HSF2 Test 3 PDF
Document Details
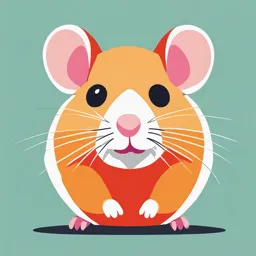
Uploaded by FrugalSet
OCR
Tags
Summary
This document is a test on the immune system, covering innate defenses and the inflammatory response. It details mechanical barriers, protective chemicals, phagocytosis, and inflammation mediators.
Full Transcript
HSF2 Test 3 Immune System I. Innate Defenses A. Mechanical Barriers to protect against pathogens: 1.Skin and Mucous Membranes: Skin: The outermost layer of the body provides a physical barrier against pathogens, preventing their entry into the body. Mucous Membranes: These line various body cavities...
HSF2 Test 3 Immune System I. Innate Defenses A. Mechanical Barriers to protect against pathogens: 1.Skin and Mucous Membranes: Skin: The outermost layer of the body provides a physical barrier against pathogens, preventing their entry into the body. Mucous Membranes: These line various body cavities and organs, such as the respiratory, gastrointestinal, and reproductive tracts, producing mucus that traps pathogens and prevents them from entering tissues. 2.Protective chemicals: a. Acid: Stomach acid and other acidic environments in the body help to kill or neutralize pathogens before they can cause infection. b. Enzymes: Lysozyme: Found in tears, saliva, and mucus, lysozyme breaks down the cell walls of certain bacteria, weakening or destroying them. c. Mucin: Mucin proteins form the basis of mucus, which helps to trap pathogens and prevent them from reaching tissues. d. Defensins: These are small, antimicrobial peptides found in various bodily secretions, including saliva, sweat, and mucus. Defensins can directly kill pathogens by disrupting their cell membranes. e. Other chemicals: Sebum: A oily substance produced by the skin’s sebaceous glands, sebum forms a protective barrier on the skin’s surface and has antimicrobial properties. Dermicidin: An antimicrobial peptide secreted by sweat glands, dermicidin helps to inhibit the growth of bacteria on the skin’s surface. B. Internal Defenses 1.Phagocytosis: a. Phagolysosome: After a pathogen is engulfed by a phagocyte, it forms a phagosome. This phagosome then fuses with lysosomes containing digestive enzymes, forming a phagolysosome. Within the phagolysosome, the pathogen is broken down and destroyed through enzymatic degradation. b. Neutrophils and Macrophages: Neutrophils are the most abundant type of white blood cell and are usually the rst responders to infection. They are highly ef cient at engul ng and destroying pathogens through phagocytosis. Macrophages, derived from monocytes, are present in tissues throughout the body and also play a critical role in phagocytosis. Macrophages are especially important for engul ng and digesting pathogens that have evaded neutrophil attack. c. Respiratory Burst: During phagocytosis, phagocytes can undergo a respiratory burst, a rapid release of reactive oxygen species (ROS) such as superoxide and hydrogen peroxide. These ROS are toxic to pathogens and help to kill them by damaging their cell membranes and DNA. d. Opsonins: Opsonins are molecules that enhance the process of phagocytosis by marking pathogens for engulfment. Opsonins bind to the surface of pathogens, tagging them for recognition by phagocytes. This tagging increases the ef ciency of phagocytosis by promoting the fi fi attachment of phagocytes to the pathogens. e. Natural Killer Cells: Natural killer (NK) cells are a type of lymphocyte that plays a crucial role in the innate immune response. Unlike other lymphocytes, NK cells do not require prior sensitization to recognize and eliminate target cells. Instead, they can directly recognize and kill virus-infected or abnormal cells, such as cancer cells. NK cells release cytotoxic granules containing perforin and granzymes, which induce apoptosis (cell death) in target cells. 2. In ammation: a. Mast Cells: Mast cells are a type of immune cell found in connective tissue and mucous membranes. They play a central role in the initiation of the in ammatory response. When activated by pathogens or tissue injury, mast cells release in ammatory mediators, including histamine, which promote vasodilation and increase vascular permeability. b. Histamine: Histamine is a key in ammatory mediator released by mast cells during the early stages of in ammation. It causes vasodilation (expansion of blood vessels) and increases vascular permeability, leading to the characteristic symptoms of in ammation such as redness, heat, and swelling. Histamine also stimulates the migration of immune cells to the site of in ammation. c. Kinins: Kinins are a group of peptides that are generated during the in ammatory response. They promote in ammation by inducing vasodilation, increasing vascular permeability, and stimulating the release of other in ammatory mediators. Kinins also contribute to the sensation of pain associated with in ammation by sensitizing pain receptors. d. Prostaglandins: Prostaglandins are lipid compounds derived from fatty acids that play diverse roles in in ammation. They are synthesized in response to in ammatory stimuli and contribute to the in ammatory response by promoting vasodilation, increasing vascular permeability, and enhancing the sensitization of pain receptors. Prostaglandins also play a role in fever induction during infection. e. Hyperemia: Hyperemia refers to an increase in blood ow to the affected area during in ammation. It occurs as a result of vasodilation, which is mediated by in ammatory mediators such as histamine and prostaglandins. The increased blood ow leads to the characteristic redness and warmth associated with in ammation. Hyperemia serves several purposes during in ammation, including delivering immune cells and mediators to the site of injury or infection, removing debris and toxins, and facilitating tissue repair. f. Phagocyte Mobilization: Phagocytes, including neutrophils and macrophages, play a crucial role in the in ammatory response by engul ng and destroying pathogens, as well as clearing cellular debris. During in ammation, various signals attract phagocytes to the site of injury or infection, a process known as phagocyte mobilization. These signals include chemotactic factors released by damaged tissues, complement proteins, and microbial products. Chemotactic factors act as chemical attractants, guiding phagocytes to the source of in ammation. Once recruited, phagocytes migrate across blood vessel walls and into the surrounding tissue, where they engulf and digest pathogens and debris. Phagocyte mobilization is essential for the effective clearance of pathogens and the resolution of in ammation. 1. Leukocytosis: Increase in circulating leukocytes, particularly neutrophils, due to in ammatory signals. 2.Margination: Adherence of leukocytes to blood vessel walls in response to endothelial activation. 3. Diapedesis: Migration of leukocytes from bloodstream to tissue through endothelial gaps. 4Chemotaxis: Directed movement of leukocytes towards in ammatory signals released by damaged tissues or pathogens. fl fl fl fl fl fl fl fl fl fl fl fl fl fl 3. Antimicrobial Proteins: a. Interferons: Interferons are a group of proteins produced by cells in response to viral infections, certain bacteria, and other pathogens. They play a critical role in the innate immune response by inhibiting viral replication within infected cells and alerting neighboring cells to the presence of the pathogen. Interferons also enhance the activity of other immune cells, such as natural killer cells and macrophages, helping to coordinate the overall immune response to infection. b. Complement: The complement system is a group of proteins that play multiple roles in the immune response, including pathogen recognition, opsonization, in ammation, and direct killing of pathogens. The complement system can be activated through three different pathways: Classical pathway: 1.The classical pathway is initiated by the binding of complement protein C1 to antibodies that have bound to pathogens. This activates a cascade of complement proteins, ultimately leading to the formation of the membrane attack complex (MAC), which creates pores in the membranes of target cells, causing lysis. Lectin pathway: 2. The lectin pathway is activated when mannose-binding lectin (MBL), a pattern recognition molecule, binds to speci c carbohydrates on the surface of pathogens. MBL then activates a cascade of complement proteins, similar to the classical pathway, leading to the formation of the MAC and pathogen lysis. Alternative pathway: 3. The alternative pathway is continuously active at a low level and can be triggered directly by certain microbial surfaces. This pathway involves the spontaneous activation of complement protein C3, leading to the assembly of the MAC and pathogen lysis. 4. Fever: a. More widespread than in ammation: Fever is a systemic response involving an increase in body temperature, usually in response to infection or in ammation. Unlike in ammation, which primarily affects speci c tissues or organs, fever affects the entire body. It is mediated by the release of pyrogens, which act on the hypothalamus in the brain to raise the body’s set-point temperature. b. Pyrogens: Pyrogens are substances that induce fever by acting on the hypothalamus, the body’s temperature-regulating center in the brain. Pyrogens can be endogenous, produced by the body itself in response to infection or in ammation, or exogenous, originating from external sources such as pathogens or toxins. Endogenous pyrogens include cytokines such as interleukin-1 (IL-1), interleukin-6 (IL-6), and tumor necrosis factor-alpha (TNF-alpha), which are released by immune cells in response to infection or tissue injury. Exogenous pyrogens include fi fl fl fl bacterial toxins and other microbial products that directly stimulate the release of endogenous pyrogens or mimic their effects. II. Adaptive Defenses: A. Humoral Immunity: Utilizes antibodies: Humoral immunity is mediated by antibodies, also known as immunoglobulins, which are proteins produced by B lymphocytes (B cells). These antibodies circulate in the bloodstream and lymphatic system, where they bind to speci c antigens, such as proteins on the surface of pathogens, marking them for destruction or neutralization by other components of the immune system. Extracellular: Humoral immunity primarily targets pathogens and toxins that are present outside of host cells, such as bacteria and viruses circulating in the bloodstream or body uids. Antibodies can neutralize pathogens by blocking their ability to infect host cells, facilitate their phagocytosis by immune cells, or activate the complement system to promote their destruction. B. Cellular Immunity: Lymphocytes: Cellular immunity is mediated by lymphocytes, a type of white blood cell that includes T lymphocytes (T cells) and natural killer (NK) cells. T cells play a central role in cellular immunity by recognizing and destroying infected or abnormal cells, such as virus-infected cells or cancer cells. NK cells also contribute to cellular immunity by recognizing and eliminating target cells without prior sensitization. Cellular Targets: Cellular immunity targets pathogens that have invaded host cells, as well as abnormal or damaged host cells. T cells recognize antigenic peptides presented on the surface of infected or abnormal cells by major histocompatibility complex (MHC) molecules. This recognition triggers the activation of T cells, leading to the release of cytotoxic molecules that induce apoptosis (cell death) in the target cells 4 key characteristics of the adaptive immune response Involves B and T lymphocytes It is speci c It is systemic It has “memory” C. Antigens: Antigen Generating: 1. Complete Antigens: a. Immunogenicity: Immunogenicity refers to the ability of a substance to stimulate the immune system, particularly speci c lymphocytes, to proliferate and generate an immune response. Complete antigens are highly immunogenic, meaning they can induce a robust immune response by activating lymphocytes, such as B cells and T cells. b. Reactivity: Reactivity describes the ability of an antigen to bind speci cally to the activated lymphocytes and the antibodies produced by fi fl fi the immune response. Complete antigens exhibit both immunogenicity and reactivity, allowing them to interact with lymphocytes and antibodies to induce and sustain an immune response. 2. Incomplete Antigens: Incomplete antigens, also known as haptens, are molecules that are too small to stimulate an immune response on their own. However, when coupled with carrier proteins or larger molecules, haptens can become immunogenic and elicit an immune response. Examples of haptens include certain drugs, chemicals, and environmental allergens. Self-Antigens – MHC Proteins (Major Histocompatibility Complex): Self-antigens are molecules derived from the body’s own cells and tissues. These molecules, including proteins and other cellular components, are recognized by the immune system as “self” and typically do not induce an immune response under normal conditions. However, in autoimmune diseases, self-antigens may be mistakenly recognized as foreign by the immune system, leading to immunemediated damage to healthy tissues. Major histocompatibility complex (MHC) proteins are a group of cell surface proteins that play a crucial role in the immune response by presenting antigenic peptides to T cells. MHC proteins are encoded by a highly polymorphic gene complex, allowing them to present a wide range of antigenic peptides to T cells. MHC molecules are divided into two classes: MHC class I molecules, which present peptides derived from intracellular pathogens to CD8+ T cells, and MHC class II molecules, which present peptides derived from extracellular pathogens to CD4+ T cells. D. B and T Lymphocytes: There are 3 cell types involved in the adaptive immune system: B lymphocytes, T lymphocytes, and APCs (Antigen-Presenting Cells). 1.Lymphocytes: a. Formed in the Bone Marrow: Lymphocytes, including both B cells and T cells, originate from hematopoietic stem cells in the bone marrow. These stem cells give rise to precursor cells that differentiate into mature lymphocytes. b. Maturation Occurs in the Bone Marrow (B Cells) and the Thymus (T Cells): B lymphocytes (B cells) undergo maturation in the bone marrow, where they acquire immunoglobulin receptors on their surface and become immunocompetent. T lymphocytes (T cells), on the other hand, undergo maturation in the thymus gland. During maturation, T cells undergo a process of positive and negative selection to ensure immunocompetence and self-tolerance. 1.) Immunocompetence: Immunocompetence refers to the ability of lymphocytes to recognize and respond to speci c antigens. B cells and T cells acquire immunocompetence through the rearrangement of their antigen receptor genes, resulting in a diverse repertoire of receptors capable of recognizing a wide range of antigens. Immunocompetent lymphocytes that encounter their speci c antigen become activated and initiate an immune response. 2.) Self-Tolerance: Self-tolerance is the ability of the immune system to distinguish between self-antigens (molecules derived from the body’s own cells and tissues) and foreign antigens. During lymphocyte maturation, cells that recognize self-antigens with high af nity are eliminated or rendered tolerant to prevent autoimmune reactions. This process ensures that the immune system can effectively target foreign pathogens while avoiding harmful immune responses against healthy tissues. c. Seeding and Circulation: Naïve: Naïve lymphocytes are immature lymphocytes that have completed maturation in the bone marrow (B cells) or thymus (T cells) but have not yet encountered their speci c antigen. Naïve lymphocytes circulate throughout the bloodstream and lymphatic system, continuously surveying peripheral tissues for the presence of antigens. Exportation: After maturation, naïve lymphocytes exit the primary lymphoid organs (bone marrow for B cells, thymus for T cells) and enter the bloodstream and lymphatic system. From there, they are systematically distributed to secondary lymphoid organs, such as lymph nodes, spleen, and mucosa-associated lymphoid tissues (MALT), where they await encounter with their speci c antigen. d. Antigen Encounter and Activation: Clonal Selection: Upon encountering their speci c antigen presented by antigen-presenting cells (APCs) in secondary lymphoid organs, naïve lymphocytes undergo activation through a process known as clonal selection. This process involves the binding of the lymphocyte’s antigen receptor to the antigen, followed by activation signals delivered by co-stimulatory molecules. Activated lymphocytes then undergo clonal expansion to generate a population of effector cells speci c for the encountered antigen. e. Proliferation and Differentiation: Clone: Clonal proliferation refers to the rapid expansion of activated lymphocytes into a large population of identical cells, known as a clone. This expansion is driven by cytokines and growth factors released during the activation process, resulting in the ampli cation of the immune response against the antigen. Effector Cells: Effector cells are the descendants of activated lymphocytes that have differentiated into specialized cell types with speci c functions in the immune response. B cells differentiate into plasma cells, which produce and secrete antibodies targeting the antigen. T cells differentiate into various effector subsets, such as cytotoxic T cells, helper T cells, and regulatory T cells, each with distinct functions in cellmediated immunity. Memory Cells: Memory cells are long-lived lymphocytes that persist after the clearance of the antigen and provide immunological memory. Memory B cells and memory T cells are capable of rapidly responding to subsequent encounters with the same antigen, mounting a faster and more robust immune response upon re-exposure. Memory cells are critical for the establishment of long-term immunity against fi fi fi pathogens and the effectiveness of vaccines. 2. Selection: a. Positive Selection: Positive selection is a process that occurs during the maturation of T lymphocytes (T cells) in the thymus. It involves the selection of T cells with functional antigen receptors capable of recognizing peptide antigens presented by major histocompatibility complex (MHC) molecules on thymic epithelial cells. During positive selection, immature T cells undergo screening to ensure that they can bind to self-MHC molecules with moderate af nity. T cells that fail to interact with self-MHC molecules undergo apoptosis, while those that successfully bind to self-MHC molecules survive and progress to the next stage of maturation. b. Negative Selection: Negative selection, also known as central tolerance, is a process that occurs during the maturation of both B lymphocytes (B cells) in the bone marrow and T cells in the thymus. It involves the elimination or inactivation of lymphocytes that recognize self-antigens with high af nity. During negative selection, immature lymphocytes are exposed to a diverse array of self-antigens presented by antigen-presenting cells (APCs) or thymic epithelial cells. Lymphocytes that react strongly with self-antigens undergo apoptosis or are rendered functionally tolerant to prevent autoimmune reactions. Negative selection helps to ensure that mature lymphocytes are capable of mounting effective immune responses against foreign pathogens while maintaining tolerance to self. 3. Antigen Presenting Cells (APCs): a. Dendritic Cells: Dendritic cells (DCs) are specialized immune cells that play a central role in antigen presentation and activation of the adaptive immune response. They are found in peripheral tissues, where they act as sentinels, continuously sampling their environment for foreign antigens. Upon encountering pathogens or tissue damage, dendritic cells undergo a process of maturation, during which they upregulate the expression of co-stimulatory molecules and migrate to secondary lymphoid organs, such as lymph nodes. There, mature dendritic cells present processed antigens to T cells, initiating T cell activation and differentiation into effector cells. Dendritic cells are highly ef cient at capturing, processing, and presenting a wide range of antigens to both CD4+ and CD8+ T cells, making them essential for the initiation and regulation of adaptive immune responses. b. Macrophages: Macrophages are phagocytic cells that play multiple roles in the immune system, including antigen presentation and phagocytosis of pathogens and cellular debris. Macrophages are present in various tissues throughout the body, where they serve as rstline defenders against infection and tissue damage. They express a wide range of pattern recognition receptors (PRRs), allowing them to recognize and engulf pathogens through phagocytosis. Once activated, macrophages process and present antigenic peptides derived from engulfed pathogens to T cells, initiating adaptive immune responses. Macrophages also produce cytokines and chemokines that regulate in ammation and coordinate the immune response. In addition to their role in antigen presentation, macrophages participate in tissue repair and remodeling following infection or injury. c. B Lymphocytes (B Cells): While B lymphocytes (B cells) are primarily known for their role in antibody production, they also function as antigen-presenting cells in certain contexts. B cells can internalize antigens through receptor-mediated endocytosis or by capturing antigens bound to their surface immunoglobulin receptors. Once internalized, B cells process antigens and present antigenic peptides to helper T fi fi fl fi cells, leading to T cell activation and collaboration between B and T cells in the germinal centers of lymphoid tissues. This interaction promotes B cell differentiation into antibody-secreting plasma cells and the production of high-af nity antibodies speci c for the encountered antigen. B cells also express co-stimulatory molecules and can provide additional signals for T cell activation and differentiation. Overall, B cells play a crucial role in the adaptive immune response by presenting antigens to T cells and producing antibodies to neutralize pathogens. III. Humoral Immunity: A. Humoral immunity is mediated by antibody molecules that are secreted by B lymphocytes: 1. Cross-linking of Antigen and Receptors: Humoral immunity begins with the recognition and binding of antigens by B cell receptors (BCRs), which are membrane-bound immunoglobulin molecules on the surface of B cells. When antigens bind to BCRs, they cross-link multiple BCRs together, leading to the activation of the B cell and initiation of intracellular signaling pathways. 2. Receptor-Mediated Endocytosis – Clonal Selection: Following antigen binding, activated B cells undergo receptor-mediated endocytosis, internalizing the bound antigens for processing and presentation to helper T cells. This process, known as clonal selection, leads to the activation and differentiation of B cells into antibody-secreting plasma cells or memory B cells. 3. Plasma Cells: Plasma cells are the effector cells of humoral immunity, derived from activated B cells through clonal selection. Plasma cells are specialized for the production and secretion of antibodies, also known as immunoglobulins (Ig). Each plasma cell secretes large quantities of a single type of antibody speci c for the recognized antigen. Plasma cells play a central role in the elimination of pathogens and the neutralization of toxins by producing antibodies that bind to and target these antigens for destruction or clearance by other components of the immune system. 4. Antibody Secretion: Antibodies are soluble proteins secreted by plasma cells that circulate in the bloodstream and lymphatic system, where they bind to speci c antigens and mediate various immune functions. Antibodies can neutralize pathogens by blocking their ability to infect host cells, enhance phagocytosis by opsonizing pathogens for engulfment by phagocytes, and activate the complement system to promote pathogen lysis and clearance. 5.Memory Cells: Memory B cells are long-lived lymphocytes that persist after the resolution of an immune response and provide immunological memory against speci c antigens. Memory B cells are generated during clonal selection and can rapidly respond to reexposure to the same antigen by differentiating into antibody-secreting plasma cells. Memory B cells play a crucial role in the secondary immune response, mounting a faster and more robust response upon re-infection or re-exposure to the antigen, leading to enhanced protection against pathogens. B. Immunological Memory: 1.Primary Immune Response: a. Occurs on First Response to the Antigen: The primary immune response is the initial encounter of the immune system with a speci c antigen. During the primary response, naïve B cells recognize the antigen, undergo clonal selection and expansion, and differentiate into plasma cells that produce and release antibodies speci c to the antigen. b. Lag Period – Allows for Proliferation of B Cells (3-6 days): The primary immune response is characterized by a lag period, during which fi fi fi fi there is a delay between antigen exposure and the production of detectable levels of antibodies. This lag period typically lasts 3-6 days and allows for the proliferation and differentiation of B cells into antibody-secreting plasma cells. c. Antibody Levels Rise (About 10 Days): Following the lag period, antibody levels in the bloodstream begin to rise as plasma cells produce and release antibodies speci c to the antigen. Peak antibody levels are usually reached around 10 days after initial antigen exposure. 2. Secondary Immune Response: a. Second Exposure to an Antigen: The secondary immune response occurs upon re-exposure to the same antigen following the primary immune response. This can occur during subsequent infections with the same pathogen or through vaccination with a weakened or inactivated form of the pathogen. b. Faster Response: The secondary immune response is characterized by a faster and more robust reaction compared to the primary response. Memory B cells, generated during the primary response, are rapidly reactivated upon antigen re-encounter and differentiate into plasma cells more quickly than naïve B cells. This results in a more rapid production of antibodies and a more ef cient clearance of the antigen. c. Immunological Memory – Provided by Memory Cells: The enhanced secondary immune response is due to the presence of memory B cells, which persist long-term after the resolution of the primary response. Memory B cells exhibit a heightened state of readiness and can rapidly differentiate into plasma cells upon re-exposure to the antigen, providing immunological memory against the pathogen. d. Times Change to Hours Instead of Days: Due to the presence of memory B cells and a more ef cient immune response, the lag period observed in the primary immune response is signi cantly reduced during secondary immune responses. Antibody levels rise more rapidly, often within hours of re-exposure to the antigen, leading to faster and more effective protection against the pathogen. C. Active and Passive Humoral Immunity: Active Humoral Immunity: a. Naturally Acquired: Naturally acquired active humoral immunity occurs when the immune system responds to exposure to a pathogen during infection. Following infection, B cells recognize the pathogen’s antigens, undergo clonal selection and expansion, and differentiate into plasma cells that produce antibodies speci c to the pathogen. This immune response leads to the development of immunological memory, providing long-term protection against reinfection with the same pathogen. b. Arti cially Acquired – Vaccine: Arti cially acquired active humoral immunity is induced through vaccination with antigens derived from pathogens or their products. Vaccines stimulate the immune system to mount an immune response without causing the full-blown disease. There are two main types of vaccines: 1) Vaccines from Dead Viruses: Inactivated or killed vaccines contain whole viruses that have been rendered non-infectious through chemical or physical methods. These vaccines typically contain intact viral particles that are unable to replicate but can still stimulate an immune response, leading to the production of antibodies and the development of immunological memory. 2) Vaccines from Attenuated Viruses: Live attenuated vaccines contain weakened forms of the virus that are still capable of replicating within fi fi fi fi fi the body but cause minimal or no disease. These vaccines closely mimic natural infection and induce a robust immune response, including the production of antibodies and the generation of immunological memory. 2. Passive Humoral Immunity: Passive humoral immunity involves the transfer of pre-formed antibodies from one individual to another, providing immediate but temporary protection against speci c pathogens. Passive immunity can be acquired naturally or arti cially. Naturally Acquired: Naturally acquired passive humoral immunity occurs when antibodies are transferred from a mother to her offspring across the placenta during pregnancy or through breastfeeding. These maternal antibodies provide temporary protection to the newborn against certain pathogens until the infant’s immune system matures and begins producing its antibodies. Arti cially Acquired: Arti cially acquired passive humoral immunity involves the administration of pre-formed antibodies derived from immune individuals or animals to non-immune individuals. This approach is used in situations where immediate protection is needed, such as post-exposure prophylaxis for certain infectious diseases or treatment of immunode cient individuals. Passive immunity is temporary, as the transferred antibodies eventually degrade and are eliminated from the recipient’s body. IV. Antibodies: A. Immunoglobulins (Igs): Immunoglobulins, also known as antibodies, are glycoprotein molecules produced by B lymphocytes (B cells) in response to the presence of speci c antigens. Antibodies are an essential component of the immune system and play various roles in recognizing, neutralizing, and eliminating pathogens, toxins, and other foreign substances. B. Antibody Structure: 1.Antibody Monomer: a. Heavy Chain (H): Antibodies consist of two identical heavy chains (H) and two identical light chains (L) linked together to form a Y-shaped structure. Each heavy chain contains multiple domains, including the variable (V) region and the constant (C) region. The heavy chains determine the class and functional properties of the antibody. b. Light Chain (L): Light chains are smaller polypeptide chains that contain a variable (V) region and a constant (C) region. Light chains associate with heavy chains to form the antigen-binding site of the antibody. 2. Variable Region: The variable region of the antibody, located at the tips of the Y-shaped structure, is highly diverse and contains antigen-binding sites that recognize and bind to speci c epitopes (antigenic determinants) on antigens. The variable regions of both the heavy and light chains contribute to the speci city and diversity of antibody recognition. 3. Constant Region: The constant region of the antibody, located at the base of the Y-shaped structure, determines the antibody’s effector functions and interactions with other components of the immune system. The constant region of the heavy chain determines the antibody’s class fi fi fi fi fi fi (isotype), which includes IgM, IgG, IgA, IgD, and IgE, each with distinct functional properties. 4. Antigen-Binding Site: The antigen-binding site, also known as the paratope, is formed by the variable regions of the heavy and light chains and is complementary in shape to the antigenic epitope. The antigen-binding site confers speci city to the antibody, allowing it to recognize and bind to speci c antigens with high af nity. C. Antibody Classes: 1.IgM (Immunoglobulin M): IgM is the rst antibody class produced during the primary immune response to an antigen. It is primarily found as a pentamer, consisting of ve monomeric subunits joined together by disul de bonds and a J chain. IgM is ef cient at agglutinating (clumping) pathogens and activating the complement system, leading to their elimination. It is particularly effective at neutralizing viruses and bacteria in the bloodstream. IgM is also involved in the early stages of B cell activation. 2. IgA (Immunoglobulin A): IgA is the predominant antibody class found in mucosal secretions, such as saliva, tears, breast milk, and mucus membranes of the respiratory, gastrointestinal, and genitourinary tracts. It exists in two main forms: secretory IgA, which is dimeric and includes a secretory component that facilitates its transport across mucosal epithelial cells, and serum IgA, which is primarily monomeric and circulates in the bloodstream. IgA plays a crucial role in mucosal immunity by preventing pathogen attachment to mucosal surfaces, neutralizing toxins, and promoting the clearance of pathogens through mucociliary clearance and the actions of phagocytes. 3. IgD (Immunoglobulin D): IgD is found in low concentrations in the bloodstream and on the surface of naïve B cells as a monomer. Its function is not fully understood, but it is believed to play a role in B cell activation and maturation, possibly by acting as a co-receptor with the B cell receptor (BCR) to enhance antigen recognition and signaling. IgD may also have a regulatory role in the immune response. 4. IgG (Immunoglobulin G): IgG is the most abundant antibody class in the bloodstream, accounting for approximately 75-80% of total circulating antibodies. It is the main antibody involved in secondary immune responses and provides long-term immunity against pathogens. IgG is highly versatile and has multiple functions, including neutralization of pathogens, opsonization for phagocytosis, activation of the complement system, and crossing the placenta to provide passive immunity to the fetus during pregnancy. There are four subclasses of IgG (IgG1, IgG2, IgG3, and IgG4), each with distinct effector functions. 5. IgE (Immunoglobulin E): IgE is present in very low concentrations in the bloodstream but plays a crucial role in allergic reactions and defense against parasitic infections. IgE binds to high-af nity Fc receptors on the surface of mast cells and basophils, sensitizing these cells to allergens. Upon reexposure to the allergen, cross-linking of IgE molecules on mast cells and basophils triggers the release of in ammatory mediators, such as histamine, leading to allergic symptoms. IgE also contributes to the immune response against parasitic worms by activating eosinophils and fi fi fi fi fi fi promoting their effector functions. D. Antibody Targets and Functions: 1. Neutralization: Neutralization is the process by which antibodies bind to antigens, such as viruses or toxins, and block their ability to infect host cells or exert their harmful effects. By binding to critical sites on the antigen, antibodies prevent the antigen from interacting with host cells or tissues, thereby neutralizing its activity. Neutralization is an essential mechanism for preventing infection and limiting the spread of pathogens in the body. 2. Agglutination: Agglutination is the clumping together of pathogens, such as bacteria or red blood cells, due to the binding of antibodies to multiple antigens on their surface. Antibodies can cross-link antigens on the surface of pathogens, forming large aggregates that are easier for immune cells to engulf and remove through phagocytosis. Agglutination enhances the ef ciency of immune clearance by concentrating pathogens and promoting their recognition and elimination by phagocytes. 3. Precipitation: Precipitation is the process by which antibodies bind to soluble antigens, forming antigen-antibody complexes that become insoluble and precipitate out of solution. This reduces the solubility of antigens and facilitates their clearance by immune cells or physical removal from the body through mucociliary clearance or phagocytosis. Precipitation is particularly relevant in the clearance of soluble antigens, such as microbial toxins or soluble proteins, from the bloodstream and extracellular spaces. 4. Complement Activation: Complement activation is a cascade of enzymatic reactions triggered by the binding of antibodies to antigens, leading to the activation of the complement system. The complement system consists of a series of plasma proteins that work together to opsonize pathogens for phagocytosis, induce in ammation, and directly lyse pathogens through the formation of membrane attack complexes (MACs). Antibodies can activate the classical pathway of complement activation by binding to antigens and recruiting complement proteins, leading to the ampli cation and coordination of the immune response against pathogens. 5. Plan of Action:The plan of action outlined involves the sequential engagement of various antibody functions to effectively combat pathogens: a. Precipitation: Antibodies bind to soluble antigens, forming antigen-antibody complexes that become insoluble and precipitate out of solution, reducing the availability of the antigen. b.Lysis (by complement): Antibodies activate the complement system, leading to the formation of membrane attack complexes (MACs) on the surface of pathogens, causing their lysis and destruction. c. Agglutination: Antibodies cross-link multiple pathogens together, forming large aggregates that are easier for immune cells to recognize and engulf. d. Neutralization: Antibodies bind to pathogens or toxins, blocking their ability to infect host cells or exert their harmful effects, thereby fl fi neutralizing their activity and preventing further damage. V. Cellular Immunity: A. T Cells: T Helper Cells (Th) - CD4: T helper cells, also known as CD4+ T cells, play a central role in orchestrating the immune response by secreting cytokines and providing help to other immune cells, including B cells, cytotoxic T cells, and macrophages. They recognize antigens presented by class II major histocompatibility complex (MHC-II) proteins on the surface of antigen-presenting cells (APCs) and become activated, leading to their proliferation and differentiation into different subsets, such as Th1, Th2, Th17, and T follicular helper (Tfh) cells. Each subset of T helper cells has distinct functions in regulating immune responses, including the activation of macrophages (Th1), the promotion of antibody production (Th2 and Tfh), and the induction of in ammation (Th17). Cytotoxic T Cells (Tc) - CD8: Cytotoxic T cells, also known as CD8+ T cells, are specialized effector cells that recognize and eliminate infected or abnormal cells by inducing apoptosis. Cytotoxic T cells recognize antigens presented by class I major histocompatibility complex (MHC-I) proteins on the surface of target cells, such as virus-infected cells or cancer cells. Upon activation, cytotoxic T cells release cytotoxic molecules, such as perforin and granzymes, which induce apoptosis in target cells, leading to their destruction. Cytotoxic T cells play a crucial role in immune surveillance and defense against intracellular pathogens and tumor cells. Regulatory T Cells (Treg) - CD4: Regulatory T cells, also known as Tregs or CD4+CD25+ T cells, are a subset of T cells that suppress immune responses and maintain immune tolerance to self-antigens and harmless foreign antigens. Tregs play a critical role in preventing autoimmunity, allergies, and excessive in ammation by inhibiting the activation and proliferation of effector T cells and other immune cells. Tregs exert their suppressive functions through various mechanisms, including the secretion of anti-in ammatory cytokines, such as interleukin-10 (IL-10) and transforming growth factor-beta (TGF-β), and direct cell-to-cell contact with target cells. B. MHC Proteins: Class I MHC Proteins: Class I major histocompatibility complex (MHC-I) proteins are cell surface glycoproteins found on almost all nucleated cells in the body. They play a central role in presenting intracellular antigens, such as viral or tumor antigens, to cytotoxic T cells (CD8+ T cells). MHC-I proteins bind to endogenous antigens derived from cytosolic proteins and present them on the cell surface for recognition by cytotoxic T cells. This interaction between MHC-I proteins and cytotoxic T cells is crucial for immune surveillance and the elimination of infected or abnormal cells. Class II MHC Proteins: Class II major histocompatibility complex (MHC-II) proteins are cell surface glycoproteins primarily found on antigen-presenting cells (APCs), such as dendritic cells, macrophages, and B cells. They play a key role in presenting extracellular antigens, such as microbial peptides, to helper T cells (CD4+ T cells). MHC-II proteins bind to exogenous antigens that have been engulfed and processed by APCs and fl fl fl present them on the cell surface for recognition by helper T cells. This interaction between MHC-II proteins and helper T cells is essential for initiating and regulating adaptive immune responses, including the activation of B cells and cytotoxic T cells. VI. Failure of the Immune System: A. Immunode ciencies: 1. Absent or Depressed Immune System: Immunode ciencies refer to conditions in which the immune system is compromised or unable to function properly, leading to an increased susceptibility to infections and other immune-related disorders. These conditions can be congenital (present at birth) or acquired (develop later in life) and may result from genetic defects, medical treatments (such as chemotherapy), or infections (such as HIV). Individuals with immunode ciencies may have reduced numbers or impaired function of immune cells, such as T cells, B cells, or phagocytes, making them more susceptible to recurrent or severe infections. 2. Examples: Acquired Immunode ciency Syndrome (AIDS): AIDS is caused by the human immunode ciency virus (HIV), which targets and destroys CD4+ T cells, leading to progressive immune system failure and increased susceptibility to opportunistic infections and cancers. DiGeorge Syndrome: DiGeorge Syndrome is a genetic disorder characterized by the absence or underdevelopment of the thymus and parathyroid glands, leading to immune de ciencies, cardiac defects, and other developmental abnormalities. B. Autoimmune Diseases: 1. The Immune System Attacks Self: Autoimmune diseases occur when the immune system mistakenly targets and attacks healthy tissues and organs in the body, leading to in ammation, tissue damage, and dysfunction. These conditions result from a breakdown in selftolerance, the immune system’s ability to distinguish between self and non-self antigens. Autoimmune diseases can affect multiple organ systems and may have diverse clinical manifestations, ranging from mild to severe. 1. Examples: Rheumatoid Arthritis (RA): RA is a chronic in ammatory autoimmune disorder that primarily affects the joints, causing pain, swelling, stiffness, and progressive joint damage. It is characterized by the in ltration of immune cells into the synovial membrane, leading to synovitis and destruction of cartilage and bone. Hashimoto’s Disease: Hashimoto’s disease, also known as autoimmune thyroiditis, is an autoimmune disorder characterized by the immune-mediated destruction of the thyroid gland, leading to hypothyroidism. It results in symptoms such as fatigue, weight gain, cold intolerance, and thyroid enlargement (goiter). Systemic Lupus Erythematosus (SLE): SLE is a chronic autoimmune disease that can affect multiple organ systems, including the skin, joints, kidneys, and cardiovascular system. It is characterized by the production of autoantibodies and immune complexes, leading to widespread in ammation and tissue damage. Myasthenia Gravis: Myasthenia gravis is an autoimmune neuromuscular disorder characterized by muscle weakness and fatigue, particularly affecting the muscles that control eye movements, facial expressions, chewing, swallowing, and breathing. It is caused by fi fi fi fl fi fl fi fi fl autoantibodies that target and block acetylcholine receptors at the neuromuscular junction. Multiple Sclerosis (MS): MS is a chronic autoimmune in ammatory disorder of the central nervous system, characterized by the destruction of myelin, the protective covering of nerve bers. It results in symptoms such as muscle weakness, impaired coordination, sensory disturbances, and cognitive de cits. C. Hypersensitivities: 1. Caused by an Allergen: Hypersensitivities, also known as allergic reactions, occur when the immune system overreacts to harmless substances, called allergens, leading to in ammation and tissue damage. These reactions can range from mild to severe and may affect various organ systems, including the skin, respiratory system, gastrointestinal tract, and cardiovascular system. 2. Examples: Allergic Rhinitis: Allergic rhinitis, also known as hay fever, is an allergic reaction characterized by in ammation of the nasal mucosa in response to airborne allergens, such as pollen, dust mites, or pet dander. It results in symptoms such as sneezing, nasal congestion, runny nose, and itching of the eyes, nose, and throat. Anaphylaxis: Anaphylaxis is a severe and potentially life-threatening allergic reaction that can occur rapidly after exposure to an allergen, such as certain foods (e.g., peanuts, shell sh), medications (e.g., antibiotics, vaccines), or insect stings (e.g., bee venom). It can cause systemic symptoms, including dif culty breathing, swelling of the face and throat, low blood pressure, rapid heartbeat, and loss of consciousness, requiring immediate medical intervention. Respiration I. Functional Respiratory Anatomy A. Upper Respiratory 1.Nose a. Provides an airway for respiration b. Moistens and warms entering air c. Filters and cleans inspired air d. Serves as a resonating chamber for speech e. Houses olfactory (smell) receptors 2. External Nose Nostrils 3. Nasal Cavity a. Warming: The nasal cavity warms inspired air to body temperature before it reaches the lower respiratory tract. b. Moistening: Moisture is added to inspired air, preventing the respiratory mucosa from drying out. c. Filtering: Airborne particles and pathogens are ltered out by mucous membranes and cilia. d. Olfactory Receptors: Specialized nerve cells in the olfactory epithelium detect odors. fl fi fi fi fl fi fi e. Resonating Chamber: The nasal cavity contributes to the resonance of sound produced by the vocal cords. 4. Nasal conchae B. Respiration De nition: Respiration refers to the process of exchanging gases between the atmosphere, blood, and cells. It involves ventilation, which is the movement of air in and out of the lungs, as well as external respiration (the exchange of gases between the lungs and blood) and internal respiration (the exchange of gases between the blood and body tissues). II. Parts of the Respiratory System Pharynx: Common passageway for air and food. Divided into nasopharynx, oropharynx, and laryngopharynx. Larynx: Contains vocal cords and serves as a passageway for air between the pharynx and trachea. Trachea: Windpipe that carries air to and from the lungs. Bronchi and Bronchioles: Branches of the trachea that further divide into smaller airways called bronchioles, leading to the alveoli. Alveoli: Small air sacs in the lungs where gas exchange occurs. A. Ventilation: Inspiration: Inhalation, which involves the intake of air into the lungs. Expiration: Exhalation, which involves the expulsion of air from the lungs. Costal Breathing: Breathing primarily using the muscles of the chest wall. Diaphragmatic Breathing: Breathing primarily using the diaphragm. Compliance: Refers to the distensibility or elasticity of the lung tissue. B. Respiratory Physiology: Charles’ Law: Describes the relationship between the volume and temperature of gases. It explains how inhaled air is warmed during inspiration, contributing to the expansion of the lungs. Decompression Sickness: Also known as “the bends,” it occurs when dissolved gases (such as nitrogen) come out of solution in the bloodstream and form bubbles during rapid decompression, typically in scuba diving. C. Respiratory Center: Medullary Rhythmicity Area: Region in the brainstem responsible for generating the basic rhythm of breathing. Pneumotaxic Area and Apneustic Area: Brainstem centers involved in modifying the rhythm and depth of breathing. D. Oxygen Dissociation Curve: Describes the relationship between the partial pressure of oxygen (PO2) and the saturation of hemoglobin with oxygen. It illustrates how hemoglobin’s af nity for oxygen changes with different levels of PO2. Binding of CO to Hemoglobin: Carbon monoxide (CO) has a high af nity for hemoglobin, leading to the formation of carboxyhemoglobin, fi fi fi which reduces the oxygen-carrying capacity of the blood. III. LUNGS Anatomy of the Lungs: The lungs are paired organs located in the thoracic cavity on either side of the heart. They are enclosed by the rib cage and protected by the pleural membranes. Each lung is divided into lobes: the right lung has three lobes (upper, middle, and lower lobes), while the left lung has two lobes (upper and lower lobes). The lobes are further subdivided into smaller segments. The main structures of the lungs include the bronchial tree, alveoli, blood vessels, and supporting tissues. Bronchial Tree: The bronchial tree consists of a network of airways that carry air to and from the lungs. The trachea (windpipe) branches into two primary bronchi, one for each lung. The primary bronchi further divide into smaller bronchi, bronchioles, and ultimately terminate in clusters of alveoli. The bronchial tree is lined with ciliated epithelial cells and mucus-secreting goblet cells, which help trap and remove foreign particles and pathogens from the airways. Alveoli: Alveoli are small, thin-walled air sacs where gas exchange occurs between the air and the blood. Each alveolus is surrounded by a network of pulmonary capillaries, allowing for the diffusion of gases (oxygen and carbon dioxide) between the air and the bloodstream. The large surface area and thin walls of the alveoli facilitate ef cient gas exchange, maximizing the diffusion of oxygen into the bloodstream and carbon dioxide out of the bloodstream. Blood Supply: The lungs receive blood supply from the pulmonary circulation, which carries deoxygenated blood from the right side of the heart to the lungs for oxygenation. Oxygenated blood from the lungs is returned to the left side of the heart via the pulmonary veins, where it is then pumped to the rest of the body. Respiratory Function: The primary function of the lungs is to facilitate gas exchange, speci cally the uptake of oxygen from the air and the removal of carbon dioxide from the bloodstream. This gas exchange occurs through diffusion across the alveolar-capillary membrane, driven by differences in partial pressure of gases. In addition to gas exchange, the lungs also play a role in regulating acid-base balance, ltering out small blood clots, and metabolizing certain substances. Mechanics of Breathing: fi fi The lungs are elastic organs that expand and contract during breathing. Inspiration (inhalation) and expiration (exhalation) are driven by changes in intrathoracic pressure and the contraction of respiratory muscles, including the diaphragm and intercostal muscles. Regulation of Breathing: Breathing is regulated by the respiratory control centers located in the brainstem, which respond to neural, chemical, and mechanical signals to adjust respiratory rate and depth in response to changing metabolic demands and environmental conditions. IV. OXYGEN DISSOCIATION CURVE A. Sigmoidal Shape: The oxygen dissociation curve typically exhibits a sigmoidal (S-shaped) curve. This shape re ects the cooperative binding of oxygen to hemoglobin molecules. As oxygen molecules bind to one subunit of hemoglobin, it increases the af nity of the remaining subunits for oxygen, making it easier for subsequent oxygen molecules to bind. Conversely, as oxygen molecules are released from hemoglobin, it decreases the af nity of the remaining subunits, facilitating the release of additional oxygen molecules. B. Relationship between PO2 and SaO2: The x-axis of the curve represents the partial pressure of oxygen (PO2) in the blood, usually measured in millimeters of mercury (mmHg) or kilopascals (kPa). The y-axis represents the saturation of hemoglobin with oxygen (SaO2), expressed as a percentage. C. Plateau Phase: At high PO2 levels, such as those found in the lungs, hemoglobin is almost fully saturated with oxygen. This plateau phase of the curve indicates that small changes in PO2 have little effect on the saturation of hemoglobin. This high af nity of hemoglobin for oxygen in the lungs ensures ef cient loading of oxygen onto hemoglobin for transport to tissues. D. Steep Portion of the Curve: As PO2 decreases, such as in peripheral tissues where oxygen is being unloaded from hemoglobin, the curve becomes steeper. This steep portion of the curve indicates that small decreases in PO2 result in large decreases in the saturation of hemoglobin with oxygen. This phenomenon is known as the Bohr effect and is important for facilitating the release of oxygen from hemoglobin to tissues with high metabolic demands. E. Factors Affecting the Oxygen Dissociation Curve: pH: Changes in blood pH (acidity) can alter the shape and position of the oxygen dissociation curve. A decrease in pH (acidosis) shifts the curve to the right, reducing hemoglobin’s af nity for oxygen (referred to as the Bohr effect). Conversely, an increase in pH (alkalosis) shifts the curve to the left, increasing hemoglobin’s af nity for oxygen. Temperature: An increase in temperature shifts the curve to the right, reducing hemoglobin’s af nity for oxygen. This facilitates the release of oxygen to tissues during periods of increased metabolic activity. Carbon dioxide (CO2): Elevated levels of CO2 also shift the curve to the right, promoting the release of oxygen from hemoglobin. This fi fi fi fi effect is known as the Haldane effect and helps enhance the unloading of oxygen in tissues with high CO2 levels. Digestive System I. Overview of the Digestive System A. The Gastrointestinal Tract (GI): Mouth: The mouth is the entry point of food into the digestive system. It begins the process of mechanical digestion through chewing, breaking down food into smaller particles. Salivary glands secrete saliva, which contains enzymes (like amylase) that start the chemical breakdown of carbohydrates. Pharynx: Also known as the throat, it serves as a passageway for food and air. It plays a role in swallowing, pushing food from the mouth to the esophagus. Esophagus: A muscular tube connecting the pharynx to the stomach. Its primary function is to transport swallowed food (bolus) from the mouth to the stomach through peristalsis, rhythmic muscle contractions. Stomach: The stomach is a muscular sac located in the upper abdomen. It continues the mechanical digestion of food through muscular churning and mixes food with gastric juices, which contain hydrochloric acid and enzymes (like pepsin) for chemical digestion. The stomach also serves as a temporary storage site for food and regulates the release of partially digested food (chyme) into the small intestine. Small Intestine: The small intestine is where most of the digestion and absorption of nutrients occur. Divided into three parts: duodenum, jejunum, and ileum. Enzymes from the pancreas and bile from the liver aid in the breakdown of carbohydrates, proteins, and fats into molecules small enough to be absorbed. Villi and microvilli increase the surface area for nutrient absorption into the bloodstream. Large Intestine (Colon): The large intestine absorbs water and electrolytes from undigested food, forming feces. It houses bene cial bacteria that aid in the fermentation of undigested carbohydrates and the synthesis of certain vitamins, such as vitamin K and some B vitamins. The colon also plays a role in the storage and elimination of feces through the rectum and anus. fi B. The Accessory Digestive Organs: Teeth and Tongue: Teeth mechanically break down food into smaller pieces, increasing the surface area for chemical digestion. The tongue helps manipulate food during chewing and swallowing and assists in forming food into a bolus for swallowing. Salivary Glands: Salivary glands produce saliva, which contains enzymes that begin the digestion of carbohydrates and lubricate food for easier swallowing. Gallbladder: The gallbladder stores and concentrates bile, a digestive uid produced by the liver. Bile aids in the digestion and absorption of fats by emulsifying them into smaller droplets, increasing their surface area for enzymatic action. Liver: The liver performs numerous functions in digestion, metabolism, and detoxi cation. It produces bile, stores glycogen, synthesizes proteins, and metabolizes nutrients and drugs. The liver also detoxi es harmful substances and removes excess nutrients from the blood. Pancreas: The pancreas secretes digestive enzymes (amylase, lipase, proteases) into the small intestine to further break down carbohydrates, fats, and proteins. It also produces bicarbonate to neutralize the acidic chyme entering the small intestine from the stomach, creating an optimal pH for enzymatic activity. C. Structures of the Gastrointestinal Tract: 1. Oral Cavity (Buccal Cavity): a. Teeth: a. Structure: Teeth are composed of multiple layers. The outermost layer is enamel, a hard substance primarily made of calcium phosphate. Beneath the enamel is dentin, a dense bony tissue. The innermost portion is the pulp, which contains nerves and blood vessels. b. Dentition: Dentition refers to the arrangement of teeth in the mouth. Humans have two sets of teeth: primary (deciduous or baby) teeth and permanent (adult) teeth. Each set consists of different types of teeth, including incisors, canines, premolars, and molars, each with a speci c fi fl fi shape and function. c. Tooth Types: Incisors: Located at the front of the mouth, these teeth are used for cutting and biting food. Canines: Also known as cuspids, these pointed teeth are used for tearing and shredding food. Premolars (Bicuspids): These teeth have at surfaces and are used for grinding and crushing food. Molars: Located at the back of the mouth, molars have broad surfaces with multiple cusps and are used for grinding and chewing food. b. Tongue: The tongue is a muscular organ located in the oor of the mouth. It plays a crucial role in manipulating food during chewing and swallowing. Taste buds on the surface of the tongue detect taste sensations (sweet, salty, sour, bitter, and umami) and help in food selection and digestion. c. Hard and Soft Palate: The hard palate is the bony structure that forms the roof of the mouth. It separates the oral cavity from the nasal cavity and provides a rigid surface for the tongue to push against during chewing and swallowing. The soft palate is a muscular structure located behind the hard palate. It helps close off the nasal passages during swallowing to prevent food from entering the nasal cavity. d. Salivary Glands: Salivary glands produce saliva, a watery uid that moistens food, aids in swallowing, and initiates the digestion of carbohydrates. There are three pairs of major salivary glands: parotid glands (located near the ears), submandibular glands (located beneath the lower jaw), and sublingual glands (located beneath the tongue). Saliva contains enzymes, such as amylase, which begin the breakdown of starches into simpler sugars like maltose. Additionally, saliva contains antibacterial agents that help protect the oral cavity from infections. ack of the mouth, molars have broad surfaces with multiple cusps and are used for grinding and chewing food. b. Tongue: The tongue is a muscular organ located in the oor of the mouth. It plays a crucial role in manipulating food during chewing and swallowing. Taste buds on the surface of the tongue detect taste sensations (sweet, salty, sour, bitter, and umami) and help in food selection and digestion. c. Hard and Soft Palate: The hard palate is the bony structure that forms the roof of the mouth. It separates the oral cavity from the nasal cavity and provides a rigid surface for the tongue to push against during chewing and fl fl fl fl swallowing. The soft palate is a muscular structure located behind the hard palate. It helps close off the nasal passages during swallowing to prevent food from entering the nasal cavity. d. Salivary Glands: Salivary glands produce saliva, a watery uid that moistens food, aids in swallowing, and initiates the digestion of carbohydrates. There are three pairs of major salivary glands: parotid glands (located near the ears), submandibular glands (located beneath the lower jaw), and sublingual glands (located beneath the tongue). Saliva contains enzymes, such as amylase, which begin the breakdown of starches into simpler sugars like maltose. Additionally, saliva contains antibacterial agents that help protect the oral cavity from infections. and chewing food. b. Tongue: The tongue is a muscular organ located in the oor of the mouth. It plays a crucial role in manipulating food during chewing and swallowing. Taste buds on the surface of the tongue detect taste sensations (sweet, salty, sour, bitter, and umami) and help in food selection and digestion. c. Hard and Soft Palate: The hard palate is the bony structure that forms the roof of the mouth. It separates the oral cavity from the nasal cavity and provides a rigid surface for the tongue to push against during chewing and swallowing. The soft palate is a muscular structure located behind the hard palate. It helps close off the nasal passages during swallowing to prevent food from entering the nasal cavity. d. Salivary Glands: Salivary glands produce saliva, a watery uid that moistens food, aids in swallowing, and initiates the digestion of carbohydrates. There are three pairs of major salivary glands: parotid glands (located near the ears), submandibular glands (located beneath the lower jaw), and sublingual glands (located beneath the tongue). Saliva contains enzymes, such as amylase, which begin the breakdown of starches into simpler sugars like maltose. Additionally, fl fl fl saliva contains antibacterial agents that help protect the oral cavity from infections. 2. Esophagus: a. Upper Esophageal Sphincter: The upper esophageal sphincter (UES) is a muscular ring located at the upper end of the esophagus, near the pharynx. It functions as a valve that opens to allow food to pass from the pharynx into the esophagus during swallowing. After swallowing, the UES contracts to prevent food and uids from entering the airway. b. Peristalsis: Peristalsis is the coordinated, rhythmic contraction and relaxation of muscles that propels food through the digestive tract. In the esophagus, peristalsis begins when food is swallowed and triggers a wave of muscular contractions that push the food downward toward the stomach. Peristalsis ensures that food moves ef ciently through the esophagus and into the stomach for digestion. c. Segmentation: Segmentation is a mixing movement that occurs in the digestive tract, including the small intestine. Unlike peristalsis, which propels food in one direction, segmentation involves alternating contractions and relaxations of adjacent segments of the digestive tract. In the esophagus, segmentation does not play a signi cant role since its primary function is to transport food to the stomach rather than mixing and digestion. d. Lower Esophageal Sphincter (LES): The lower esophageal sphincter (LES), also known as the gastroesophageal or cardiac sphincter, is a muscular ring located at the lower end of the esophagus, near the entrance to the stomach. It acts as a valve that opens to allow food to pass from the esophagus into the stomach. The LES normally remains closed to prevent stomach contents, including acidic gastric juices, from re uxing (back owing) into the esophagus. Dysfunction of the LES can lead to gastroesophageal re ux disease (GERD), characterized by symptoms such as heartburn, regurgitation, and chest pain. 3. Stomach: a. Anatomical Divisions: The fundus is the upper portion of the stomach that bulges above the level of the cardia. The body is the central and largest part of the stomach, where most digestion occurs. The pylorus is the lower portion of the stomach that connects to the small intestine through the pyloric sphincter. fl The cardia is the region of the stomach closest to the esophagus and contains the lower esophageal sphincter (LES). fl fi The stomach can be anatomically divided into four main regions: the cardia, fundus, body, and pylorus. fi b. Sphincters: The stomach has two main sphincters: the lower esophageal sphincter (LES) and the pyloric sphincter. The LES, also known as the cardiac sphincter, regulates the passage of food from the esophagus into the stomach and prevents the back ow of stomach contents into the esophagus. The pyloric sphincter controls the release of partially digested food (chyme) from the stomach into the small intestine and regulates the rate of gastric emptying. c. Glands: The stomach contains several types of glands that secrete gastric juice, a mixture of hydrochloric acid, enzymes, and mucus. The gastric glands, located in the stomach lining, contain different types of cells that produce speci c components of gastric juice: Chief cells secrete pepsinogen, an inactive precursor of the enzyme pepsin, which breaks down proteins. Parietal cells secrete hydrochloric acid, which creates an acidic environment necessary for the activation of pepsin and the digestion of proteins. Mucous cells secrete mucus, which forms a protective barrier that prevents the stomach lining from being damaged by the acidic gastric juice. Enteroendocrine cells secrete hormones such as gastrin, which stimulates the secretion of gastric juice, and ghrelin, which regulates hunger and appetite. d. Chyme: Chyme is the semi-liquid mixture of partially digested food and gastric juice that is formed in the stomach. As food is churned and mixed with gastric juice in the stomach, it is gradually broken down into smaller particles. Chyme is released from the stomach into the small intestine in controlled amounts to ensure ef cient digestion and absorption of nutrients. 4. Intestines: a. Small Intestine: Anatomy: The small intestine is a long, coiled tube located between the stomach and the large intestine. It is divided into three segments: the duodenum, jejunum, and ileum. The duodenum is the shortest segment and is primarily responsible for receiving partially digested food from the stomach, mixing it with digestive enzymes and bile, and continuing the process of digestion. The jejunum and ileum are the longer segments where most of the absorption of nutrients occurs. They have numerous nger-like fl projections called villi and microvilli that greatly increase the surface area for absorption. Mechanical Digestion: Mechanical digestion in the small intestine primarily involves segmentation, a mixing movement that helps to thoroughly mix food with digestive enzymes and bring it into contact with the absorptive surface of the intestine. Contractions of circular muscles in the intestinal wall segment and mix the chyme, aiding in the digestion process and facilitating nutrient absorption. Chemical Digestion: Chemical digestion in the small intestine is facilitated by various enzymes produced by the pancreas and the small intestine itself. These enzymes break down complex molecules into simpler forms that can be absorbed. a.) Dextrinase: Dextrinase, also known as glucoamylase, is an enzyme that breaks down dextrins, which are intermediate products of starch digestion, into maltose, a disaccharide made of two glucose molecules. b.) Maltase: Maltase is an enzyme that hydrolyzes maltose into two glucose molecules. It is located on the surface of the microvilli in the small intestine. c.) Sucrase: Sucrase, also known as invertase, is an enzyme that breaks down sucrose (table sugar) into glucose and fructose, which can then be absorbed into the bloodstream. d.) Lactase: Lactase is an enzyme that catalyzes the breakdown of lactose, the sugar found in milk, into glucose and galactose. Individuals who are lactose intolerant have reduced or absent levels of lactase, leading to dif culty digesting dairy products. b. Large Intestine: 1) Cecum: The cecum is a pouch-like structure located at the beginning of the large intestine, where it connects to the small intestine. It serves as a site for the fermentation of indigestible carbohydrates and the absorption of water and electrolytes. The appendix is a small, nger-like projection attached to the cecum, although its function in humans is not fully understood. 2) Colon: The colon is the longest segment of the large intestine and is divided into several regions: the ascending colon, transverse colon, descending colon, and sigmoid colon. Its primary functions include absorbing water and electrolytes from undigested food, forming and storing feces, and facilitating the fi fi elimination of waste. 3) Rectum: The rectum is the terminal portion of the large intestine, located between the sigmoid colon and the anus. Its main function is to temporarily store feces until defecation occurs. The rectal walls contain stretch receptors that signal the need for defecation when feces accumulate. 4) Ileocecal Valve: The ileocecal valve is a sphincter located at the junction between the small intestine (ileum) and the large intestine (cecum). It regulates the passage of partially digested food (chyme) from the small intestine into the large intestine, preventing back ow. 5) Appendix: The appendix is a small, nger-shaped pouch attached to the cecum, near the junction with the small intestine. While its function in humans is not entirely clear, it is believed to play a role in the immune system as a reservoir for bene cial bacteria and lymphoid tissue. In some cases, the appendix can become in amed, leading to a condition known as appendicitis, which requires surgical removal (appendectomy). 5. Intestinal Crypts: a.) Enterocytes: Enterocytes are the most abundant cells lining the intestinal villi and crypts. They are specialized absorptive cells responsible for absorbing nutrients, electrolytes, and water from the intestinal lumen into the bloodstream. Enterocytes have microvilli on their apical surface, which greatly increase their surface area for nutrient absorption. b.) Goblet Cells: Goblet cells are specialized secretory cells found throughout the epithelium of the small and large intestines. They secrete mucus, a viscous uid that lubricates the intestinal lining and protects it from mechanical damage, chemical irritation, and pathogens. The mucus layer also helps facilitate the movement of food along the intestinal tract. c.) Enteroendocrine Cells: Enteroendocrine cells are specialized cells scattered throughout the epithelium of the small intestine and colon. They secrete various hormones that regulate digestive processes, appetite, and other physiological functions. Two important hormones secreted by enteroendocrine cells are: Secretin: Stimulates the pancreas to release bicarbonate-rich pancreatic juice, which helps neutralize acidic chyme entering the duodenum from the stomach. Cholecystokinin (CCK): Stimulates the release of digestive enzymes from the pancreas and bile from the gallbladder, as well as fl fl fi reducing gastric emptying and promoting satiety. d.) Paneth Cells: Paneth cells are specialized secretory cells located at the base of the intestinal crypts, primarily in the small intestine. They secrete antimicrobial peptides, including defensins and lysozymes, which help protect the intestinal epithelium from bacterial infections. Defensins disrupt bacterial cell membranes, while lysozymes break down bacterial cell walls. e.) Stem Cells: Stem cells are undifferentiated cells located at the base of the intestinal crypts, adjacent to Paneth cells. They continuously divide and differentiate to replenish the epithelial lining of the intestine, including enterocytes, goblet cells, enteroendocrine cells, and Paneth cells. Stem cells play a crucial role in maintaining the integrity and function of the intestinal epithelium, ensuring its constant renewal and repair. II. Accessory Structures of the Digestive Structure A. Pancreas: Exocrine Function: The exocrine function of the pancreas involves the secretion of digestive enzymes and bicarbonate into the duodenum to aid in the digestion of food. These digestive enzymes are produced by specialized cells called acinar cells, which make up the majority of the pancreas. Secretions – Acinar Cells: Acinar cells secrete several enzymes and bicarbonate ions into small ducts that merge to form the main pancreatic duct, which then joins the common bile duct before emptying into the duodenum through the hepatopancreatic ampulla (ampulla of Vater). a. Sodium Bicarbonate: Sodium bicarbonate is a buffer that neutralizes the acidic chyme entering the duodenum from the stomach, creating a more alkaline environment. This alkaline environment is necessary for the optimal activity of pancreatic enzymes and protects the duodenal mucosa from damage by gastric acid. b. Pancreatic Amylase: Pancreatic amylase is an enzyme that hydrolyzes starches and glycogen into maltose and other smaller carbohydrate molecules. It continues the digestion of carbohydrates initiated by salivary amylase in the mouth. c. Trypsin: Trypsin is a serine protease enzyme that hydrolyzes peptide bonds in proteins, breaking them down into smaller peptides. It is produced in an inactive form called trypsinogen, which is activated to trypsin by an enzyme called enterokinase in the duodenum. d. Chymotrypsin: Chymotrypsin is another serine protease enzyme produced by the pancreas, which hydrolyzes peptide bonds in proteins, particularly at the carboxyl ends of large, hydrophobic amino acids. Like trypsin, it is initially produced as an inactive precursor (chymotrypsinogen) and activated in the duodenum. e. Carboxypeptidase: Carboxypeptidase is an enzyme that hydrolyzes peptide bonds at the carboxyl end of peptides and proteins, releasing individual amino acids. It plays a role in the nal stages of protein digestion in the small intestine. f. Pancreatic Lipase: Pancreatic lipase is an enzyme that hydrolyzes triglycerides into fatty acids and monoglycerides, which can be absorbed by enterocytes in the small intestine. fi It is essential for the digestion and absorption of dietary fats. g. Ribonuclease and Deoxyribonuclease: Ribonuclease and deoxyribonuclease are enzymes that hydrolyze RNA and DNA, respectively, into nucleotides. They are involved in the digestion of nucleic acids present in food. B. Liver: 1. Anatomy: a. Lobes: The liver is divided into four lobes: the right lobe, left lobe, caudate lobe, and quadrate lobe. The right lobe is the largest and occupies most of the upper right quadrant of the abdomen, while the left lobe is smaller and located in the upper left quadrant. The caudate lobe is located posteriorly, near the inferior vena cava, and the quadrate lobe is situated anteriorly, adjacent to the gallbladder. b. Falciform Ligament: The falciform ligament is a fold of peritoneum that attaches the liver to the anterior abdominal wall and diaphragm. It separates the right and left lobes of the liver and contains the ligamentum teres (round ligament), a remnant of the fetal umbilical vein. c. Hepatocytes: Hepatocytes are the main functional cells of the liver and constitute about 60-80% of its cellular mass. They perform numerous metabolic, synthetic, and detoxi cation functions, including the production of bile, metabolism of nutrients, synthesis of plasma proteins, and detoxi cation of drugs and toxins. d. Kupffer Cells: Kupffer cells are specialized macrophages located within the sinusoids of the liver. They play a crucial role in immune surveillance and defense by engul ng and removing pathogens, foreign particles, and damaged cells from the bloodstream. 2. Blood Supply: a. Hepatic Artery: The hepatic artery supplies oxygenated blood to the liver, delivering nutrients and oxygen to hepatocytes for metabolic processes. It branches off from the celiac trunk, a major branch of the abdominal aorta. b. Hepatic Portal Vein: The hepatic portal vein carries nutrient-rich blood from the gastrointestinal tract, pancreas, and spleen to the liver for processing and detoxi cation. It transports absorbed nutrients, such as glucose, amino acids, and lipids, as well as metabolic byproducts, to the liver before they fi fi fi fi enter the systemic circulation. 3. Bile: a. Bile Salts: Bile salts are amphipathic molecules synthesized from cholesterol in the liver. They emulsify dietary fats into smaller droplets, increasing their surface area for enzymatic digestion by pancreatic lipase in the small intestine. Bile salts also facilitate the absorption of lipids, fat-soluble vitamins, and cholesterol in the small intestine. b. Bilirubin: Bilirubin is a yellow pigment derived from the breakdown of heme molecules in aged red blood cells. It is processed and excreted by hepatocytes into bile, where it contributes to the characteristic color of bile. Excess bilirubin can accumulate in the bloodstream, leading to jaundice, a yellowing of the skin and eyes. 4. Regulation: The liver plays a central role in metabolic regulation, maintaining glucose, lipid, and protein homeostasis. It responds to hormonal and neural signals to regulate blood glucose levels by storing glucose as glycogen (glycogenesis) or releasing glucose into the bloodstream (glycogenolysis and gluconeogenesis). The liver also regulates lipid metabolism by synthesizing and storing triglycerides, synthesizing cholesterol, and converting excess carbohydrates into fatty acids for storage or energy production. Additionally, it produces bile acids necessary for lipid digestion and absorption. Protein metabolism in the liver involves the synthesis of plasma proteins, such as albumin, clotting factors, and complement proteins, as well as the conversion of ammonia into urea for excretion. Detoxi cation of drugs, toxins, and metabolic byproducts occurs in hepatocytes, where they are metabolized and eliminated from the body through bile or urine. C. Gallbladder: Stores Bile: The gallbladder is a small, pear-shaped organ located beneath the liver. Its primary function is to store and concentrate bile, a digestive uid produced by the liver, until it is needed for digestion. Bile is released from the gallbladder into the small intestine in response to the ingestion of fatty foods. CCK – Stimulates Ejection of Bile: Cholecystokinin (CCK) is a hormone produced by enteroendocrine cells in the duodenum and jejunum in response to the presence of fats and proteins in the small intestine. CCK stimulates the contraction of the gallbladder and relaxation of the sphincter of Oddi (hepatopancreatic sphincter), facilitating the ejection of bile into the duodenum. fl fi This coordinated action ensures that bile is delivered to the small intestine to aid in the emulsi cation and digestion of fats. Cystic Duct: The cystic duct is a narrow tube that connects the gallbladder to the common bile duct, which carries bile to the small intestine. Bile ows from the gallbladder into the cystic duct when the gallbladder contracts in response to CCK stimulation. The cystic duct joins the common bile duct to form the bile duct, through which bile is transported to the duodenum. III. Ion Speci c Uptake in the Intestines: A. Iron Uptake in the Intestine: 1. Enterocytes: Enterocytes are specialized cells lining the epithelium of the small intestine responsible for the absorption of nutrients, including iron. They play a crucial role in the uptake, transport, and regulation of iron in the body. 2. Divalent Metal Transporter 1 (DMT1): Divalent Metal Transporter 1 (DMT1), also known as Nramp2 (Natural resistance-associated macrophage protein 2), is a transmembrane protein expressed on the apical membrane of enterocytes. It facilitates the uptake of divalent metals, including ferrous iron (Fe^2+), from the intestinal lumen into enterocytes. 3. Ferroportin: Ferroportin is a transmembrane protein located on the basolateral membrane of enterocytes. It functions as an iron exporter, transporting ferrous iron (Fe^2+) from enterocytes into the bloodstream, where it binds to transferrin for transport to various tissues and organs. 4. Ferritin: Ferritin is an intracellular protein complex composed of ferritin heavy chains and ferritin light chains. It serves as the primary storage form of iron in enterocytes and other cells. When iron levels are high, ferritin sequesters excess iron within enterocytes, preventing iron toxicity and maintaining cellular iron homeostasis. Conversely, when iron levels are low, ferritin releases stored iron to meet the body’s metabolic demands, ensuring a steady supply of iron for essential physiological processes. 5. Hepcidin: a. Regulates Iron Homeostasis: Hepcidin is a peptide hormone that plays a central role in the regulation of iron homeostasis. It controls the absorption, distribution, and release of iron in the body by regulating the activity of ferroportin, the iron exporter protein expressed on the surface of cells involved in iron transport. When hepcidin levels are high, it binds to ferroportin, leading to its internalization and degradation, thereby reducing iron export from cells and iron absorption from the intestine. fi Conversely, when hepcidin levels are low, ferroportin is not inhibited, allowing for increased iron export and absorption. fl b. Produced by the Liver: Hepcidin is primarily produced and secreted by hepatocytes, specialized cells of the liver. Hepatocytes sense systemic iron levels and respond by synthesizing and releasing hepcidin into the bloodstream. Hepcidin production is regulated by multiple factors, including iron stores, erythropoiesis, in ammation, and oxygen levels. c. De ciency Targets the Duodenum and Spleen: Hepcidin de ciency or dysfunction can lead to dysregulated iron metabolism and iron overload disorders, such as hereditary hemochromatosis. In hereditary hemochromatosis, mutations in genes involved in hepcidin regulation result in decreased hepcidin production or impaired hepcidin function. This leads to excessive iron absorption from the duodenum and increased release of iron from macrophages in the spleen, resulting in iron accumulation in various organs and tissues, including the liver, heart, pancreas, and joints. d. Hemochromatosis: Hemochromatosis is a hereditary or acquired disorder characterized by excessive iron absorption and accumulation in the body. Hereditary hemochromatosis, the most common form of the disorder, is caused by mutations in genes such as HFE, which regulate hepcidin production or function. As a result of hepcidin de ciency or dysfunction, individuals with hemochromatosis absorb more iron from the diet than is needed, leading to iron overload and toxicity in various organs. Symptoms of hemochromatosis can include fatigue, joint pain, abdominal pain, liver cirrhosis, diabetes, and heart failure. Treatment typically involves therapeutic phlebotomy (blood removal) to reduce iron levels, along with dietary modi cations and iron chelation therapy in some cases. 6. Ferroportin: a. Major Iron Export Protein: Ferroportin is the primary iron exporter protein found on the surface of cells involved in iron transport. It facilitates the ef ux of ferrous iron (Fe^2+) from cells into the bloodstream, allowing for the distribution of iron to various tissues and organs throughout the body. Ferroportin is expressed on the basolateral membrane of enterocytes in the small intestine, macrophages in the spleen and liver, and other cells involved in iron metabolism. b. Hepcidin Limits Iron Fluxes to the Bloodstream by Promoting Degradation of Ferroportin in Target Cells: Hepcidin is a peptide hormone produced by the liver that regulates iron homeostasis by controlling the activity of ferroportin. When hepcidin levels are elevated, such as in response to high iron stores or in ammation, hepcidin binds to ferroportin and induces its internalization and degradation. fl fi fl fi fi This leads to a reduction in ferroportin expression on the cell surface and decreased iron export from cells into the bloodstream. By inhibiting ferroportin, hepcidin limits the release of iron from enterocytes in the intestine and macrophages in the spleen and liver, thereby reducing iron uxes to the bloodstream and maintaining iron balance in the body. c. Transferrin: Transferrin is a glycoprotein found in the bloodstream that binds to and transports iron throughout the body. It serves as the primary iron transport protein, delivering iron from the intestines (via absorption) or from iron-storing cells (such as hepatocytes and macrophages) to tissues and organs that require iron for various physiological processes. Iron bound to transferrin is in the ferric (Fe^3+) state, which is more stable and less reactive than ferrous iron (Fe^2+), allowing for safe transport and delivery to target cells. B. Calcium Absorption: 1. Intestinal Absorption: a. Small Intestine: The majority of calcium absorption occurs in the small intestine, particularly in the duodenum and proximal jejunum. Calcium is absorbed through both paracellular and transcellular pathways, with the transcellular pathway being the primary route. b. Large Intestine: While the large intestine can absorb some calcium, its contribution to overall calcium absorption is minimal compared to the small intestine. 2. Paracellular: Paracellular absorption refers to the movement of calcium between epithelial cells through the tight junctions that connect them. In the paracellular pathway, calcium ions passively diffuse across the epithelial cell layer down their concentration gradient, driven by the electrochemical gradient created by active transport of other ions, such as sodium and potassium. Paracellular absorption is important for the uptake of calcium in the small intestine, particularly in regions where calcium concentrations are high. 3. Transcellular: Transcellular absorption involves the active transport of calcium ions across the apical and basolateral membranes of enterocytes (intestinal epithelial cells). This process requires the concerted action of several proteins, including calcium channels, calcium-binding proteins, and ATPdependent calcium pumps. Vitamin D plays a crucial role in regulating transcellular calcium absorption. When activated by sunlight or dietary intake, vitamin D3 (cholecalciferol) promotes the expression of proteins involved in calcium transport, such as calcium-binding protein (calbindin) and calcium ATPases, enhancing calcium absorption in the small intestine. Vitamin D3: fl Vitamin D3, also known as cholecalciferol, is a fat-soluble vitamin that plays a critical role in calcium homeostasis and bone health. Vitamin D3 is synthesized in the skin upon exposure to sunlight (UV-B radiation) and can also be obtained from dietary sources or supplements. In the intestines, vitamin D3 enhances calcium absorption by promoting the expression of proteins involved in transcellular calcium transport, such as calbindin and calcium ATPases. Additionally, vitamin D3 stimulates calcium absorption in the kidneys and regulates calcium reabsorption in the bones, helping to maintain calcium balance in the body. De ciency of vitamin D3 can lead to impaired calcium absorption, resulting in decreased bone mineral density and an increased risk of conditions such as osteoporosis and rickets. 4. Claudin: Claudins are a family of proteins that are integral components of tight junctions, which are specialized cell-cell junctions found in epithelial and endothelial tissues. Tight junctions seal the intercellular space between adjacent cells, regulating the passage of ions, solutes, and water across epithelial barriers. Claudins play a crucial role in determining the permeability and selectivity of tight junctions, in uencing the movement of molecules through the paracellular pathway. Different claudin isoforms exhibit varying degrees of tight junction sealing properties and ion selectivity, allowing for the ne-tuning of epithelial barrier function in different tissues and physiological conditions. In the context of calcium absorption, claudins contribute to the regulation of paracellular calcium transport across the epithelial cell layer of the small intestine, helping to maintain calcium homeostasis. 5. Calbindin: Calbindin is a calcium-binding protein that is expressed in various tissues, including the intestine, kidneys, and brain. In the context of calcium absorption, calbindin plays a critical role in the transcellular pathway of calcium transport across intestinal epithelial cells. Calbindin binds to calcium ions within the cytoplasm of enterocytes, facilitating their intracellular transport and delivery to the basolateral membrane for export into the bloodstream. By buffering intracellular calcium levels and enhancing calcium transport across the enterocyte, calbindin helps to maintain ef cient calcium absorption in the small intestine. The expression of calbindin is regulated by factors such as vitamin D, which promotes its synthesis in response to increased calcium fi demand, thereby enhancing calcium absorption in the intestines.