Earth Materials and the Rock Cycle PDF
Document Details
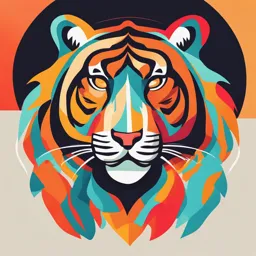
Uploaded by HaleJasper9450
Tags
Summary
This document provides an overview of Earth materials and the rock cycle. Topics covered include geologic time, Earth's interior, and plate tectonics. The document is likely a lecture or textbook.
Full Transcript
Chapter 11: Earth Materials and the Rock Cycle 1. Geologic Time and Change Time Divisions: ○ Eons: Largest division of time (e.g., Precambrian, Phanerozoic). ○ Eras: Subdivisions of eons (e.g., Paleozoic, Mesozoic, Cenozoic). ○ Periods and Epochs: Smaller d...
Chapter 11: Earth Materials and the Rock Cycle 1. Geologic Time and Change Time Divisions: ○ Eons: Largest division of time (e.g., Precambrian, Phanerozoic). ○ Eras: Subdivisions of eons (e.g., Paleozoic, Mesozoic, Cenozoic). ○ Periods and Epochs: Smaller divisions providing detailed timelines. Principle of Uniformitarianism: ○ Earth processes like erosion, volcanic eruptions, and sediment deposition operate consistently over time. ○ Contrasts with earlier views that all changes occurred through sudden, catastrophic events. ○ Evidence includes sediment layers and fossils. 2. Earth’s Interior Structure: ○ Crust: Continental Crust: 35-70 km thick, composed mainly of granite. Oceanic Crust: ~7 km thick, denser, composed mainly of basalt. ○ Mantle: Extends to ~2,900 km depth. Composed of silicate minerals rich in magnesium and iron. Convection currents drive plate tectonics. ○ Core: Outer core (liquid iron-nickel) generates the magnetic field. Inner core (solid iron-nickel) remains solid due to immense pressure. Asthenosphere and Lithosphere: ○ Lithosphere: Rigid outer shell (crust + upper mantle). ○ Asthenosphere: Ductile, allows tectonic plates to move over it. Earth’s Magnetism: ○ Generated by convection in the liquid outer core. ○ Protects Earth from solar wind and creates the magnetosphere. 3. Earth Materials and the Rock Cycle Igneous Processes: ○ Form from cooling and solidification of magma/lava. ○ Intrusive Igneous Rocks: Form below the surface; slow cooling leads to large crystals (e.g., granite). ○ Extrusive Igneous Rocks: Form at the surface; rapid cooling results in small crystals (e.g., basalt). ○ Landforms: Batholiths, sills, dikes, volcanic necks. Sedimentary Processes: ○ Sediments (from weathering/erosion) compacted and cemented over time. ○ Types: Clastic: Made of rock fragments (e.g., sandstone). Chemical: Precipitated from solution (e.g., limestone). Organic: Composed of biological material (e.g., coal). Metamorphic Processes: ○ Rocks altered by heat, pressure, or chemically active fluids. ○ Types: Foliated: Minerals aligned (e.g., schist, gneiss). Non-foliated: No alignment (e.g., marble, quartzite). The Rock Cycle: ○ Interactions among rock types: Igneous rocks weather into sediments. Sediments lithify into sedimentary rocks. Rocks buried and subjected to heat/pressure become metamorphic. Melting forms magma, restarting the cycle. 4. Plate Tectonics Continental Drift: ○ Theory by Alfred Wegener: Evidence: Fossil distribution (e.g., Mesosaurus in South America and Africa). Matching geological formations across continents. Paleoclimate indicators (e.g., glacial deposits in warm regions). ○ Supercontinent Pangaea existed ~250 million years ago. Seafloor Spreading: ○ New crust forms at mid-ocean ridges due to upwelling magma. ○ Magnetic striping reveals reversals in Earth’s magnetic field. ○ Older crust subducts at ocean trenches, recycling material. Subduction and Plate Motion: ○ Denser oceanic crust sinks beneath less dense crust at convergent boundaries. ○ Generates volcanic arcs and earthquakes. Plate Boundaries: ○ Divergent Boundaries: Plates move apart (e.g., Mid-Atlantic Ridge). Creates new crust and rift valleys. ○ Convergent Boundaries: Plates collide; outcomes depend on crust types: Oceanic-oceanic: Island arcs (e.g., Japan). Oceanic-continental: Volcanic mountain ranges (e.g., Andes). Continental-continental: High mountain ranges (e.g., Himalayas). ○ Transform Boundaries: Plates slide past each other (e.g., San Andreas Fault). Creates strike-slip faults and frequent earthquakes. Earthquake and Volcanic Activity: ○ Most earthquakes and volcanoes occur at plate boundaries due to crustal interactions. ○ Seismology reveals plate movement dynamics. Hot Spots: ○ Stationary mantle plumes form volcanic islands (e.g., Hawaiian Islands). ○ Track plate motion as islands are carried away from the hotspot. 5. The Geologic Cycle Tectonic Cycle: ○ Processes that build Earth’s surface (e.g., mountain building, volcanism). Rock Cycle: ○ Transformation among igneous, sedimentary, and metamorphic rocks. Hydrologic Cycle: ○ Movement of water through evaporation, precipitation, and runoff. Erosion and Deposition: ○ Shaping Earth’s surface via rivers, glaciers, and wind. 6. Endogenic and Exogenic Processes Endogenic (Internal): ○ Driven by Earth’s internal heat from radioactive decay. ○ Includes tectonics, earthquakes, and volcanism. Exogenic (External): ○ Driven by solar energy and gravity. ○ Includes weathering, erosion, and deposition by water, wind, and ice. ○ Chapter 12: Crustal Deformation, Earthquakes, and Volcanism Crustal Formation Continental Shields: ○ Shields represent the ancient core areas of continents. ○ Composed of igneous and metamorphic rocks formed billions of years ago. ○ They are tectonically stable and often covered by younger sedimentary layers at their edges. ○ Examples: Canadian Shield: The exposed Precambrian rock in North America. African Shield: Found across large parts of Africa. Building Continental Crust: ○ Processes contributing to crustal growth: Volcanic Activity: Adds new material from magma to the surface. Sediment Deposition: Layers accumulate over time, compacting into sedimentary rock. Tectonic Uplift: Raises crustal material, exposing it to surface processes. ○ New crust forms primarily at subduction zones and mid-ocean ridges. Accretion of Terranes: ○ Occurs when small crustal fragments (terranes) are transported by tectonic plate motion and "stuck" onto larger continental masses. ○ Identifiable by mismatched geology compared to surrounding areas. ○ Examples: The western coast of North America has numerous terranes of Pacific origin. New Zealand is composed of accreted terranes from different geologic ages. Crustal Deformation Folding and Broad Warping: ○ Folding: Caused by compressional forces where rocks deform plastically. Anticlines: Upward-arching folds. Synclines: Downward-trough folds. Example: The Valley and Ridge province of the Appalachians. ○ Broad Warping: Uplift or subsidence over large areas due to mantle processes or isostatic adjustments. Example: Broad uplift of regions previously covered by glaciers. Faulting: ○ Faults are fractures in the crust where displacement occurs. ○ Types of Faults: Normal Faults: Form under tensional forces; the hanging wall moves downward (e.g., East African Rift Valley). Reverse Faults: Form under compressional forces; the hanging wall moves upward (e.g., Himalayas). Strike-Slip Faults: Horizontal movement due to shear forces (e.g., San Andreas Fault in California). ○ Faulting creates landforms such as escarpments, horsts (uplifted blocks), and grabens (down-dropped blocks). Mountain Building Mountain Building at Plate Boundaries: ○ Oceanic–Continental Convergence: Denser oceanic plate subducts beneath lighter continental plate. Forms volcanic mountain chains (e.g., Andes Mountains). ○ Oceanic–Oceanic Convergence: One oceanic plate subducts beneath another, creating island arcs (e.g., Mariana Islands). ○ Continental–Continental Convergence: Both plates are buoyant and resist subduction, leading to intense folding and uplift. Example: Himalayas formed by the collision of the Indian and Eurasian plates. Examples of Orogenies: ○ Laramide Orogeny: Formed the Rocky Mountains in the western U.S. ○ Appalachian Orogeny: Resulted from ancient continental collisions during the formation of Pangaea. Earthquakes Fault Mechanics and Earthquake Anatomy: ○ Stress: Build-up of force in the Earth's crust. ○ Strain: Deformation resulting from stress. ○ When stress exceeds rock strength, fault rupture occurs. ○ Key features: Focus (Hypocenter): Point within the Earth where the earthquake originates. Epicenter: Surface point directly above the focus. Earthquake Intensity and Magnitude: ○ Intensity: Measures the effects on people, buildings, and the landscape (Modified Mercalli Scale). ○ Magnitude: Measures the energy released at the source (Richter or Moment Magnitude Scale). ○ Ground Shaking: Stronger near the epicenter; depends on magnitude, depth, and local geology. Case Studies: ○ Japan Earthquake and Tsunami (2011): 9.1 magnitude; caused by subduction along the Japan Trench. Generated a devastating tsunami, impacting nuclear facilities. ○ Haiti Earthquake (2010): 7.0 magnitude; high casualty rate due to poor construction practices. ○ Nepal Earthquake (2015): 7.8 magnitude; caused widespread damage and triggered landslides. Human-Induced Earthquakes: ○ Triggered by activities like fluid injection (fracking), reservoir filling, and geothermal energy extraction. Volcanism Settings for Volcanic Activity: ○ Divergent Boundaries: Volcanism at mid-ocean ridges (e.g., Iceland). ○ Convergent Boundaries: Subduction zones with stratovolcanoes (e.g., Mount Fuji). ○ Hot Spots: Stationary mantle plumes (e.g., Yellowstone, Hawaii). Volcanic Materials: ○ Lava: Basaltic Lava: Low viscosity, forms shield volcanoes. Andesitic/Rhyolitic Lava: High viscosity, associated with explosive eruptions. ○ Pyroclastics: Includes ash, pumice, lapilli, and volcanic bombs. ○ Volcanic Gases: Water vapor, carbon dioxide, and sulfur dioxide. Volcanic Landforms: ○ Shield Volcanoes: Wide, gently sloping; formed by basaltic lava (e.g., Mauna Loa). ○ Composite Volcanoes (Stratovolcanoes): Steep, alternating layers of lava and pyroclastics (e.g., Mount St. Helens). ○ Cinder Cones: Small, steep cones of pyroclastic material (e.g., Sunset Crater). ○ Flood Basalts: Large-scale basaltic flows (e.g., Deccan Traps). Eruption Types: ○ Effusive Eruptions: Gentle, with extensive lava flows (e.g., Kilauea). ○ Explosive Eruptions: Violent, producing ash clouds and pyroclastic flows (e.g., Krakatoa). Volcano Forecasting and Planning:Volcano Forecasting and Planning: Monitoring seismic activity, gas emissions, and thermal anomalies. Effective planning includes evacuation routes and hazard mapping. Chapter 13: Weathering, Karst Landscapes, and Mass Movement Basic Concepts for Landform Study Landforms and Dynamic Equilibrium: ○ Landforms are in a state of dynamic equilibrium, where processes of erosion and deposition work together to create stable features over time. For example, mountains are eroded by wind and water, but new material is constantly being deposited to maintain their shape. ○ Magnitude and Frequency: Refers to the size and frequency of geological events (e.g., large storms, earthquakes) that influence landforms. More frequent smaller events may cause more gradual changes, while rare but large events can have dramatic effects. ○ Forces at Work on Slopes: Gravity plays a central role in slope dynamics. On a slope, materials are held in place by cohesive forces (e.g., friction and particle attraction) and are driven to move by gravity. If the gravitational force exceeds the resisting forces, mass movement (erosion or landslides) will occur. Weathering Processes Weathering refers to the breakdown of rocks and minerals at Earth's surface. It can be categorized into physical and chemical processes: Factors Influencing Weathering: ○ Climate: Warm, wet climates accelerate chemical weathering, while cold, dry climates favor physical weathering (e.g., freeze-thaw cycles). ○ Rock Composition: Hard rocks like granite weather slower than softer rocks like sandstone. ○ Topography: Steeper slopes can accelerate physical weathering processes like rock falls or slides, while flatter terrain can retain soil moisture, promoting chemical weathering. ○ Vegetation: Plants contribute to weathering by producing organic acids and through root expansion. Physical Weathering Processes Physical weathering involves the mechanical breakdown of rocks without altering their chemical composition: Frost Wedging (Freeze-Thaw Action): ○ Water enters cracks in rocks. When temperatures drop, the water freezes, expanding by about 9% in volume. This expansion exerts pressure on the rock, causing it to fracture. This process repeats when temperatures fluctuate, resulting in the gradual disintegration of rocks. Salt-Crystal Growth (Salt Weathering): ○ In arid climates, evaporating water leaves behind salt crystals in rock pores. As the crystals grow, they exert pressure on the surrounding rock, causing it to break apart. This is particularly common along coastal areas where seawater evaporates. Exfoliation (Pressure-Release Jointing): ○ Exfoliation occurs when rocks are brought to the surface from deep within the Earth, where pressure is high. When the pressure is released (as the rock moves towards the surface), it causes the outer layers to peel off in thin sheets, similar to the way an onion skin peels. Chemical Weathering Processes Chemical weathering changes the chemical composition of minerals, often creating new minerals and solutes: Hydration and Hydrolysis: ○ Hydration occurs when minerals absorb water, causing them to expand and break apart. ○ Hydrolysis is a process where minerals react with water and form a new mineral (e.g., feldspar turning into clay minerals). Oxidation: ○ In oxidation, minerals containing iron (like olivine) react with oxygen and water, causing the iron to rust and turn reddish-brown. This weakens the rock, making it more prone to disintegration. Dissolution (Carbonation): ○ Carbonation happens when carbon dioxide from the atmosphere reacts with water to form carbonic acid, which dissolves limestone and other carbonate minerals. Over time, this creates caves, sinkholes, and other karst features. Spheroidal Weathering: ○ A specific form of weathering where angular rock fragments become rounded as weathering attacks the edges and corners more than the flat faces, resulting in a more spherical shape. Differential Weathering Differential Weathering occurs when different types of rocks weather at different rates, leading to uneven terrain. Softer rocks are weathered more quickly than harder rocks, creating features like valleys and ridges. For instance, limestone weathers more easily than granite, leading to a jagged landscape. Karst Topography Karst landscapes are formed by the dissolution of soluble bedrock such as limestone, dolomite, or gypsum. These landscapes often feature underground drainage systems and unique surface features. Formation of Karst: ○ Karst topography forms when water reacts with soluble rocks. Over time, chemical weathering (dissolution) erodes the rock, creating tunnels, caves, and other landforms. Karst Landforms: ○ Sinkholes: Depressions formed when the roof of a cave collapses or when surface material dissolves away. ○ Cockpit Karst: A landscape characterized by steep-sided, circular depressions (sinkholes) surrounded by ridges. ○ Tower Karst: Isolated, steep-sided towers of rock, typically seen in tropical areas with heavy rainfall and high dissolution rates. Caves and Caverns: ○ Formed by the dissolution of limestone, caves and caverns are often filled with stalactites (hanging from the ceiling) and stalagmites (growing up from the floor). These are formed by the deposition of minerals from dripping water. ○ The formation of these features is gradual, taking thousands or millions of years. Mass Movement Processes Mass movement refers to the downslope movement of rock, soil, and debris due to gravity. Mass movements can range from slow, imperceptible flows to rapid, catastrophic slides. Mass-Movement Mechanics: ○ The driving force for mass movement is gravity, while friction, cohesion, and water act as resistive forces. When the driving forces exceed resistive forces, mass movement occurs. Classes of Mass Movements: ○ Creeps: Slow, gradual movement of soil and rock. This often occurs when water content increases, reducing friction. ○ Slides: Rock Slides: A block of rock slides along a steep surface. Debris Slides: Larger, faster-moving slides that involve a mixture of soil, rocks, and other debris. ○ Flows: Mudflows: A rapid flow of water-saturated soil and debris. Debris Flows: Similar to mudflows but include larger rocks and boulders. ○ Falls: Rockfalls: The free-fall of rocks from a steep cliff, often triggered by weathering, earthquakes, or other disturbances. Human-Induced Mass Movement: ○ Activities like mining, construction, and deforestation can trigger mass movements. For example, mountaintop removal in mining leads to destabilization of slopes, causing landslides and mudflows in the affected regions. Examples of Mass Movements and Their Effects Talus Slopes: ○ Steep slopes often accumulate rock fragments at their base, known as talus. These are formed by the repeated action of frost wedging and other weathering processes. Landslides: ○ Large-scale mass movements, such as the Loma Prieta Earthquake Landslide in California, show the catastrophic effects of seismic events on landscapes, often involving debris flow and large-scale destruction. Debris Flow in Macedonia: ○ This type of mass movement, driven by heavy rainfall, is characterized by fast-moving slurry of rock, soil, and water, which can devastate local infrastructure. Mudflow in Southern California: ○ Often triggered by rainstorms after wildfires, these flows can rapidly carry large amounts of mud, trees, and debris down hillsides, threatening homes and roads. ____________________________________________________________________________ Chapter 14: River Systems Introduction to River Systems Rivers are key components in the Earth's hydrological system. They carry water from higher elevations to lower ones, eventually reaching the ocean or large lakes. River systems shape landscapes by eroding, transporting, and depositing sediments. The study of river systems involves understanding the processes of river flow, sediment transport, and the development of river features like deltas and floodplains. River Systems and Hydrological Cycle Rivers are part of the hydrological cycle, which describes how water moves through the Earth's atmosphere, surface, and subsurface. Water from precipitation either infiltrates the ground, runs off into rivers, or evaporates. River Basins and Watersheds: A river basin is the area drained by a river and its tributaries. The watershed is the specific area of land that contributes to a particular river or stream's flow. Watersheds are separated by drainage divides, which are typically ridges or higher land areas. River Characteristics Discharge: The amount of water flowing through a river at any given time. It is measured in cubic meters per second (m³/s). Discharge increases downstream as tributaries join the main river. Channel: The path through which the river flows. Channels vary in size and shape, from narrow mountain streams to wide, meandering lowland rivers. Gradient: The slope or steepness of the riverbed. Steeper gradients result in faster-moving water, while gentle gradients slow the flow. River Erosion and Transport Erosion: Rivers erode their channels through processes like abrasion (grinding of rocks by sediment), hydraulic action (the force of water against rocks), and solution (dissolution of soluble materials). Sediment Transport: Rivers carry sediments in three ways: ○ Dissolved load: Ions carried in solution from chemical weathering. ○ Suspended load: Particles carried by the water, such as silt and clay, that remain suspended. ○ Bed load: Larger particles like sand, gravel, and rocks that roll or slide along the riverbed. River Landforms As rivers move water and sediment, they create various landforms. These include features shaped by erosion, deposition, and flooding: V-shaped Valleys: Steep, narrow valleys formed by the erosive action of rivers, especially in mountainous regions. The shape of the valley reflects the ongoing downward erosion of the river. Floodplains: Flat, low-lying areas adjacent to rivers, formed by the deposition of sediments during floods. Floodplains are fertile areas often used for agriculture. Meanders: Curves or bends in a river's course that develop as the river erodes its banks and deposits sediments on the inside bends. Over time, meanders can grow, and river channels can shift. Oxbow Lakes: Formed when a meander is cut off, leaving a crescent-shaped body of water that no longer connects to the river. Alluvial Fans: Conical deposits of sediment formed where a river flows out of a mountain range and spreads out onto a flatter plain. These are often seen in arid regions. Deltas Deltas form when rivers flow into standing bodies of water, like seas or lakes. The river loses its speed and deposits sediments that have been carried downstream. The shape and characteristics of deltas depend on the balance between sediment deposition and water flow. Arcuate Delta: A fan-shaped delta with a curved, crescent-shaped outline. An example is the Nile River Delta. Estuarine Delta: A delta formed in areas where the river enters an estuary, where tides and currents influence sediment deposition. These deltas often have a more irregular shape. Bird Foot Delta: A delta with distinct "fingers" or "bird's feet," formed by the river splitting into many smaller channels as it enters the water. An example is the Mississippi River Delta. The Nile River Delta The Nile River Delta is one of the most famous and fertile deltas in the world. It forms in the Mediterranean Sea and is characterized by a fan-shaped distribution of sediment. The delta has been a key factor in the development of civilizations in Egypt. Human activity has altered the delta significantly, such as damming the Nile with the Aswan High Dam. The Mississippi River Delta The Mississippi River Delta is another iconic delta, formed by the river flowing into the Gulf of Mexico. Over time, the river has built up a vast region of sediment, creating a diverse ecosystem. The delta has been heavily influenced by human activity, including the construction of levees and other flood control measures to prevent flooding and protect cities like New Orleans. Levees and Flooding Levees are natural or artificial embankments that control river flooding by confining the river’s flow to its channel. They are often built along rivers to protect nearby human settlements from floods. Natural Levees: Formed by the deposition of sediment during floods, creating embankments along the river's edge. Artificial Levees: Human-made embankments that are built to raise the banks of the river. These levees increase the risk of catastrophic flooding if they fail. Flooding and River Management Flooding occurs when a river's discharge exceeds its channel capacity, often due to heavy rainfall or snowmelt. While floods can be devastating, they also play a vital role in replenishing nutrients in floodplains. Flood Control: Various measures like dams, levees, and floodwalls are implemented to control and prevent flooding. However, these structures can have significant environmental impacts, such as disrupting ecosystems and altering sediment transport. Human Impact on River Systems Human activity has significantly altered many river systems. Dams, urbanization, and agricultural practices have all affected the natural processes of rivers. For example, damming rivers to create reservoirs can prevent sediment deposition downstream, affecting ecosystems and deltas. Chapter 16: Oceans and Coastal Systems Introduction to Oceans and Coastal Systems The ocean covers more than 70% of Earth's surface, influencing weather patterns, ecosystems, and human activities. Coastal systems are the dynamic boundaries between land and sea, where interactions between tides, waves, and currents shape the environment. This chapter explores the chemical composition and physical structure of the ocean, coastal processes, and their impacts on coastal landforms and ecosystems. Global Oceans and Seas Properties of Seawater: Seawater is primarily a solution of water and dissolved solids (salts) with various gases (oxygen, carbon dioxide, nitrogen) dissolved in it. ○ Salinity: Salinity refers to the concentration of dissolved salts in seawater. The average global salinity is 3.5%, or 35‰ (parts per thousand). Variations in salinity occur depending on location (e.g., near freshwater inputs, like rivers, salinity is lower). ○ Brackish Water: Water with salinity lower than 3.5%, typically found in areas where freshwater runoff dilutes seawater, such as in estuaries. ○ Brine: Seawater with salinity higher than 3.5%, found in areas of high evaporation, like the Persian Gulf or Red Sea, where salinity can reach up to 22.5%. Oceanic Acidification: Oceans absorb CO2 from the atmosphere, which forms carbonic acid in seawater, lowering the ocean's pH. This change in pH (currently around 8.1) is expected to decrease further, affecting marine life and ecosystems. Vertical Zonation of the Ocean The ocean's structure is divided into vertical zones based on light penetration, temperature, and salinity: Epipelagic Zone: The uppermost layer, where sunlight supports photosynthesis, typically extending from the surface to around 200 meters. Mesopelagic Zone: The twilight zone, where light is faint and most organisms rely on bioluminescence. Bathypelagic and Abyssopelagic Zones: The deeper, darker regions with no natural light, inhabited by unique organisms adapted to extreme conditions. Coastal System Components Coastal Environment: Coastal systems involve the interaction of land, sea, and atmosphere. Inputs include solar energy, atmospheric winds, climate, and human activity. ○ Littoral Zone: The coastal zone affected by tidal actions, extending from the high tide line to the low tide line. ○ Sea Level: Fluctuations in sea level are influenced by climatic cycles, such as ice ages, where sea levels were lower during colder periods and higher during warmer periods. Human activities, such as glacial melting, also affect sea levels. Tides Tidal Movements: Tides are the periodic rise and fall of sea levels caused by the gravitational pull of the Moon and the Sun. Tides occur roughly every 12 hours and 25 minutes due to the moon's orbit around Earth. ○ Spring Tides: Occur when the Sun and Moon align during full and new moons, causing the highest tidal range. ○ Neap Tides: Occur when the Sun and Moon are at right angles during the first and third quarter moons, resulting in the smallest tidal range. ○ Other Factors Influencing Tides: Ocean basin size, depth, shoreline shape, and latitude can influence tidal range and behavior. For example, the highest tides occur in partially enclosed bays. Waves Wave Formation: Waves are created by wind friction on the ocean's surface. Their size and energy depend on wind speed, duration, and fetch (the distance over which the wind blows). ○ Wave Refraction: The bending of waves as they approach the shore, which can straighten irregular coastlines. ○ Longshore Currents and Beach Drift: Waves hitting the coast at an angle create longshore currents, which move sand along the beach, contributing to coastal erosion and deposition. Tsunamis: Large seismic waves caused by sudden disturbances in the seafloor, such as earthquakes, landslides, or volcanic eruptions. Tsunamis travel at high speeds across the ocean and can increase dramatically in height when reaching shallow coastal waters. Coastal Processes and Landforms Coastal systems are shaped by both erosional and depositional processes: Erosional Coasts: Found in areas with high relief and tectonic activity, such as the Pacific coast of North America, where coastlines are shaped by wave action and coastal cliffs. ○ Landforms: Includes features like sea stacks, arches, and cliffs created by the erosion of rock. Depositional Coasts: Found along regions with gentle slopes and tectonic stability, such as the Atlantic and Gulf coasts of the U.S. These areas experience more sediment deposition, creating beaches, spits, and coastal dunes. Barrier Islands and Beaches Barrier Beaches and Islands: Long, narrow islands made of sand that form parallel to the coast, protecting inland areas from wave action. ○ Hazards: Barrier islands are dynamic and can change shape or migrate due to storms and rising sea levels. They are vulnerable to erosion and flooding, posing risks to human settlements. ○ Barrier Island Development: Guidelines for development on barrier islands focus on reducing risk from storms and ensuring environmental protection. Coral Reefs Coral Ecosystems: Coral reefs are biological formations made from the calcium carbonate skeletons of corals, which are marine animals living in a symbiotic relationship with algae. The algae provide energy through photosynthesis, and the corals provide protection and nutrients. ○ Coral Bleaching: When corals experience thermal stress from high water temperatures, they expel the algae, causing the coral to turn white and weakening the ecosystem. ○ Distribution: Coral reefs are mostly found in tropical and subtropical regions between 30°N and 30°S, with the highest concentrations near the equator. Coastal Wetlands Wetlands Types: Coastal wetlands, such as salt marshes and mangrove swamps, are vital ecosystems that support diverse wildlife. Salt marshes are typically found in temperate regions, while mangroves thrive in tropical zones. ○ Functions: Wetlands serve as wildlife habitats, flood buffers, water filters, and carbon sinks. They play a critical role in mitigating climate change and protecting coastal areas from storm surges. Human Impacts on Coastal Ecosystems Human activities such as development, pollution (including oil spills), and climate change have significant impacts on coastal systems: Oil Spills: These events can cause severe damage to coastal ecosystems, particularly coral reefs and wetlands, by contaminating water and disrupting habitats. ○ Protection and Conservation: Efforts to protect coastal systems focus on sustainable development, pollution control, and the restoration of damaged ecosystems. Chapter 17: Glacial Landscapes and the Cryosphere Learning Objectives: 1. Explain how snow transforms into glacial ice. 2. Differentiate between alpine glaciers and continental ice sheets. 3. Understand how glaciers move. 4. Identify and explain the erosional and depositional landforms that glaciers create. 5. Discuss the distribution of permafrost and periglacial processes. 6. Examine the landscapes of the Pleistocene Epoch and the ongoing changes in polar regions today. Chapter Outline: 1. Snow into Ice: The Basis of Glaciers ○ Properties of snow ○ Snow avalanches ○ Formation of glacial ice ○ Types of glaciers: Alpine glaciers, continental ice sheets 2. Glacial Processes ○ Glacial mass balance ○ Glacial movement ○ Erosional and depositional landforms created by glaciers 3. Periglacial Landscapes ○ Permafrost and its distribution ○ Periglacial processes 4. The Pleistocene Epoch ○ Pleistocene landscapes ○ Paleolakes 5. Arctic and Antarctic Regions ○ Recent polar region changes due to climate change Key Concepts in Detail: Glaciers: Glaciers are massive ice bodies that exist on land or as floating ice shelves. They are different from frozen lakes or groundwater ice because glaciers move slowly and flow like rivers of ice. About 77% of Earth’s freshwater is frozen in glaciers, and their movement plays a vital role in shaping the Earth's surface. Types of Glaciers: 1. Alpine Glaciers: ○ These glaciers form in mountainous regions and flow through valleys. Common in places like the Alps, Rockies, and Himalayas. ○ Subtypes of alpine glaciers: Cirque glaciers: Small glaciers confined to bowl-shaped depressions at the head of valleys. Valley glaciers: Large glaciers that flow through steep-walled valleys, often leading to the formation of U-shaped valleys. Piedmont glaciers: These form at the base of mountain ranges where valley glaciers spread out and meet flat land. Tidewater glaciers: Glaciers that flow into the sea and calve off, creating icebergs, especially in coastal regions. ○ Glacial Calving: The process by which large chunks of ice break off from glaciers, forming icebergs. 2. Continental Glaciers: ○ These glaciers are much larger and cover vast areas, such as the Greenland Ice Sheet and the Antarctic Ice Sheet. ○ Types of continental glaciers: Ice sheets: Cover more than 50,000 km² of land (e.g., Antarctica and Greenland). Ice caps: Smaller than ice sheets but still large, covering less than 50,000 km². These are roughly circular. Ice fields: Elongated and often found in mountain regions, covering smaller areas than ice caps. Formation of Glacial Ice: Snow accumulates during winter. In the following years, if the snow survives summer melt, it compresses under its own weight and transforms into firn (an intermediate stage between snow and glacial ice). Over time, firn compresses further, undergoes metamorphism, and becomes dense glacial ice. The transformation from snow to ice can take hundreds to thousands of years. In Antarctica, due to dry conditions and extremely low temperatures, this process may take as long as 1,000 years. Glacial Movement: Glaciers move in stream-like patterns, though much more slowly. Their movement is driven by the weight of the ice, gravity, and meltwater at the base that lubricates movement. Two primary modes of glacial movement: ○ Internal Deformation: Ice crystals within the glacier change shape and flow, like a plastic material. ○ Basal Sliding: Ice moves over its bed due to meltwater acting as a lubricant. Glaciers can advance (move forward) or retreat (move backward), leaving behind erosional features such as striae(scratches in rock surfaces) caused by rocks embedded in the glacier. Roche Moutonnée: A rock feature shaped by glacial erosion, with one side smoothed by ice flow and the other side steepened by abrasion. Erosional and Depositional Landforms: 1. Erosional Landforms: ○ Cirques: Bowl-shaped hollows at the head of a glacier, formed by erosion. ○ U-shaped valleys: Formed when glaciers erode a previously V-shaped river valley, leaving a broad, flat valley floor. ○ Fjords: U-shaped valleys that are submerged by rising sea levels after glaciers retreat. 2. Depositional Landforms: ○ Moraines: Deposits of rock and sediment left by glaciers. Lateral moraines: Form at the sides of glaciers. Medial moraines: Created when two glaciers converge, merging their lateral moraines. Terminal moraines: Mark the furthest advance of a glacier, where it has left a large pile of debris. Ground moraines (till plains): Formed when debris is spread across the landscape by glaciers. ○ Drumlins: Smooth, elongated hills of sediment formed under glaciers, aligned in the direction of ice flow. ○ Eskers: Long, winding ridges of sediment deposited by meltwater streams beneath glaciers. ○ Kettle lakes: Formed when blocks of ice left by glaciers melt and create depressions filled with water. Periglacial Landscapes: Periglacial regions are those that exist near glaciers or in cold climates where glaciers no longer exist but still experience the effects of freezing temperatures. Permafrost is a layer of ground that remains frozen for two or more consecutive years. It is found in regions like the tundra and high-altitude areas. ○ Permafrost distribution: Covers about 20% of Earth's land, with 80% of Alaska experiencing permafrost. ○ Continuous permafrost: Found in the coldest areas, where permafrost is present throughout the year. ○ Discontinuous permafrost: Occurs in areas where the ground is frozen but thawing occurs in some parts of the year. Periglacial Processes: ○ Frost heaving: The upward movement of soil and rocks caused by the freezing and thawing of water in the ground. ○ Frost thrusting: The horizontal displacement of rocks and soil due to freezing conditions. ○ Ice wedges: Cracks that develop in the permafrost when liquid water enters and freezes, expanding the cracks. ○ Patterned ground: Geometric patterns of stones and soil, formed by frost action in permafrost regions. Pleistocene Epoch: During the Pleistocene Epoch, Earth underwent several ice ages, significantly shaping global landscapes. Pleistocene glaciations covered much of the northern hemisphere, causing changes in sea levels and the creation of paleolakes (ancient lakes formed by glacial meltwater). Recent Polar Region Changes: Climate change is rapidly affecting polar regions: ○ Darkening of ice sheet surfaces: As the ice melts, darker areas are exposed, absorbing more heat and accelerating the melting process. ○ Increase in melt ponds and supraglacial lakes: These water bodies form on top of glaciers and accelerate ice loss by increasing the absorption of solar energy. ○ Ice shelf breakup: Large chunks of ice that were once connected to glaciers have broken off, contributing to rising sea levels. Key Figures and Visuals: Malaspina Glacier (Alaska) and Midgard Glacier (Greenland) are shown to illustrate the size and flow of glaciers. Permafrost maps: These figures show the global distribution of permafrost. Glacial features: Diagrams of moraines, drumlins, and eskers highlight the landscape modifications caused by glacial activity. Chapter 4: Atmospheric Energy and Global Temperatures Learning Objectives: 1. Alternative pathways for solar energy as it passes through the troposphere and the concept of albedo (reflectivity). 2. Four types of heat transfer: radiation, conduction, convection, and advection. 3. The effects of greenhouse gases, clouds, and aerosols on atmospheric heating and cooling. 4. Review of the Earth–atmosphere energy balance and patterns of net radiation at the surface. 5. Define the concept of temperature and the principal temperature controls that produce global temperature patterns. 6. Interpret global temperature patterns from January and July temperature maps and from annual temperature range maps. 7. Discuss heat waves and human responses to temperature extremes. 8. Describe urban heat island conditions and the impacts of human activities on global temperatures. Chapter Outline: 1. Energy-Balance Essentials ○ Solar Radiation: Pathways and Principles ○ Heat Transfer at Earth’s Surface 2. Energy Balance in the Troposphere ○ Outgoing Radiation and the Greenhouse Effect ○ Earth–Atmosphere Energy Balance 3. Energy Balance at Earth’s Surface ○ Daily Radiation Patterns ○ A Simplified Surface Energy Budget 4. Temperature Concepts and Measurement ○ Temperature Scales and Measuring Temperature 5. Principal Temperature Controls ○ Latitude, Altitude, Cloud Cover, Land–Water Heating Differences 6. Earth’s Temperature Patterns ○ Global January and July Temperature Comparison ○ Annual Temperature Range and Polar Region Temperatures 7. Cold Snaps and Heat Waves 8. Human Impacts on Earth’s Energy Balance ○ Global Temperature Increase and Urban Environments Key Concepts in Detail: Solar Radiation: Pathways and Principles 1. Transmission: Refers to energy passing through the atmosphere or water without being absorbed or altered. 2. Absorption: The assimilation of radiation by matter (such as CO2 or water vapor), converting it into heat energy. ○ CO2 and water vapor are critical in absorbing both solar and longwave radiation, contributing to the greenhouse effect. 3. Scattering: The redirection of light without changing its wavelengths. This scattering causes diffuse radiation(light that reaches the surface indirectly). 4. Refraction: Changes the direction and speed of light as it passes through different mediums (such as the atmosphere). 5. Reflection: The portion of radiation that bounces off surfaces without being absorbed. The concept of albedo(reflectivity) refers to how much radiation is reflected back into space. High albedo surfaces (e.g., ice or snow) reflect more sunlight, while dark surfaces (e.g., oceans) absorb more. 6. Albedo: Defined as the ratio of reflected solar radiation to incoming solar radiation. For example, snow has a high albedo (reflecting about 80-90% of sunlight), while the ocean has a low albedo (reflecting only 10-20%). Key Figures: Annual Mean Insolation: Shows the average solar energy received at Earth’s surface, with higher values at the equator and lower values at the poles. Reflection and Absorption Diagrams: These demonstrate how different surfaces, such as forests, oceans, or snow, interact with incoming solar radiation. Heat Transfer at Earth’s Surface Radiation: Energy traveling through space or air, such as sunlight. Conduction: Molecule-to-molecule transfer of heat energy, typically from warmer to cooler areas. Occurs in solids, liquids, and gases. Convection: Vertical movement of energy due to heat transfer. Warm air rises, and cooler air sinks, creating convection currents. Advection: Horizontal movement of heat through the atmosphere, primarily by wind. Latent Heat: Heat absorbed or released when water changes state, such as from liquid to gas (evaporation) or from gas to liquid (condensation). Key Figures: Heat Transfer Diagrams: Visualizations of how radiation, conduction, convection, and advection occur in the atmosphere and on Earth's surface. The Greenhouse Effect and Energy Budget 1. Greenhouse Analogy: ○ The atmosphere absorbs heat energy from Earth’s surface and delays its transfer into space, similar to how a greenhouse traps heat. ○ The greenhouse gases (CO2, water vapor, methane) absorb longwave radiation, warming the atmosphere and surface. 2. Clouds and Aerosols: ○ Low, thick clouds cool the surface by reflecting sunlight. ○ High-altitude ice clouds allow sunlight to pass through but trap heat, warming the surface. ○ Aerosols (small particles like dust and pollutants) reflect sunlight, contributing to cooling effects. Greenhouse Gas Impact: The greenhouse effect traps heat in the atmosphere, keeping Earth warmer than it would be otherwise. However, increased concentrations of greenhouse gases are leading to global warming. Key Figures: Cloud-Albedo Forcing: A diagram showing the contrasting effects of low and high clouds on Earth’s energy budget. Energy Balance at Earth’s Surface 1. Net Radiation: ○ The difference between incoming solar radiation and outgoing radiation. This balance is crucial for determining the energy available for heating Earth's surface and atmosphere. 2. Latent Heat of Evaporation: ○ Energy involved in the change of state of water (e.g., from liquid to vapor). It plays a key role in regulating surface temperatures, particularly in humid regions. 3. Sensible Heat: ○ Heat that can be measured with a thermometer and is responsible for the temperature of the air. 4. Ground Heating and Cooling: ○ The process by which heat is absorbed or released by the Earth's surface, influencing temperature changes over time. Temperature Concepts and Measurement Heat vs. Temperature: ○ Heat is the total energy of molecular motion, while temperature measures the average kinetic energy of molecules. ○ Temperature changes result from the absorption or emission of heat energy. Temperature Scales: ○ Fahrenheit (°F): Used primarily in the U.S. (freezing point: 32°F, boiling point: 212°F). ○ Celsius (°C): Used internationally (freezing point: 0°C, boiling point: 100°C). ○ Kelvin (K): Absolute temperature scale, starting at absolute zero (-273.15°C). 0 K represents no molecular motion. Key Figures: Thermometers and Instrument Shelters: Visuals showing how temperature is measured and how instruments are protected from external influences like direct sunlight. Principal Temperature Controls 1. Latitude: ○ Areas near the equator (low latitudes) experience higher average temperatures and smaller temperature ranges. Areas near the poles (high latitudes) experience lower average temperatures and larger daily and seasonal temperature ranges. 2. Altitude/Elevation: ○ Temperature decreases with altitude. Higher elevations tend to have cooler temperatures due to thinner air and lower ability to absorb heat. 3. Cloud Cover: ○ Clouds increase albedo and moderate temperatures by cooling days and warming nights. 4. Land–Water Heating Differences: ○ Water heats and cools more slowly than land due to its higher specific heat and evaporation rate. ○ Land heats up faster during the day and cools quicker at night compared to water. Key Figures: Latitude and Temperature: Diagrams showing temperature differences between high and low latitudes. Earth’s Temperature Patterns 1. January vs. July Temperature Maps: ○ In January, the thermal equator (line of highest temperatures) shifts southward, and in July, it moves northward. ○ The difference in temperature distribution between the two months is more pronounced over large landmasses. 2. Annual Temperature Range: ○ Continentality: Areas farther from the ocean (continental) experience a greater variation in temperature, both daily and annually, than coastal areas (marine effect). Key Figures: Temperature Maps: Global maps showing January, July, and annual temperature patterns, as well as polar region temperatures. Human Impacts on Earth’s Energy Balance 1. Global Temperature Increase: ○ Human activities, particularly the burning of fossil fuels, are increasing greenhouse gas concentrations, leading to a rise in global temperatures. 2. Urban Heat Island (UHI): ○ Urban environments are often much warmer than rural areas due to human activities, increased surface areas (concrete, asphalt), and reduced vegetation. Key Figures: Global Temperature Increase: A chart showing the rise in global average temperatures over time. Urban Heat Island Effect: A diagram showing temperature differences between urban and rural areas. Air Temperature and the Human Body Wind Chill: The combined effect of temperature and wind speed, indicating the perceived temperature on exposed skin. Heat Index: The apparent temperature caused by the combination of air temperature and humidity. Key Figures: Wind Chill Table: A chart showing wind chill values for various temperatures and wind speeds. Heat Index Table: A chart showing the heat index for various temperatures and humidity levels Chapter 5: Atmospheric and Oceanic Circulations Learning Objectives: 1. Define wind and air pressure and describe the instruments used to measure them. ○ Wind refers to the horizontal movement of air, primarily caused by differences in air pressure. Wind is typically described by two properties: wind speed (how fast the air is moving) and wind direction (where the air is coming from). ○ Winds are named based on the direction they originate from. For example, a north wind blows from the north toward the south. ○ Air pressure is the force per unit area exerted by the weight of the air above a given point. This force decreases as altitude increases due to the reduction in the weight of the air above. ○ Instruments used to measure these variables include: Anemometer: Measures wind speed by counting the number of rotations of cups or vanes, typically in meters per second (m/s) or kilometers per hour (km/h). Wind vane (weather vane): Measures the wind's direction by indicating the direction from which the wind is blowing. Barometer: Measures atmospheric pressure. The two types of barometers are: Mercury barometer: A tube filled with mercury, where the height of mercury in the tube corresponds to air pressure. Aneroid barometer: A metal box with flexible sides that contracts or expands based on changes in air pressure. 2. Explain the driving forces within the atmosphere—gravity, pressure gradient force, Coriolis force, and friction force—that affect wind. ○ Gravity is the force that keeps the atmosphere in place, ensuring that air does not escape into space. It creates the weight of the air and contributes to the formation of atmospheric pressure. ○ Pressure gradient force (PGF) refers to the difference in pressure between high and low-pressure areas. Air flows from regions of high pressure to regions of low pressure, creating wind. The steeper the pressure gradient, the stronger the wind. ○ Coriolis force is caused by the Earth's rotation. As the Earth spins, moving objects (like air) are deflected. In the Northern Hemisphere, moving air is deflected to the right, while in the Southern Hemisphere, it is deflected to the left. This force causes winds to move in curved paths rather than straight lines. The strength of the Coriolis effect increases with the speed of the wind and is strongest at the polesand weakest at the equator. ○ Friction force slows down wind speed, especially near the Earth's surface. It arises from the interaction of wind with Earth's surface, including land, water, and vegetation. It causes surface winds to move at an angle to the pressure gradient rather than directly from high to low pressure. 3. Locate primary high- and low-pressure areas and the principal winds on Earth. ○ The Earth's primary pressure systems are broadly determined by the latitudes and the Coriolis effect. These pressure systems influence global wind patterns. Equatorial Low-Pressure Trough (ITCZ): Found near the equator, this low-pressure zone is characterized by rising warm air. As air rises, it cools and condenses, forming clouds and precipitation. This zone is associated with the Intertropical Convergence Zone (ITCZ), where trade winds converge and cause frequent thunderstorms. Subtropical High-Pressure Cells: Located around 30° N and S latitude (e.g., the Bermuda High in the Atlantic and the Pacific High in the Pacific). These regions are characterized by descending, dry air that creates clear skies and deserts. Subpolar Low-Pressure Cells: Located around 60° N and S latitude, where the polar front lies. These regions are cool and moist, associated with rising air and frequent precipitation. Polar High-Pressure Cells: Found at the poles, these regions are frigid and dry, with air descending from higher altitudes. The polar easterlies (winds from east to west) are characteristic here. 4. Describe upper-air circulation and define the jet streams. ○ Upper-air circulation refers to the movement of air high in the atmosphere, particularly the air flow that is not affected by surface friction. This circulation significantly influences weather patterns by directing storm systems and the distribution of heat. ○ Jet streams are fast-moving narrow bands of air located in the upper atmosphere (typically at altitudes between 9 to 16 km). There are two main jet streams: Polar jet stream: Found around 60° latitude, this jet stream is strong and cold, often steering weather systems and influencing storm tracks. Subtropical jet stream: Located around 30° latitude, it is typically weaker and warmer than the polar jet. It plays a role in controlling the movement of tropical and subtropical systems. 5. Explain several types of local and regional winds, including the monsoons. ○ Local Winds are winds that occur on a small scale due to local temperature and pressure differences. Examples include: Land and Sea Breezes: These are diurnal winds (occuring daily) caused by differences in the heating and cooling rates of land and water. During the day, land heats up faster than the sea, causing air to rise over the land and draw in cooler air from the sea. At night, the reverse happens: land cools faster than the sea, causing air to flow from the land to the sea. Mountain and Valley Breezes: During the day, mountains heat up more quickly than valleys, causing warm air to rise up the slopes (valley breeze). At night, the air in the valleys cools more rapidly, causing air to flow down into the valleys (mountain breeze). Monsoonal Winds: Seasonal winds that change direction depending on the time of year, bringing wet conditions during certain months and dry conditions during others. They are most famous in the Indian subcontinent, where the wet monsoon occurs from the southwest in summer, while the dry monsoon blows from the northeast in winter. 6. Sketch the basic pattern of Earth’s major surface ocean currents and deep thermohaline circulation. ○ Surface Currents: These are primarily driven by surface winds, the Coriolis force, and the interaction of winds with ocean water. These currents form large-scale circular patterns known as gyres. In the Northern Hemisphere, gyres flow clockwise (e.g., Gulf Stream), while in the Southern Hemisphere, they flow counterclockwise (e.g., Antarctic Circumpolar Current). ○ Thermohaline Circulation (Deep Currents): These currents are driven by differences in water temperature and salinity, which affect water density. Cold, salty water sinks in polar regions, driving the deep ocean currents. This circulation is a critical component of the Earth's climate system as it helps regulate the distribution of heat around the planet. 7. Summarize the El Niño and La Niña oscillations of air pressure and circulation in the Pacific Ocean. ○ El Niño is a climatic event that occurs when the trade winds in the Pacific Ocean weaken, leading to a warming of sea surface temperatures in the central and eastern Pacific. This disrupts typical weather patterns, causing: Warmer-than-normal conditions in the Pacific, especially near the equator. Altered weather patterns globally, such as droughts in Southeast Asia and heavy rains in the southern United States. ○ La Niña is the opposite phase, characterized by stronger-than-normal trade winds and cooler-than-normal sea surface temperatures in the central Pacific. La Niña typically leads to: Cooler and drier conditions in the eastern Pacific. An increase in hurricanes in the Atlantic Ocean due to stronger winds. Chapter Outline Expanded: 1. Wind Essentials ○ Wind Measurement Instruments: Anemometer, Wind Vane, Barometer ○ Atmospheric Pressure and Wind Definition, units, and measurement methods Relationship between pressure and wind 2. Driving Forces Within the Atmosphere ○ Pressure Gradient Force (PGF) Mechanisms of air movement Effect of isobar spacing on wind speed ○ Coriolis Force Impact of Earth's rotation on wind direction Variations by hemisphere and latitude ○ Friction Force Interaction with surface features and impact on wind ○ Summary of Physical Forces on Winds Combined effects of PGF, Coriolis, and friction 3. Atmospheric Patterns of Motion ○ Primary Pressure Areas and Associated Winds ITCZ, Subtropical Highs, Subpolar Lows, Polar Highs ○ Upper Atmospheric Circulation Jet Streams, Rossby Waves, and their influence on weather ○ Local and Regional Winds Diurnal and seasonal variations in wind direction and speed ○ Monsoonal Winds Seasonal reversal of winds and precipitation patterns 4. Oceanic Currents ○ Surface Currents and their global distribution ○ Thermohaline Circulation and its role in global heat distribution 5. Natural Oscillations in Global Circulation ○ El Niño-Southern Oscillation (ENSO) and its impacts on weather ○ Pacific Decadal Oscillation (PDO) and long-term climate patterns ○ Arctic and North Atlantic Oscillations and their effects on Northern Hemisphere weather Key Concepts and In-Depth Details: Wind Essentials Wind is generated by pressure differences and modified by various forces as it moves across Earth's surface. These movements shape weather systems and are crucial for climate regulation. Driving Forces and Wind Mechanics The balance between the pressure gradient force, Coriolis force, and friction dictates wind patterns. Their interactions lead to complex, dynamic weather systems. Pressure and Wind Patterns Pressure systems dictate the general circulation of winds, which are altered by latitude, seasonal changes, and local geographical factors (e.g., mountains, oceans). Oceanic Currents and Thermohaline Circulation Surface currents, driven by wind, and deep ocean currents, driven by density differences, help regulate Earth's climate by distributing heat and nutrients. Chapter 7: Weather Learning Objectives: 1. Define the basic lifting mechanisms that lead to cloud formation and precipitation. 2. Describe the structure of different types of storms, including thunderstorms, tornadoes, and tropical cyclones. 3. Explain how cyclonic systems develop, particularly midlatitude cyclones and tropical cyclones. 4. Identify and understand the mechanisms of frontal lifting and the effects of different types of fronts. Key Concepts: Lifting Mechanisms for Cloud Formation Lifting refers to the process by which air is forced to rise, cool, and condense, forming clouds and potentially precipitation. This process is essential for weather formation and can occur through different mechanisms: 1. Convergent Lifting ○ Mechanism: Air flows toward an area of low pressure. When air converges (flows inward), it is forced to rise, leading to cooling and condensation. ○ Characteristics: Occurs around low-pressure systems, where the converging air masses are typically warmer and less dense. This process is fundamental in the formation of cyclonic systems. 2. Example: Tropical cyclones, where warm air converges toward the center and rises, creating cloud formation and precipitation. 3. Convectional Lifting ○ Mechanism: Air above warmer surfaces is heated by direct contact with the ground and becomes buoyant. This air rises due to its lower density. ○ Characteristics: Common in areas with significant surface heating, such as over land during the day, where the surface warms more quickly than the surrounding air, causing localized convection. 4. Example: Afternoon thunderstorms, which form as the surface heats and causes warm air to rise rapidly. 5. Orographic Lifting ○ Mechanism: Occurs when air is forced to ascend over a mountain range. As the air rises, it cools and moisture condenses, potentially leading to precipitation. ○ Characteristics: The windward side of the mountain range experiences rising air and often rainfall, while the leeward side (rain shadow) sees drier conditions due to descending air. 6. Example: The Pacific Northwest of the U.S., where moist air from the ocean is forced up by the Cascade Range, creating rain on the west side and dry conditions in eastern Washington and Oregon (rain shadow effect). 7. Frontal Lifting ○ Mechanism: A front is the boundary between two air masses with differing temperature, humidity, and density. The less dense, warmer air is forced to rise over the denser, colder air. ○ Types of Fronts: Cold Front: Cold air forces warm air aloft. These are often associated with rapid, intense weather changes. Warm Front: Warm air moves up and over colder air. These fronts tend to produce more gradual changes in weather, with extended periods of precipitation ahead of the front. ○ Frontal Lifting and Precipitation: Cold Front: As cold air pushes under the warm air, it forces it upward, causing condensation and precipitation. Typically results in brief, intense weather events, like thunderstorms. Warm Front: Warm air gradually rises over cooler air, causing slow, steady precipitation, often in the form of light rain or snow ahead of the front. Cyclonic Systems Cyclonic systems are large-scale weather systems driven by low-pressure centers where air converges and rises. These systems can cause significant weather patterns, including stormy conditions, precipitation, and severe weather events. 1. Midlatitude Cyclone ○ Structure: A midlatitude cyclone is a large-scale weather system that forms along the polar front, between the warm tropical air and cold polar air. It has a well-defined low-pressure center with cold and warm fronts associated with it. ○ Air Mass Model: The cyclone consists of distinct air masses of differing temperatures and humidity levels. The boundary between these masses forms the fronts. ○ Conveyor Belt Model: In this model, the cyclone’s structure includes a warm conveyor belt of air that rises and an associated cold conveyor belt that descends, helping to transfer heat and moisture, contributing to cloud formation and precipitation. 2. Frontal Systems in Cyclones ○ Cold Fronts: As cold fronts move rapidly through a region, they cause intense, short-term precipitation, often leading to thunderstorms or even tornadoes. ○ Warm Fronts: These fronts move more slowly, and the warm air rises over the cold air, causing more prolonged and steady precipitation. Severe Weather Events This section covers the formation and characteristics of violent weather phenomena, such as ice storms, thunderstorms, tornadoes, derechos, and tropical cyclones. 1. Thunderstorms ○ Formation: Thunderstorms are initiated by convectional lifting, where warm, moist air rises, cools, and condenses to form clouds. The release of latent heat during condensation leads to further rising air and intensified convection. ○ Characteristics: Thunderstorms can produce intense precipitation, lightning, hail, and even tornadoes. ○ Development: The stages of thunderstorm development include: Cumulus Stage: The initial stage of convection where warm air rises. Mature Stage: The storm reaches its maximum intensity, with strong updrafts, precipitation, and possible lightning. Dissipation Stage: As the updrafts weaken and precipitation becomes lighter, the storm dies down. 2. Lightning ○ Mechanism: Lightning occurs when there is a build-up of electrical charges within a thunderstorm, usually between the cloud and the ground or within the cloud itself. ○ Types: Cloud-to-ground, cloud-to-cloud, and intra-cloud lightning. 3. Derechos ○ Characteristics: A derecho is a widespread, long-lived wind storm that is associated with a fast-moving band of severe thunderstorms. It can produce straight-line winds, sometimes exceeding 100 mph, and cause widespread damage similar to that of a tornado but over a larger area. 4. Tornadoes ○ Development: Tornadoes form from severe thunderstorms when conditions like wind shear (changes in wind direction and speed with altitude) and atmospheric instability create rotating columns of air. ○ Formation Process: The interaction between warm, moist air and cooler, drier air creates wind patterns conducive to the development of tornadoes. A rotating updraft, called a mesocyclone, can spawn tornadoes when it stretches downward. ○ Tornado Characteristics: Tornadoes are classified by the Fujita scale, ranging from F0 (weak) to F5 (extremely strong). The path of a tornado is typically narrow but can be several miles long. 5. Tropical Cyclones ○ Formation: Tropical cyclones form over warm ocean waters (typically >26°C) where they gain energy through the evaporation of warm water. The rising moist air creates a low-pressure center and starts to rotate due to the Coriolis effect. ○ Structure: Tropical cyclones have a well-defined eye (the calm center), surrounded by intense wind and precipitation in the eyewall. The system can extend outward for hundreds of miles. ○ Cyclone Tracks: These systems follow a predictable path depending on global wind patterns. The tracks of tropical cyclones typically move from east to west in tropical regions, then curve poleward. ○ Tropical Cyclone Naming: In the Atlantic and Eastern Pacific Oceans, storms are given names to avoid confusion when multiple storms occur simultaneously. Cyclone Formation Areas and Tracks 1. Primary Areas of Cyclone Formation: ○ Tropical cyclones (hurricanes or typhoons) form in tropical regions, particularly between latitudes 5° and 20° in both hemispheres. The warm ocean waters in these regions provide the necessary heat and moisture for storm development. 2. Cyclone Tracks: ○ Tropical cyclones follow specific tracks, influenced by prevailing winds and atmospheric pressure systems. In the Northern Hemisphere, they generally move westward and then curve poleward due to the Coriolis effect and prevailing westerly winds.