Untitled Document-9 PDF
Document Details
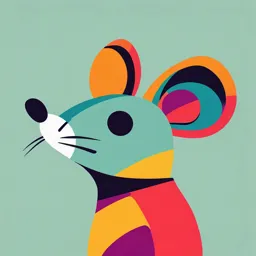
Uploaded by ProminentPorcupine4196
Tags
Summary
This document covers the basics of homeostasis, including temperature regulation and blood sugar levels. It also discusses the difference between negative and positive feedback loops, and the major types of bonds, including ionic and covalent, and how water is polar.
Full Transcript
What is homeostasis, and what is its role in the human body? Homeostasis is the process by which the body maintains a stable internal environment despite changes in the external environment. This includes regulating factors like temperature, pH, hydration, and electrolyte levels. Tempe...
What is homeostasis, and what is its role in the human body? Homeostasis is the process by which the body maintains a stable internal environment despite changes in the external environment. This includes regulating factors like temperature, pH, hydration, and electrolyte levels. Temperature Regulation: The body maintains an average temperature around 98.6°F (37°C). If it gets too hot or cold, mechanisms like sweating or shivering kick in to bring it back to normal. Blood Sugar Levels: Homeostasis helps regulate blood sugar through insulin and glucagon, ensuring our cells get the energy they need. Fluid Balance: The body controls water levels to prevent dehydration or overhydration, which can affect cellular function. What is the difference between negative and positive feedback loops? Understand examples of each Negative Feedback Loops-These loops work to counteract a change, helping to return the body to a set point. When a variable deviates from its normal range, negative feedback initiates processes to reverse that change. Example: Temperature Regulation When your body temperature rises (like during exercise), sensors detect this change. The hypothalamus in the brain triggers sweating and increased blood flow to the skin to cool you down. Once your temperature returns to normal, these processes slow down. Positive Feedback Loops-These loops amplify a change, moving the body away from its set point. They are less common and typically occur during specific processes that need to be completed quickly. Example: Childbirth During labor, the release of the hormone oxytocin increases contractions in the uterus. Stronger contractions push the baby down, which stimulates more oxytocin release. This loop continues until the baby is born, after which the process stops. What are the three major types of bonds discussed in class?/examples 1. Ionic Bonds Description: Formed when one atom transfers electrons to another, creating charged ions that attract each other. Example: Sodium chloride (table salt) is formed from sodium (Na) and chlorine (Cl). 2. Covalent Bonds Description: Formed when two atoms share electrons to fill their outer shells. Example: Water (H₂O) is formed when hydrogen (H) and oxygen (O) share electrons. 3. Hydrogen Bonds Description: A weak bond that occurs between a hydrogen atom in one molecule and an electronegative atom (like oxygen or nitrogen) in another molecule. Example: The bonds between water molecules, which give water its unique properties. Explain why water has polarity. Water has polarity because of its molecular structure. Here’s a simple breakdown: 1. Molecular Shape: Water (H₂O) has a bent shape, with the oxygen atom at the center and two hydrogen atoms at an angle. 2. Electronegativity: Oxygen is more electronegative than hydrogen, meaning it has a stronger pull on the electrons it shares with hydrogen. This results in the oxygen atom having a partial negative charge and the hydrogen atoms having partial positive charges. 3. Unequal Sharing of Electrons: Because the electrons are not shared equally, this creates a dipole moment—one end of the molecule is slightly negative (the oxygen side) and the other end is slightly positive (the hydrogen side). Importance of Polarity: Hydrogen Bonding: The polarity of water molecules allows them to form hydrogen bonds with each other and with other substances, which is crucial for many of water's unique properties, such as its high boiling point and ability to dissolve many substances. In summary, water’s polarity arises from its shape and the unequal sharing of electrons, making it a versatile and essential molecule in biological systems! What kind of bonds can water form? 1. Hydrogen Bonds Description: These are weak bonds that occur between the slightly positive hydrogen atoms of one water molecule and the slightly negative oxygen atoms of another water molecule. Importance: Hydrogen bonds are responsible for many of water's unique properties, such as its high boiling point, surface tension, and ability to dissolve various substances. 2. Polar Covalent Bonds Description: Within a water molecule, the bonds between the hydrogen and oxygen atoms are polar covalent bonds. This means that the electrons are shared unequally, resulting in a partial charge. Importance: These bonds give water its polar nature, allowing it to interact with other polar substances. 3. Ionic Interactions Description: While not a bond in the traditional sense, water can interact with ionic compounds (like salt) by surrounding and separating the individual ions. Importance: This property allows water to dissolve many ionic substances, making it a universal solvent. What are some other functions of water in the human body 1. Regulating Body Temperature Water helps maintain a stable temperature through processes like sweating and evaporation. 2. Transportation It serves as a medium for transporting nutrients, hormones, and waste products in the bloodstream. 3. Chemical Reactions Water is involved in many biochemical reactions, including digestion and metabolism. 4. Lubrication It acts as a lubricant in joints and tissues, reducing friction and facilitating movement. 5. Hydration Water is essential for maintaining cell structure and function, keeping cells hydrated and healthy. 6. Maintaining pH Balance Water helps regulate the body's pH levels, which is crucial for enzyme function and overall homeostasis. 7. Waste Removal It aids in the excretion of waste products through urine and sweat. What is the difference between polar and nonpolar molecules? Polar Molecules: Charge Distribution: Uneven distribution of electrons, leading to partial positive and negative charges. Example: Water (H₂O) has a bent shape, causing the oxygen atom to have a partial negative charge and the hydrogen atoms to have partial positive charges. Solubility: Generally soluble in water (hydrophilic) due to their ability to form hydrogen bonds. Nonpolar Molecules: Charge Distribution: Even distribution of electrons, resulting in no significant charge difference across the molecule. Example: Lipids, like oils, have symmetrical structures that do not have distinct positive or negative ends. Solubility: Generally not soluble in water (hydrophobic) because they cannot form favorable interactions with polar solvents. In summary, polar molecules have regions of partial charge due to unequal sharing of electrons, while nonpolar molecules have no such charge separation and tend to be hydrophobic. Where can the following functional groups be found: hydroxyl (OH), amino (NH2), and carboxyl (COOH) 1. Hydroxyl Group (–OH) Found In: Alcohols (e.g., ethanol) and sugars (e.g., glucose). Characteristics: Makes compounds polar and soluble in water, contributing to the formation of hydrogen bonds. 2. Amino Group (–NH₂) Found In: Amino acids (the building blocks of proteins) and certain neurotransmitters (e.g., dopamine). Characteristics: Acts as a base, accepting protons and increasing solubility in water. 3. Carboxyl Group (–COOH) Found In: Amino acids, fatty acids, and organic acids (e.g., acetic acid). Characteristics: Can donate a proton (acting as an acid) and contributes to the acidity of solutions; also important for forming peptide bonds in proteins. What are some examples of carbohydrates 1. Monosaccharides Glucose: A primary energy source for cells. Fructose: Found in fruits, often sweeter than glucose. Galactose: A component of lactose, found in milk. 2. Disaccharides Sucrose: Common table sugar, made of glucose and fructose. Lactose: Sugar found in milk, composed of glucose and galactose. Maltose: Malt sugar, made of two glucose units. 3. Oligosaccharides Raffinose: Found in beans, whole grains, and vegetables; composed of three to ten sugar units. 4. Polysaccharides Starch: A storage form of glucose in plants, found in foods like potatoes and rice. Glycogen: A storage form of glucose in animals, primarily found in the liver and muscles. Cellulose: A structural component of plant cell walls, not digestible by humans but important for dietary fiber. What elements are found in carbohydrates? Carbon (C): The backbone of carbohydrate molecules. Hydrogen (H): Usually bonded to carbon atoms; the ratio of hydrogen to oxygen is typically 2:1. Oxygen (O): Often found in hydroxyl groups (–OH) and as part of the carbonyl group (C=O). What is the difference between dehydration synthesis and hydrolysis reactions? Dehydration Synthesis Process: Involves the removal of a water molecule to join two smaller molecules together. Outcome: Forms a larger molecule (e.g., a polymer) from smaller subunits (e.g., monomers). Example: The formation of disaccharides (like sucrose) from monosaccharides (like glucose and fructose). Hydrolysis Process: Involves the addition of a water molecule to break down a larger molecule into smaller units. Outcome: Produces smaller molecules (e.g., monomers) from a larger molecule (e.g., a polymer). Example: The breakdown of starch into glucose units. Summary Dehydration Synthesis: Builds molecules by removing water. Hydrolysis: Breaks down molecules by adding water. Give examples of disaccharides. Sucrose: Composed of glucose and fructose; commonly known as table sugar, found in many plants. Lactose: Composed of glucose and galactose; found in milk and dairy products. Maltose: Composed of two glucose molecules; found in malted foods and beverages, and produced during the digestion of starch. What is the difference between glycogen and starch? 1. Structure Glycogen: Highly branched structure, with more frequent branching points than starch. It is composed entirely of glucose units. Starch: Primarily composed of two types of molecules: amylose (linear) and amylopectin (branched), with less frequent branching than glycogen. 2. Function Glycogen: Serves as the main storage form of glucose in animals. It can be rapidly mobilized to meet energy demands. Starch: Serves as the primary storage form of glucose in plants, providing energy reserves for plant metabolism and growth. 3. Location Glycogen: Mainly stored in the liver and muscle tissues of animals. Starch: Found in various plant tissues, particularly in seeds, tubers (like potatoes), and roots. What are some roles of lipids in the human body? lipids are vital for energy storage, cellular structure, hormone synthesis, and many other physiological functions in the body. What is the difference between saturated and unsaturated fatty acids? Give examples of each Saturated Fatty Acids Structure: Saturated fatty acids have no double bonds between carbon atoms; all carbon atoms are fully "saturated" with hydrogen atoms. Physical State: Typically solid at room temperature. Examples: ○ Stearic Acid: Found in animal fats and some vegetable oils. ○ Palmitic Acid: Common in palm oil and animal fat. Health Implications: High intake is associated with increased levels of LDL (bad cholesterol) and may raise the risk of heart disease. Unsaturated Fatty Acids Structure: Unsaturated fatty acids contain one or more double bonds between carbon atoms. This creates kinks in the structure, preventing tight packing. Physical State: Generally liquid at room temperature. Types: ○ Monounsaturated: One double bond (e.g., oleic acid found in olive oil). ○ Polyunsaturated: Multiple double bonds (e.g., omega-3 fatty acids like alpha-linolenic acid found in flaxseeds and fish oil). Health Implications: Considered heart-healthy; can lower LDL cholesterol levels and are beneficial for overall cardiovascular health. Summary Saturated: No double bonds, solid at room temperature (e.g., butter, coconut oil). Unsaturated: One or more double bonds, liquid at room temperature (e.g., olive oil, fish oil). Where are phospholipids found in the human body? 1. Cell Membranes Phospholipids are the main components of cell membranes, forming the lipid bilayer that provides structural integrity and flexibility to cells. This includes the membranes of all types of cells in the body. 2. Organelles Within cells, phospholipids are present in the membranes of various organelles, such as: ○ Nucleus: Surrounds the genetic material. ○ Mitochondria: Involved in energy production. ○ Endoplasmic Reticulum: Plays a role in protein and lipid synthesis. ○ Golgi Apparatus: Involved in modifying and packaging proteins and lipids. 3. Lipoproteins Phospholipids are also found in lipoproteins, which transport lipids in the bloodstream. They help to form the outer layer of these particles, facilitating the transport of cholesterol and triglycerides. 4. Surfactant in the Lungs In the lungs, phospholipids are a key component of pulmonary surfactant, which reduces surface tension in the alveoli, preventing collapse and aiding in gas exchange. Overall, phospholipids are essential for maintaining cellular integrity, facilitating communication, and supporting various physiological functions throughout the human body. Cholesterol is used for the production of what? Steroid Hormones Cholesterol serves as a precursor for steroid hormones, such as: ○ Cortisol: Involved in stress response and metabolism. ○ Estrogens: Female sex hormones that regulate reproductive functions. ○ Testosterone: Male sex hormone important for development and reproduction. 2. Bile Acids Cholesterol is converted into bile acids, which are essential for the digestion and absorption of dietary fats in the intestine. 3. Vitamin D Cholesterol is a precursor for the synthesis of vitamin D, which is important for bone health, immune function, and calcium metabolism. 4. Cell Membrane Structure Cholesterol is a critical component of cell membranes, helping to maintain membrane fluidity and integrity. In summary, cholesterol is vital for the production of hormones, bile acids, vitamin D, and for maintaining healthy cell membranes. What are some major functions of proteins? 1. Enzymatic Activity Proteins act as enzymes, catalyzing biochemical reactions that are crucial for metabolism and cellular processes. 2. Structural Support Proteins provide structure and support to cells and tissues. Examples include collagen in connective tissues and keratin in hair and nails. 3. Transport Proteins transport substances throughout the body. Hemoglobin, for instance, carries oxygen in the blood, while membrane proteins help transport molecules across cell membranes. 4. Defense Antibodies are proteins that play a key role in the immune system, helping to identify and neutralize foreign invaders like bacteria and viruses. 5. Signaling Many proteins function as hormones or receptors, facilitating communication between cells and coordinating various physiological processes (e.g., insulin regulating blood sugar levels). 6. Movement Proteins are involved in muscle contraction and movement. Actin and myosin are key proteins in muscle fibers. 7. Storage Some proteins serve as storage molecules for essential nutrients. For example, ferritin stores iron in the body. 8. Regulation of Gene Expression Certain proteins, like transcription factors, help regulate the expression of genes, influencing cellular function and activity. Overall, proteins are crucial for virtually every biological process in the body, contributing to structure, function, and regulation of the body's tissues and organs. What functional groups are found in proteins? What is the difference between essential and non-essential amino acids? Functional Groups in Proteins Proteins are made up of amino acids, which contain the following functional groups: 1. Amino Group (–NH₂): This group consists of a nitrogen atom bonded to two hydrogen atoms. It acts as a base and can accept protons. 2. Carboxyl Group (–COOH): This group consists of a carbon atom double-bonded to an oxygen atom and single-bonded to a hydroxyl group. It can donate protons, making amino acids acidic. 3. R Group (Side Chain): Each amino acid has a unique side chain (R group) that determines its properties and functionality. The nature of the R group can be polar, nonpolar, acidic, or basic. Difference Between Essential and Non-Essential Amino Acids Essential Amino Acids: ○ Definition: These amino acids cannot be synthesized by the body and must be obtained through the diet. ○ Examples: There are nine essential amino acids, including lysine, leucine, and valine. Non-Essential Amino Acids: ○ Definition: These amino acids can be synthesized by the body, so they do not need to be obtained directly from food. ○ Examples: Common non-essential amino acids include alanine, aspartic acid, and glutamic acid. Summary Functional Groups: Proteins contain amino groups, carboxyl groups, and unique R groups. Essential vs. Non-Essential Amino Acids: Essential amino acids must be consumed in the diet, while non-essential amino acids can be produced by the body. Describe the levels of protein structure and give examples of each.. Primary Structure Description: The primary structure is the linear sequence of amino acids in a polypeptide chain. This sequence is determined by the gene encoding the protein. Example: Insulin, a hormone that regulates blood sugar levels, has a specific amino acid sequence that is crucial for its biological activity. 2. Secondary Structure Description: The secondary structure refers to local folding of the polypeptide chain into structures such as alpha helices and beta-pleated sheets, stabilized by hydrogen bonds between the backbone atoms. Example: The alpha helix in the protein keratin, which provides structural support in hair and nails. 3. Tertiary Structure Description: The tertiary structure is the overall three-dimensional shape of a single polypeptide chain, formed by interactions among R groups (side chains) and between the polypeptide and its environment. These interactions include hydrogen bonds, ionic bonds, hydrophobic interactions, and disulfide bridges. Example: Myoglobin, a protein that carries oxygen in muscle cells, has a complex tertiary structure that allows it to effectively bind oxygen. 4. Quaternary Structure Description: The quaternary structure refers to the assembly of multiple polypeptide chains (subunits) into a larger functional protein complex. The interactions between these subunits can involve various types of bonding. Example: Hemoglobin, which consists of four subunits (two alpha and two beta chains), is responsible for transporting oxygen in the blood. Summary Primary: Amino acid sequence (e.g., insulin). Secondary: Local folding (e.g., alpha helices in keratin). Tertiary: Overall 3D shape of a single chain (e.g., myoglobin). Quaternary: Assembly of multiple chains (e.g., hemoglobin). What does denaturing do to proteins? Which bonds are affected? denaturing refers to the process by which a protein loses its native structure due to the disruption of various interactions that maintain its shape. This change can result in the loss of its biological function. Effects of Denaturing on Proteins 1. Loss of Secondary Structure: Denaturing can unravel alpha helices and beta-pleated sheets, leading to a loss of the protein’s characteristic folding. 2. Loss of Tertiary Structure: The overall three-dimensional shape of the protein is altered, which is critical for its function. 3. Loss of Quaternary Structure: If the protein consists of multiple subunits, denaturation can lead to the dissociation of these subunits. Bonds Affected by Denaturing Denaturing affects several types of bonds and interactions within the protein: 1. Hydrogen Bonds: These bonds, which stabilize secondary and tertiary structures, are often disrupted by changes in temperature, pH, or chemical agents. 2. Ionic Bonds: Electrostatic interactions between charged side chains can be disrupted, especially with changes in pH. 3. Hydrophobic Interactions: The unfolding of the protein can expose hydrophobic regions that were previously buried in the core, leading to the aggregation of denatured proteins. 4. Disulfide Bridges: Covalent bonds formed between cysteine residues can be broken by reducing agents, leading to structural changes. Summary Denaturing results in the loss of a protein's structure and function by disrupting hydrogen bonds, ionic bonds, hydrophobic interactions, and disulfide bridges. What does hydrolysis do to proteins? Which bonds are affected? Hydrolysis is a biochemical process that breaks down proteins into smaller peptides or individual amino acids by adding water. This reaction is essential for digestion and the recycling of proteins in the body. Effects of Hydrolysis on Proteins 1. Breaks Down Peptide Bonds: Hydrolysis specifically cleaves the peptide bonds that link amino acids together in a protein chain. 2. Results in Smaller Fragments: The protein is broken down into smaller peptides or free amino acids, which can then be utilized by the body for various functions, including the synthesis of new proteins. Bonds Affected by Hydrolysis Peptide Bonds: These covalent bonds form between the amino group of one amino acid and the carboxyl group of another during protein synthesis. Hydrolysis breaks these bonds, leading to the separation of amino acids. Summary Hydrolysis of proteins involves the addition of water to break peptide bonds, resulting in the breakdown of proteins into smaller peptides or amino acids, which are crucial for various metabolic processes. How do enzymes work, and what effect do they have on activation energy? How Enzymes Work 1. Substrate Binding: Enzymes have specific active sites that bind to substrates (the reactants in a reaction). The binding often involves complementary shapes and chemical interactions, leading to the formation of an enzyme-substrate complex. 2. Induced Fit: Upon substrate binding, the enzyme may undergo a conformational change that enhances the interaction between the enzyme and the substrate, further stabilizing the transition state of the reaction. 3. Catalysis: The enzyme facilitates the conversion of substrates into products through various mechanisms, such as: ○ Proximity and Orientation: Bringing substrates close together in the correct orientation to react. ○ Strain: Distorting substrate bonds to make them easier to break. ○ Microenvironment: Providing an optimal environment (like acidity or polarity) for the reaction to occur. 4. Product Release: After the reaction occurs, the products are released from the active site, and the enzyme returns to its original state, ready to catalyze another reaction. Effect on Activation Energy Lowering Activation Energy: Enzymes significantly reduce the activation energy required for a reaction. This means that reactions can occur more quickly and at lower temperatures than would be possible without the enzyme. Summary Enzymes work by binding substrates, forming an enzyme-substrate complex, and facilitating the conversion of substrates to products while lowering the activation energy required for the reaction. This increases the reaction rate and efficiency in biological processes. What causes enzymes to not work? 1. Denaturation: High temperatures or extreme pH can unfold the enzyme, altering its structure. 2. Inhibition: ○ Competitive Inhibition: Substrate-like molecules block the active site. ○ Non-competitive Inhibition: Molecules bind elsewhere, changing the enzyme's shape. 3. Cofactor/Coenzyme Availability: Lack of necessary cofactors or coenzymes can inhibit activity. 4. Substrate Availability: Insufficient substrates reduce enzyme activity. 5. Allosteric Regulation: Regulatory molecules can enhance or inhibit activity by binding to allosteric sites. 6. Chemical Damage: Exposure to toxins or harsh conditions can alter the enzyme’s structure. These factors can hinder enzyme function and reduce reaction rates. What nitrogenous bases are found in DNA? Adenine (A) Thymine (T) Cytosine (C) Guanine (G) What nitrogenous bases are found in RNA? 1. Adenine (A) 2. Uracil (U) (replaces thymine found in DNA) 3. Cytosine (C) 4. Guanine (G) What are some differences between DNA and RNA? Structure DNA: Double-stranded helix. RNA: Single-stranded. 2. Sugar DNA: Contains deoxyribose sugar. RNA: Contains ribose sugar. 3. Nitrogenous Bases DNA: Adenine (A), Thymine (T), Cytosine (C), Guanine (G). RNA: Adenine (A), Uracil (U), Cytosine (C), Guanine (G). 4. Function DNA: Stores genetic information and instructions for development and functioning. RNA: Involved in protein synthesis (messenger RNA), as well as roles in regulation and catalysis (e.g., ribosomal RNA, transfer RNA). 5. Location DNA: Primarily found in the nucleus (in eukaryotes). RNA: Found in the nucleus and cytoplasm. What is the cellular currency to do work, and where is it produced? Cellular Currency: Adenosine triphosphate (ATP) Function: Provides energy for biological processes (e.g., muscle contraction, protein synthesis, active transport) Production Site: Primarily in the mitochondria Process: Produced through cellular respiration (glycolysis, citric acid cycle, oxidative phosphorylation) Alternative Production: Some ATP generated in the cytoplasm through glycolysis What is the cell membrane composed of? Phospholipid Bilayer: Two layers of phospholipids with hydrophilic (water-attracting) heads facing outward and hydrophobic (water-repelling) tails facing inward. Proteins: Integral and peripheral proteins are embedded within or attached to the phospholipid bilayer, playing roles in transport, signaling, and structural support. Carbohydrates: Often attached to proteins (glycoproteins) or lipids (glycolipids), these molecules are involved in cell recognition and communication. Cholesterol: Interspersed within the phospholipid bilayer, cholesterol helps to maintain membrane fluidity and stability. This composition allows the cell membrane to be selectively permeable, regulating the movement of substances in and out of the cell. What is the fluid called on the inside and outside of the cell? Fluid Inside the Cell: Cytosol: The liquid component of the cytoplasm, excluding organelles. Fluid Outside the Cell: Extracellular Fluid: Includes interstitial fluid (surrounding cells in tissues) and blood plasma (in the circulatory system). Describe the role of the glycocalyx. Protection: It acts as a protective barrier for the cell, shielding it from mechanical damage and chemical stress. Cell Recognition: The glycocalyx contains glycoproteins and glycolipids that help cells recognize each other, facilitating cell-cell communication and interaction. Adhesion: It aids in the adhesion of cells to surfaces and to each other, which is crucial for tissue formation and integrity. Filtration: The glycocalyx can help filter substances that enter and exit the cell, contributing to selective permeability. Immune Response: It plays a role in the immune system by helping to identify foreign cells and pathogens, aiding in immune recognition and response. Overall, the glycocalyx is essential for maintaining cellular function and communication in a variety of biological processes. What are some transmembrane proteins covered in class? Channel Proteins: Facilitate the passive transport of ions and molecules across the membrane (e.g., sodium channels, potassium channels). Carrier Proteins: Bind to specific substances and help transport them across the membrane (e.g., glucose transporters). Receptor Proteins: Receive and transmit signals from the extracellular environment to the inside of the cell (e.g., insulin receptors, G-protein coupled receptors). Enzymatic Proteins: Catalyze biochemical reactions at the membrane surface (e.g., adenylate cyclase). Adhesion Proteins: Help cells adhere to one another and to the extracellular matrix (e.g., integrins). These proteins play crucial roles in various cellular functions, including transport, communication, and structural support. What is the role of the mitochondria? Energy Production: Mitochondria are known as the "powerhouses of the cell" because they produce adenosine triphosphate (ATP) through cellular respiration, primarily via the citric acid cycle and oxidative phosphorylation. Regulation of Metabolism: They are involved in various metabolic pathways, including the metabolism of carbohydrates, fats, and proteins. Calcium Storage: Mitochondria help regulate intracellular calcium levels, which are important for cellular signaling and muscle contraction. Apoptosis: Mitochondria play a role in programmed cell death (apoptosis) by releasing factors that activate the apoptotic pathways. Heat Production: In some specialized cells, mitochondria can generate heat through a process called thermogenesis. What is the rough endoplasmic reticulum, and what organelle is embedded in it to give it the rough appearance? What occurs in this organelle The rough endoplasmic reticulum (RER) is a type of endoplasmic reticulum characterized by its studded appearance due to the presence of ribosomes on its cytoplasmic surface. This gives the RER a "rough" texture. Key Functions of the Rough Endoplasmic Reticulum: Protein Synthesis: Ribosomes on the RER are responsible for synthesizing proteins, particularly those destined for secretion, incorporation into the cell membrane, or for use in lysosomes. Protein Folding and Modification: The RER provides an environment for newly synthesized proteins to fold properly and undergo post-translational modifications, such as glycosylation (adding sugar molecules). Quality Control: The RER monitors protein folding and can retain improperly folded proteins for refolding or degradation, ensuring that only correctly folded proteins are transported to their next destination. In summary, the rough endoplasmic reticulum is crucial for the synthesis and processing of proteins, with ribosomes embedded in its membrane giving it a rough appearance. What type of bonds do chaperone proteins assist in forming? Type of Bonds: Chaperone proteins assist in forming: Hydrogen Bonds: Stabilize the structure of proteins. Non-Covalent Interactions: Include ionic bonds and hydrophobic interactions that help maintain proper folding. Function: Prevent misfolding and aggregation of proteins. Facilitate correct three-dimensional structure for protein functionality. What is the role of the Golgi apparatus? Protein Modification: It modifies proteins synthesized in the rough endoplasmic reticulum (RER) by adding carbohydrate groups (glycosylation) and other chemical modifications. Sorting and Packaging: The Golgi apparatus sorts proteins and lipids received from the RER and packages them into vesicles for transport to their final destinations, such as the cell membrane, lysosomes, or outside the cell. Creation of Lysosomes: It helps in the formation of lysosomes by packaging digestive enzymes. Synthesis of Certain Macromolecules: The Golgi apparatus is involved in the synthesis of some polysaccharides and glycoproteins. Overall, the Golgi apparatus is essential for processing, sorting, and distributing proteins and lipids within the cell. What do lysosomes do, and what produces them? lysosomes are membrane-bound organelles that play a crucial role in cellular digestion and waste processing. Here’s what they do: Digestive Functions: Lysosomes contain hydrolytic enzymes that break down various biomolecules, including proteins, lipids, carbohydrates, and nucleic acids. This process helps recycle cellular components and remove waste. Autophagy: They help in the degradation of damaged or obsolete organelles and proteins, a process known as autophagy, which is essential for cellular maintenance and renewal. Defense Mechanism: Lysosomes can also digest foreign substances, such as bacteria and viruses, contributing to the cell’s immune defense. Production: Lysosomes are produced by the Golgi apparatus. The Golgi modifies enzymes synthesized in the rough endoplasmic reticulum and packages them into vesicles that mature into lysosomes. What is the role of the smooth endoplasmic reticulum? he smooth endoplasmic reticulum (SER) has several important roles in the cell: Lipid Synthesis: The SER is involved in the synthesis of lipids, including phospholipids and cholesterol, which are essential for cell membrane formation. Detoxification: It helps detoxify harmful metabolic byproducts and drugs, particularly in liver cells. Calcium Storage: The SER stores calcium ions, which are important for various cellular processes, including muscle contraction and signaling. Metabolism of Carbohydrates: It plays a role in carbohydrate metabolism, such as the conversion of glucose-6-phosphate to glucose. Hormone Production: In endocrine cells, the SER is involved in the synthesis of steroid hormones. Overall, the smooth endoplasmic reticulum is crucial for lipid metabolism, detoxification, and calcium regulation in the cell. What is the largest organelle in the cell, and what does it store? Largest Organelle: Nucleus Storage: Genetic Material: Stores DNA (deoxyribonucleic acid). Nucleoplasm: Gel-like substance that provides a medium for molecular interactions. Functions: Site of DNA replication and transcription. Houses the nucleolus, which produces ribosomal RNA (rRNA) and assembles ribosomes. What is the role of the nucleoli? Ribosome Production: The primary function of the nucleolus is to synthesize ribosomal RNA (rRNA) and assemble ribosomal subunits, which are essential for protein synthesis. Processing of rRNA: It processes and modifies rRNA to ensure it is properly folded and ready for incorporation into ribosomes. Assembly of Ribosomal Subunits: The nucleolus assembles the rRNA with proteins to form the small and large subunits of ribosomes, which are then transported to the cytoplasm for protein synthesis. Regulation of Cellular Functions: The nucleolus may also play a role in regulating cellular stress responses and the cell cycle. Overall, the nucleolus is essential for ribosome biogenesis and thus for the overall process of protein synthesis in the cell. What are four examples of passive transport? Diffusion: The movement of molecules from an area of higher concentration to an area of lower concentration until equilibrium is reached. For example, oxygen and carbon dioxide gases move in and out of cells via diffusion. Facilitated Diffusion: This process involves the use of specific proteins (carrier or channel proteins) to help molecules, such as glucose or ions, move across the cell membrane along their concentration gradient. Osmosis: The diffusion of water molecules across a semipermeable membrane from an area of lower solute concentration to an area of higher solute concentration until equilibrium is achieved. Filtration: The movement of water and solutes through a membrane due to hydrostatic pressure, as seen in the kidneys during urine formation. What are four examples of active transport? Sodium-Potassium Pump: This pump actively transports sodium ions (Na⁺) out of the cell and potassium ions (K⁺) into the cell against their concentration gradients, using ATP for energy. Proton Pump: This pump moves protons (H⁺ ions) across membranes, such as in the stomach lining, creating an acidic environment necessary for digestion. Endocytosis: This process involves the engulfing of materials by the cell membrane to form vesicles. Types of endocytosis include: Phagocytosis: Engulfing large particles or cells (e.g., immune cells taking in bacteria). Pinocytosis: Taking in liquid and small solutes. Exocytosis: The process of vesicles fusing with the plasma membrane to release their contents outside the cell, such as the secretion of neurotransmitters or hormones. What are the major differences between active and passive transport? Energy Requirement Active Transport: Requires energy (ATP).low to high Passive Transport: Does not require energy. High to low Direction of Movement Active Transport: Moves substances from low to high concentration. Passive Transport: Moves substances from high to low concentration. Types of Transport Active Transport: Includes sodium-potassium pumps, proton pumps, endocytosis, and exocytosis. Passive Transport: Includes diffusion, facilitated diffusion, osmosis, and filtration. Specificity Active Transport: Often specific to certain molecules. Passive Transport: Generally relies on natural movement and may be less selective. What are factors that affect the rate of diffusion? Concentration Gradient: A larger difference in concentration between two areas increases the rate of diffusion. Temperature: Higher temperatures increase molecular movement, which enhances the rate of diffusion. Molecular Size: Smaller molecules diffuse more quickly than larger ones. Medium of Diffusion: Diffusion occurs faster in gases than in liquids, and faster in liquids than in solids. Surface Area: A larger surface area allows for more molecules to diffuse simultaneously, increasing the rate. Distance: Shorter distances facilitate quicker diffusion. What is the difference between a solvent and solutes? Which one moves duringn osmosis? Solvent: A substance that dissolves a solute; it is typically the component present in the greater amount. In biological systems, water is the most common solvent. Solute: A substance that is dissolved in a solvent; it is present in a lesser amount. Examples include salts, sugars, and gases. Movement During Osmosis During osmosis, the solvent (usually water) moves across a semipermeable membrane from an area of lower solute concentration to an area of higher solute concentration. This movement helps equalize solute concentrations on both sides of the membrane. Understand the differences between isotonic, hypotonic, and hypertonic environments. Isotonic Definition: Solutions with equal solute concentrations on both sides of a membrane. Effect on Cells: No net movement of water; cells retain their shape and size. Hypotonic Definition: Solutions with a lower solute concentration compared to the inside of the cell. Effect on Cells: Water enters the cell, causing it to swell and potentially burst (lyse). Hypertonic Definition: Solutions with a higher solute concentration compared to the inside of the cell. Effect on Cells: Water leaves the cell, leading to cell shrinkage (crenation). Are phagocytosis and pinocytosis examples of active or passive transport? What is the difference between the two? Phagocytosis and pinocytosis are examples of active transport. Differences: Phagocytosis: ○ Definition: The process by which a cell engulfs large particles or other cells. ○ Function: Often used by immune cells to remove pathogens or debris. ○ Mechanism: The cell membrane extends around the particle, forming a vesicle that brings it into the cell. Pinocytosis: ○ Definition: The process by which a cell engulfs small droplets of extracellular fluid along with dissolved solutes. ○ Function: Allows the cell to sample its environment and take in nutrients. ○ Mechanism: The cell membrane invaginates to form small vesicles that capture fluid and solutes. Both processes require energy to move substances into the cell against their concentration gradients. How is energy created from adenosine triphosphate (ATP)? What is broken to create this energy? Energy is created from adenosine triphosphate (ATP) through a process called hydrolysis. Key Points: Breaking the Bonds: The energy is released when the terminal phosphate bond in ATP is broken, converting ATP into adenosine diphosphate (ADP) and an inorganic phosphate (Pi). Energy Release: This reaction releases energy that can be used by the cell for various functions, such as muscle contraction, active transport, and biochemical reactions. Recycling ATP: ADP can be converted back into ATP through processes like cellular respiration, where energy from food molecules is used to reattach a phosphate group. In summary, the energy stored in ATP is released when the high-energy phosphate bond is broken during hydrolysis.