the opposite profile (off-center on-surround cells). Light over the entire receptive field may elicit no net effect because the center and surround inhibit each other. These center-surround cells form the building blocks for more advanced processing by the brain, enabling detection of, among other things, edges and orientations. The output of the retinal ganglion cells is relayed to the brain via the optic nerves. The point at which the optic nerve leaves the eye is called the 146 THE STUDENT’S GUIDE TO COGNITIVE NEUROSCIENCE FIGURE 7.3: To find your blind spots, hold the image about 50 cm away. With your left eye open (right closed), look at the +. Slowly bring the image (or move your head) closer while looking at the + (do not move your eyes). At a certain distance, the dot will disappear from sight … this is when the dot falls on the blind spot of your retina. Reverse the process: Close your left eye and look at the dot with your right eye. Move the image slowly closer to you and the + should disappear. K EY TERMS blind spot, because there are no rods and cones present there. If you open only one of your eyes (and keep it stationary), there is a spot in which there is Blind spot no visual information. Yet, one does not perceive a black hole in one’s vision The point at which the (try the experiment in Figure 7.3). This is another example of the brain filling optic nerve leaves the eye. There are no rods in missing information. and cones present there. Primary visual cortex The primary visual cortex and geniculostriate pathway (or V1) Although there is a single channel of neural output from each eye, via the optic The first stage of visual nerve, this subsequently splits into multiple branches (in the optic tract and processing in the cortex; the region retains the beyond) with the net result being that there are a number of different pathways spatial relationships from the retina to the brain (for a review, see Stoerig & Cowey, 1997). The found on the retina and dominant visual pathway in the human brain travels to the primary visual cortex combines simple visual at the back, or posterior, of the brain, via a processing station called the lateral features into more com- geniculate nucleus (LGN) as shown in Figure 7.4. The LGN is part of the plex ones. thalamus which has a more general role in processing sensory information; there is one LGN in each hemisphere. The primary visual cortex is also referred to as V1, or as the striate cortex because it has a larger than usual stripe running through one layer that can be seen when stained and viewed under a microscope. This particular route is called the geniculostriate pathway. The neural representation in the lateral geniculate nucleus divides up information on the retinal surface in a number of interesting ways. Objects in the right side of space (termed the right visual field) fall on the left side of the retina of both eyes and project to the left lateral geniculate nucleus. The representation in the lateral geniculate nucleus thus contains information from both the left and right eyes. This information is segregated into the six different neuronal layers of this structure, three for each eye. The layers of the lateral geniculate nucleus are not only divided according to the eye (left or right) but also contain a further subdivision. The upper four layers have small cell bodies and have been termed parvocellular, or P layers, whereas the lower two layers contain larger cell bodies and have been termed FIGURE 7.4: Connections from the retina to the primary visual magnocellular, or M layers. Parvocellular cells cortex—the geniculostriate pathway. From Zeki, 1993. © Blackwell Publishing. Reproduced with permission. The seeing brain 147 respond to detail and are concerned with color vision. Magnocellular cells are more sensitive to movement than color and respond to larger areas of visual field (Maunsell, 1987). More recently a third type of cell (K or konio) has been documented in the LGN that lies between the magnocellular (magno) and parvocellular (parvo) layers (Hendry & Reid, 2000). These cells show much less functional specificity than magno and parvo cells and have a different pattern of connectivity. The neurons in the primary visual cortex (V1) transform the information in the lateral geniculate nucleus into a basic code that enables different kinds of visual information to be extracted by later stages of processing. First of all, neurons need to be able to represent how light or dark something is. In addition, neurons need to represent the color of an object to distinguish, say, between fruit and foliage of comparable lightness/darkness but complementary in color. Edges also need to be detected, and these might be defined as abrupt changes in brightness or color. These edges might be useful for perceiving the shape of objects. Changes in brightness or color could also reflect movement of an object, and it is conceivable that some neurons may be specialized for extracting this type of visual information. Depth may also be perceived by comparing the two different retinal images. The properties of neurons in the primary visual cortex were elucidated by pioneering work by David Hubel and Torsten Wiesel (1959, 1962, 1965, 1968, 1970a), for which they were awarded the Nobel Prize in Medicine in 1981. The method they used was to record the response of single neurons in the visual cortex of cats and monkeys. As with many great discoveries, there was an element of chance. Hubel and Wiesel noted that an oriented crack in a projector slide drove a single cell in V1 wild, i.e., it produced lots of action potentials (cited in Zeki, 1993). They then systematically went on to show that many of these cells responded only to particular orientations. These were termed simple cells. The responses of these simple cells could be construed KEY TERM as a combination of the responses of center-surround cells in the lateral Simple cells geniculate nucleus (Hubel & Wiesel, 1962), as shown in Figure 7.5. The cells In vision, cells that also integrate information across both eyes and respond to similar input to respond to light in a either the left or right eye. Many orientation-selective cells were found to be particular orientation (or wavelength-sensitive too (Hubel & Wiesel, 1968), thus providing a primitive points of light along that code from which to derive color. line). Just as center-surround cells might be the building blocks of simple cells, Hubel and Wiesel (1962) speculated that simple cells themselves EYE–BRAIN MYTH 2 If you think that the response of neurons on the retina or in the brain is like the response of pixels in a television screen, then think again. Some visual neurons respond when light is taken away, or when there is a change in light intensity across the region that they respond to. Other neurons in extrastriate areas respond only to certain colors, or move- ment in certain directions. These neurons often have very large recep- tive fields that do not represent a very precise pixel-like location at all. 148 THE STUDENT’S GUIDE TO COGNITIVE NEUROSCIENCE might be combined into what they termed complex cells. These are o rientation-selective too, but can be distinguished from simple cells by their larger receptive fields and the fact that complex cells require stimulation across their entire length, whereas simple cells will respond to single points of light within the excitatory region. Outside of V1, another type of cell, termed hypercomplex cells, which can be built from the responses of several complex cells, was observed (Hubel & Wiesel, 1965). These cells were also orientation-sensitive, but the length was also FIGURE 7.5: A simple cell in V1 responds to lines of particular critical. The receptive fields of hypercomplex length and orientation. Its response may be derived from a cells may consist of adding excitatory complex combination of responses from different cells with center-surround cells, but with inhibitory complex cells located properties such as those located in the lateral geniculate nucleus. at either end to act as “stoppers.” In sum, the From Zeki, 1993. © Blackwell Publishing. Reproduced with permission. response properties of cells in V1 enable more complex visual information (e.g., edges) to be constructed out of more simple information. K EY TERMS The take-home message of the work of Hubel and Wiesel is of a Complex cells hierarchically organized visual system in which more complex visual features In vision, cells that are built (bottom-up) from more simple ones. However, this is only half of respond to light in a the story. Information from more complex representations also propagates particular orientation but down the hierarchy. For instance, in the Kanizsa illusion, there are cells in do not respond to single V2 (but not V1) that respond to the illusory “white edges” of the triangle points of light. (Von der Heydt et al., 1984). This is assumed to reflect feedback information Hypercomplex cells to V2 from regions in the brain that represent shapes and surfaces In vision, cells that (Kogo & Wagemans, 2013). respond to particu- lar orientations and particular lengths. Cortical and non-cortical routes to seeing To date, around 10 different pathways from the eye to the brain have been discovered (Figure 7.6), of which the pathway via the lateral geniculate nucleus to V1 is the most well understood and appears to make the largest contribution to human visual perception (Stoerig & Cowey, 1997). The other routes are evolutionarily more ancient. Evolution appears not to have replaced these routes with “better” ones, but has retained them and added new routes that enable finer levels of processing or that serve somewhat different functions. For example, a visual route from retinal ganglion cells to the suprachiasmatic nucleus (SCN) in the hypothalamus provides information about night and day that is used to configure a biological clock (Klein et al., 1991). Other routes, such as via the superior colliculus and inferior pulvinar, are important for orienting to stimuli (e.g., a sudden flash of light) by initiating automatic body and eye movements (Wurtz et al., 1982). These latter routes are faster than the route via V1 and can thus provide an early warning signal; for instance, to threatening or unexpected stimuli. This can explain how it is possible to unconsciously turn to look at something without realizing its importance until after orienting. More recently, an alternative pathway from the LGN (via the K-cells) to the cortex has been documented that projects to a part The seeing brain 149 FIGURE 7.6: There are LGN Geniculo striate believed to be 10 different pathway Primary visual Magno routes from the retina to cortex (V1) different regions of the brain. Parvo Konio Extrastriate (e.g. V5/MT) Superior Colliculus Retina Inferior Pulvinar Suprachiasmatic nucleus (SCN) Pretectum Nucleus of optic tract Terminal nuclei of accessory optic tract (x3 = dorsal, medial and lateral) of the brain that is specialized for process of visual motion (area V5/MT) without first projecting to V1 (Sincich et al., 2004). This may account for the fact that some patients with cortical blindness can still discriminate motion. Evaluation The primary visual cortex (V1) contains cells that enable a basic detection of visual features, such as edges, that are likely to be important for segregating the scene into different objects. There is some evidence for a hierarchical processing of visual features such that responses of earlier neurons in the hierarchy form the building blocks for more advanced responses of neurons higher up in the hierarchy. A number of other routes operate in parallel to the geniculostriate route to V1. These may be important for early detection of visual stimuli, among other things. CO R T I C A L B L I N D N ES S AN D “ B LINDSIGHT” Loss of one eye, or the optic nerve of that eye, results in complete blindness in that eye. The spared eye would still be able to perceive the left and right sides of space and transmit information to the left and right primary visual cortex. But what would be the consequences of complete damage to one side of the primary visual cortex itself ? In this instance, there would be cortical blindness for one side of space (if the left cortex is damaged, then the right visual field would be blind, and vice versa). The deficit would be present when using either the left or right eye alone, or both eyes together. This deficit is 150 THE STUDENT’S GUIDE TO COGNITIVE NEUROSCIENCE K EY TERMS termed hemianopia (or homonymous hemianopia). Partial damage to the primary visual cortex might affect one subregion of space. As the upper part Hemianopia of V1 (above a line called the calcarine fissure) represents the bottom side of Cortical blindness space, and the lower part of V1 represents the top part of space, damage here restricted to one half of the visual field (associ- can give rise to cortical blindness in a quarter of the visual field (so-called ated with damage to the quadrantanopia). Blindness in a smaller region of space is referred to as a primary visual cortex in cortical scotoma. These are illustrated in Figure 7.7. Note that the layout of one hemisphere). visual information in V1 parallels that found on the retina. That is, points Quadrantanopia that are close in space on the retina are also close in space in V1. Areas such Cortical blindness as V1 are said to be retinotopically organized. restricted to a quarter of The previous section described how there are several visual routes from the visual field. the eye to the brain. Each of these routes makes a different contribution to Scotoma visual perception. Taking this on board, one might question whether damage A small region of cortical to the brain (as opposed to the eyes) could really lead to total blindness blindness. unless each and every one of these visual pathways coincidentally happened Retinotopic organization to be damaged. In fact, this is indeed the case. Damage to the primary The receptive fields of visual cortex does lead to an inability to report visual stimuli presented in a set of neurons are organized in such a way as to reflect the spatial organization present in the retina. (a) Hemianopia (b) Scotoma FIGURE 7.7: Partial damage to the primary visual cortex (V1) can result in blindness in specific regions (shown here in gray). This is because this region of the brain is retinotopically organized. Area V1 is at the back of the brain and on the middle surface between the two hemispheres. (c) Quadrantanopia Adapted from Zeki, 1993. The seeing brain 151 EYE–BRAIN MYTH 3 The image on the retina and the representation of it in V1 are “upside down” with respect to the outside world. As such, one might wonder how the brain turns it the right way up. This question is meaningless because it presupposes that the orientation of things in the outside world is in some way “correct” and the brain’s representation of it is in some way “incorrect.” There is no “correct” orientation (all orientation is relative) and the brain does not need to turn things around to per- ceive them appropriately. What does need to happen is that neurons coding the position of space in the visual field need to interface appropriately with, for instance, neurons that guide reaching movements of the hand upwards or downwards such that visual spatial information is appropriate- ly calibrated with other kinds of non-visual spatial information. the corresponding affected region of space and can be disabling for such a KEY TERM person. Nevertheless, the other remaining visual routes might permit some aspects of visual perception to be performed satisfactorily in exactly the same Blindsight A symptom in which the regions of space that are reported to be blind. This paradoxical situation has patient reports not being been referred to as “blindsight” (Weiskrantz et al., 1974). able to consciously see Patients exhibiting blindsight deny having seen a visual stimulus even stimuli in a particular though their behavior implies that the stimulus was in fact seen (for a review, region but can never- see Cowey, 2010). For example, patient DB had part of his primary visual theless perform visual cortex (V1) removed to cure a chronic and severe migraine (this was reported discriminations (e.g., long, short) accurately. in detail by Weiskrantz, 1986). When stimuli were presented in DB’s blind field, he reported seeing nothing. However, if asked to point or move his eyes to the stimulus, then he could do so with accuracy, while still maintaining that he saw nothing. DB could perform a number of other discriminations well above chance, such as orientation discrimination (horizontal, vertical or diagonal), motion detection (static or moving) and contrast discrimination (gray on black versus gray on white). In all these tasks DB felt as if he was guessing even though he clearly was not. Some form/shape discrimination was possible but appeared to be due to detection of edges and orientations rather than shape itself. For example, DB could discriminate between X and O, but not between X and A and not between squares and rectangles that contain lines of similar orientation (but see Marcel, 1998). How can the performance of patients such as DB be explained? First of all, one needs to eliminate the possibility that the task is being performed by remnants of the primary visual cortex. For example, there could be islands of spared cortex within the supposedly damaged region (Campion et al., 1983). However, many patients have undergone structural MRI and it has been established that no cortex remains in the region corresponding to the “blind” field (Cowey, 2010). Another explanation is that light from the stimulus is scattered into other intact parts of the visual field and is detected by intact parts of the primary visual cortex. For example, some patients may be able to detect stimuli supposedly in their blind field because of light reflected on their nose or other surfaces in the laboratory (Campion et al., 1983). Evidence against this comes from the fact that performance is superior in the “blindsight” region to the natural blind spot (found in us all). This cannot be 152 THE STUDENT’S GUIDE TO COGNITIVE NEUROSCIENCE FIGURE 7.8: If a visually presented semi-circle abuts a cortical scotoma (the shaded area), then the patient = “Semi-circle” = “Circle” might report a complete circle. Thus, rather than seeing a gap in their vision, patients with blindsight might fill in the gap using visual information in the spared field. If the semi-circle is presented inside the scotoma, it isn’t seen at all, whereas if it is away from the scotoma, it is perceived normally. Adapted from Torjussen, 1976. = “Nothing” Blind region accounted for by scattered light (see Cowey, 2010). Thus, the most satisfactory explanation of blindsight is that it reflects the operation of other visual routes from the eye to the brain rather than the residual ability of V1. For instance, the ability to detect visual motion in blindsight might be due to direct projections from the LGN to area V5/MT that bypasses V1 (Hesselmann et al., 2010). This account raises important questions about the functional importance of conscious versus unconscious visual processes. If unconscious visual processes can discriminate well, then why is the conscious route needed at all? As it turns out, such questions are misguided because the unconscious routes (used in blindsight) are not as efficient and are only capable of coarse discriminations in comparison to the finely tuned discriminations achieved by V1 (see Cowey, 2010). At present, we do not have a full understanding of why some neural processes but not others are associated with conscious visual experiences (e.g., Leopold, 2012). Nevertheless, studies of patients with blindsight provide important clues about the relative contribution and functions of the different visual pathways in the brain (Figure 7.8). Blindsight ≠ normal vision − awareness of vision K EY TERMS Blindsight = impaired vision + no awareness of vision Ventral stream In vision, a pathway ex- tending from the occipital F U N C T I O N AL SPE CIALIZATION OF THE VISUAL lobes to the temporal C O R T EX B EYO ND V1 lobes involved in object recognition, memory and The neurons in V1 are specialized for detecting edges and orientations, semantics. wavelengths and light intensity. These form the building blocks for Dorsal stream constructing more complex visual representations based on form (i.e., shape), In vision, a pathway color and movement. Some of the principal anatomical connections between extending from the these regions are shown in Figure 7.9. One important division, discussed occipital lobes to the in more detail in later chapters, is between the ventral stream (involved in parietal lobes involved object recognition and memory) and the dorsal stream (involved in action in visually guided action and attention. and attention). The ventral stream runs along the temporal lobes whereas the dorsal stream terminates in the parietal lobes. The seeing brain 153 FIGURE 7.9: Information Posterior Dorsal from V1 is sent in parallel parietal regions Stream to a number of other V3A regions in the extrastriate cortex, some of which are specialized for processing V1 V2 V3 V5/MT Superior temporal particular visual attributes FST sulcus (STS) (e.g., V5/MT for movement). These extrastriate regions interface with the temporal V4 cortex (involved in object TEO recognition) and the parietal cortex (involved in space and attention). Inferotemporal Ventral region (IT) Stream Striate Prestriate Non-visual (visual) (visual) cortical areas The occipital cortex outside V1 is known as the extrastriate cortex KEY TERMS (or prestriate cortex). The receptive fields in these extrastriate visual areas become increasingly broader and less coherently organized in space, with V4 A region of the extras- areas V4 and V5/MT having very broad receptive fields (Zeki, 1969). The triate cortex associated extrastriate cortex also contains a number of areas that are specialized for with color perception. processing specific visual attributes such as color (area V4) and movement (area V5 or MT, standing for medial temporal). To some extent, the brain’s V5 (or MT) A region of the extras- strategy for processing information outside of V1 is to “divide and conquer.” triate cortex associated For example, it is possible to have brain damage that impairs color perception with motion perception. (cerebral achromatopsia) or movement perception (cerebral akinetopsia) Achromatopsia that preserves other visual functions. A failure to perceive color (the world appears V4: the main color center of the brain in grayscale), not to be confused with color blind- Area V4 is believed to be the main color center in the human brain because ness (deficient or absent lesions to it result in a lack of color vision, so that the world is perceived in types of cone cell). shades of gray (Heywood et al., 1998; Zeki, 1990). This is termed cerebral Akinetopsia achromatopsia. It is not to be confused with color blindness in which people A failure to perceive (normally men) have difficulty discriminating, for instance, reds and greens visual motion. because of a deficiency in certain types of retinal cells. Achromatopsia is rare because there are two V4 areas in the brain and it is unlikely that brain damage would symmetrically affect both hemispheres. Damage to one of the V4s would result in one side of space being seen as colorless (left V4 represents color for the right hemifield and vice versa). Partial damage to V4 can result in colors that appear “dirty” or “washed out” (Meadows, 1974). In people who have not sustained brain injury, area V4 can be identified by functional imaging by comparing viewing patterns of colored squares (so-called Mondrians, because of a similarity to the work of that artist) with their equivalent 154 THE STUDENT’S GUIDE TO COGNITIVE NEUROSCIENCE grayscale picture (Zeki et al., 1991). The grayscale pictures are matched for K EY TERM luminance such that if either image were viewed through a black-and-white Color constancy camera, they would appear identical to each other. The color of a surface is Why is color so important that the brain would set aside an entire region perceived as constant dedicated to it? Moreover, given that the retina contains cells that detect even when illuminated in different lighting con- different wavelengths of visible light, why does the brain need a dedicated ditions. color processor at all? To answer both of these questions, it is important to understand the concept of color constancy. Color constancy refers to the fact that the color of a surface is perceived as constant even when illuminated in different lighting conditions and even though the physical wavelength composition of light reflected from a surface can be shown (with recording devices) to differ under different conditions. For example, a surface that reflects a high proportion of long-wave “red” light will appear red when illuminated with white, red, green or any other type of light. Color constancy ONLINE RESOURCES is needed to facilitate recognition of, say, red tomatoes across a wide variety What colors do of viewing conditions. The online viral phenomenon known as “The Dress” is you see? Visit the also an example of color constancy (see Figure 7.10). If the viewer interprets companion website the dress as being illuminated by yellowish light then the fabric color is (www.routledge.com/ cw/ward) for the perceived as black and blue, but if it is interpreted as being illuminated by original photo of the blue light then the color is perceived as white and gold (Lafer-Sousa et al., dress. 2015). The close cropping of the image helps to create the initial ambiguity in the background lighting condition. The derivation of color constancy appears to be the function of V4 (Zeki, 1983) (Figure 7.11). Neurons in V4 may achieve this by comparing the wavelength in their receptive fields with the wavelength in other fields. In this way, it is possible to compute the color of a surface while taking into account the illuminating conditions of the whole scene (Land, 1964, 1983). Cells in earlier visual regions (e.g., V1) respond only to the local wavelength in their receptive field and their response would change if the light source were changed even if the color of the stimulus was not (Zeki, 1983), a finding that has been replicated in humans using fMRI (Brouwer & Heeger, 2009). Achromatopsic patients with damage to V4 are able to use earlier visual processes FIGURE 7.10: What color is “The Dress”? The original image that are based on wavelength discrimination in went viral on social media because some people perceive it as the absence of color experience. For example, black and blue, and others as white-gold. This image shows how the same dress can be perceived differently depending on patient MS could tell if two equiluminant whether the ambient lighting is assumed to be bright or dark. colored patches were the same or different if https://commons.wikimedia.org/wiki/File:Wikipe-tan_wearing_The_ they abutted to form a common edge, but not Dress_reduced.svg. Licensed under CC BY-SA 3.0 if they were separated (Heywood et al., 1991). This occurs because wavelength comparisons outside of V4 are made at a local level. Although earlier visual regions respond to wavelength, V4 has some special characteristics. The neurons in V4 tend to have larger receptive fields than earlier regions. Moreover, The seeing brain 155 evidence from fMRI shows that voxels that are sensitive to one color (e.g., red) tend to have graded selectivity to perceptually neighboring colors (e.g., violets, yellows), but this is not found in earlier visual regions (Brouwer & Heeger, 2009). It suggests that V4 implements a relational coding between colors (analogous to a color wheel) that may also be helpful for color constancy. Although V4 has been described as “the main color center in the human brain,” it does not follow that it only processes color information. Evidence from macaque single- cell recordings suggests neurons in V4 also respond to visual features such as shape and texture (Roe et al., 2012). Moreover, it should be pointed out that V4 is not the only color- responsive region of the brain. For example, Zeki and Marini (1998) compared viewing of appropriately colored objects (e.g., a red tomato) with inappropriate ones (e.g., a blue tomato) in FIGURE 7.11: Area V5/MT (in red) lies near the outer surface of both hemispheres and is responsible for perception of visual humans and found activation in, among other motion. Area V4 (in blue) lies on the under surface of the brain, regions, the hippocampus, which may code in each hemisphere, and is responsible for the perception of long-term memory representations. Memory color. This brain is viewed from the back. representations of color can also activate the visual cortex: viewing a grayscale banana can induce a pattern of activity consistent with yellow in regions such as V1 and V4 (Bannert & Bartels, 2013). V5/MT: the main movement center of the brain If participants in a PET scanner view images of moving dots relative to static dots, a region of the extrastriate cortex called V5 (or MT) becomes particularly active (Zeki et al., 1991). Earlier electrophysiological research on the monkey had found that all cells in this area are sensitive to motion, and that 90 percent of them respond preferentially to a particular direction of motion and will not respond at all to the opposite direction of motion (Zeki, 1974). None were color-sensitive. Patient LM lost the ability to perceive visual movement after bilateral damage to area V5/MT (Zihl et al., 1983). This condition is termed akinetopsia (for a review, see Zeki, 1991). Her visual world consists of a series of still frames: objects may suddenly appear or disappear, a car that is distant may suddenly be seen to be near, and pouring tea into a cup would invariably end in spillage as the level of liquid appears to rise in jumps rather than smoothly. Other studies have suggested that other types of movement perception do not rely on V5/MT. For example, LM is able to discriminate biological from non-biological motion (McLeod et al., 1996). The perception of 156 THE STUDENT’S GUIDE TO COGNITIVE NEUROSCIENCE K EY TERM biological motion is assessed by attaching light points to the joints and then recording someone walking/running in the dark (Figure 7.12). When only the Biological motion light points are viewed, most people are still able to detect bodily movement The ability to detect whether a stimulus is (relative to a condition in which these moving lights are presented jumbled animate or not from up). LM could discriminate biological from non-biological motion, but could movement cues alone. not perceive the overall direction of movement. Separate pathways for this type of motion have been implied by functional imaging (Vaina et al., 2001). LM was able to detect movement in other sensory modalities (e.g., touch, audition), suggesting that her difficulties were restricted to certain types of visual movement (Zihl et al., 1983). However, functional imaging studies have identified supramodal regions of the brain (in the parietal cortex) that appear to respond to movement in three different senses—vision, touch and hearing (Bremmer et al., 2001). Evaluation One emerging view of visual processing in the brain beyond V1 is that different types of visual information get parsed into more specialized brain regions. Thus, when one looks at a dog running across the garden, information about its color resides in one region, information about its movement resides in another and information about its identity (this is my dog rather than any dog) resides in yet another, to name but a few. The question of how these different streams of information come back together (if at all) is not well understood, but may require the involvement of non-visual processes related to attention (see Chapter 9). R EC O G N I Z I N G OBJE CTS For visual information to be useful it must make contact with knowledge that has been accumulated about the world. There is a need to recognize places that have been visited and people who have been seen, and to recognize other stimuli in the environment in order to, say, distinguish between edible and non-edible substances. All of these examples can be subsumed under the process of “object recognition.” Although different types of object (e.g., faces) may recruit some different mechanisms, there will nevertheless be some FIGURE 7.12: When this array of dots is set in motion, most people can distinguish between biological and non-biological motion. The seeing brain 157 HOW DOES THE BRAIN RESPOND TO VISUAL ILLUSIONS? When you look at the top part of Figure 7.13 do you have a sense of m otion in the circles even though the image is static? This image is called the Enigma il- lusion. When you look at the bottom image do you see one vase or two faces? Does this image appear to spontaneously flip between one interpretation and the other, even though the image remains constant? Examples such as these reveal how the brain’s perception of the world can differ from the external physical reality. This is, in fact, a normal part of seeing. Visual illu- sions are in many respects the norm rather than the exception, even though we are not always aware of them as such. A functional imaging study has shown that parts of the brain specialized for de- tecting real movement (area V5/MT) also respond to the Enigma illusion (Zeki et al., 1993). It has been suggested that the illusion of movement is driven by tiny ad- justments in eye fixation (Troncoso et al., FIGURE 7.13: Do you see movement in the image on the top 2008). An fMRI study using bi-stable when you stare at the center? Do you see a vase or faces on the bottom? How does the brain interpret such ambiguities? stimuli such as the face–vase has shown Godong / Alamy Stock Photo how different visual and non-visual brain structures cooperate to maintain perceptual stability. The momentary breakdown of activity in these regions is associated with the timing of the subjective perceptual flip (Kleinschmidt et al., 1998). TMS over the right parietal lobes affects the rate of switch between bi-stable images with adjacent regions either promoting stability or generating instability (Kanai et al., 2011). This suggests differ- ent top-down biasing influences on perception. common mechanisms shared by all objects, given that they are extracted from the same raw visual information. Figure 7.14 outlines several basic stages in object recognition that, terminology aside, bear a close resemblance to Marr’s (1976) theory of vision: ONLINE RESOURCES 1. The earliest stage in visual processing involves basic elements such as To learn more about edges and bars of various lengths, contrasts and orientations. This stage visual illusions and how they are created has already been considered above. by the brain, check out 2. Later stages involve grouping these elements into higher-order units that illusionoftheyear.com code depth cues and segregate surfaces into figure and ground. Some and visit the companion of these mechanisms were first described by the Gestalt psychologists website (www.routledge. com/cw/ward). 158 THE STUDENT’S GUIDE TO COGNITIVE NEUROSCIENCE and are considered below. It is possible that this stage is also influenced by top- down information based on stored knowledge. These visual representations, however, represent objects according to the observer’s viewpoint and object constancy is not present. 3. The viewer-centered description is then matched onto stored three-dimensional descriptions of the structure of objects (structural descriptions). This store is often assumed to represent only certain viewpoints and thus the matching process entails the computation of object constancy (i.e., an understanding that objects remain the same irrespective of differences in viewing condition). There may be two different routes to achieving object constancy, depending on whether the view is “normalized” by rotating the object to a standard orientation. 4. Finally, meaning is attributed to the stimulus and other information (e.g., the name) becomes available. This will be considered primarily in Chapter 12. Disorders of object recognition are referred to as visual agnosia, and these have been traditionally subdivided into apperceptive agnosia and associative agnosia, depending FIGURE 7.14: A simple model of visual object recognition. on whether the deficit occurs at stages involved From Riddoch and Humphreys, 2001. in perceptual processing or stages involving stored visual memory representations (Lissauer, 1890). This classification is perhaps too simple to be of much use in modern cognitive neuroscience. K EY TERMS Models such as the one of Riddoch and Humphreys (2001) acknowledge that Structural descriptions both perception and the stored properties of objects can be broken down A memory representation into even finer processes. It is also the case that most contemporary models of the three-dimensional of object recognition allow for interactivity between different processes structure of objects. rather than discrete processing stages. This is broadly consistent with the Apperceptive agnosia neuroanatomical data (see earlier) of connections between early and late A failure to understand visual regions and vice versa. the meaning of objects due to a deficit at the lev- el of object perception. Parts and wholes: gestalt grouping principles Associative agnosia In the 1930s, Gestalt psychologists identified a number of principles that A failure to understand explain why certain visual features become grouped together to form the meaning of objects perceptual wholes (Figure 7.15). These operations form a key stage in due to a deficit at the lev- el of semantic memory. translating simple features into three-dimensional descriptions of the world, essential for object recognition. The process of segmenting a visual The seeing brain 159 FIGURE 7.15: The Gestalt principles of (a) law of proximity, (b) law of similarity, (c) law of good continuation and (d) law of closure. display into objects versus background surfaces is also known as figure– KEY TERM ground segregation. The Gestalt approach identified five basic principles to account for how basic visual features are combined: Figure–ground segregation The process of segment- 1. The law of proximity states that visual elements are more likely to be ing a visual display into grouped if they are closer together. For example, the dots in (a) in the objects versus back- figure tend to be perceived as three horizontal lines because they are ground surfaces. closer together horizontally than vertically. 2. The law of similarity states that elements will be grouped together if they share visual attributes (e.g., color, shape). For example, (b) tends to be perceived as vertical columns rather than rows, because elements in columns share both shape and color. 3. The law of good continuation states that edges are grouped together to avoid changes or interruptions; thus, (c) is two crossing lines rather than > and