Unit-3 Carbonyl Compounds Part-A PDF
Document Details
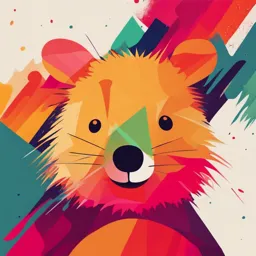
Uploaded by JovialHydrogen2487
RK University
Ashwini Dhruv
Tags
Related
- Intro To Organic Chemistry Summary PDF
- Med-108 Organic Chemistry Aldehydes & Ketones 2024 PDF
- Organic Chemistry Bundle PDF
- Chemistry Notes for Class 12 Chapter 12 Aldehydes, Ketones and Carboxylic Acids PDF
- AQA Chemistry A-level 3.3.8 Aldehydes and Ketones Detailed Notes PDF
- Organic Chemistry III Problem Set No. 1 PDF
Summary
This document is a study guide on aldehydes and ketones, detailing their properties, reactions, and nomenclature. It's geared towards undergraduate students.
Full Transcript
Prepared By: Ashwini Dhruv Assistant Professor RK University Unit-5 I BP202T Pharmaceutical Organic Chemistry-I Electromeric effect, aldol condensation, Crossed Aldol condensation, Cannizzaro reaction, Crossed Cannizzaro reaction, Benzoin condensation, Perkin condensation, qualitativ...
Prepared By: Ashwini Dhruv Assistant Professor RK University Unit-5 I BP202T Pharmaceutical Organic Chemistry-I Electromeric effect, aldol condensation, Crossed Aldol condensation, Cannizzaro reaction, Crossed Cannizzaro reaction, Benzoin condensation, Perkin condensation, qualitative tests, Structure and uses of Formaldehyde, Paraldehyde, Acetone, Chloral hydrate, Hexamine, Benzaldehyde, Vanillin, Cinnamaldehyde. Aldehydes are compounds of the general formula RCHO; ketones are compounds of the general formula RR’ CO. The groups R and R’ may be aliphatic or aromatic. Both aldehydes and ketone contain carbonyl group, C=O and are often referred to collectively as carbonyl compounds. It is carbonyl group that largely determines chemistry of aldehydes and ketones. In aldehydes, the carbonyl group has one hydrogen atom attached to it together with either a 2nd hydrogen atom or a hydrogen group which may be an alkyl group or one containing a benzene ring. In ketones, the carbonyl group has 2 hydrocarbon groups attached to it. These can be either the ones containing benzene rings or alkyl groups. Ketone does not have a hydrogen atom attached to the carbonyl group. 1. Their polarity makes aldehydes and ketones have higher boiling points than alkenes of similar molecular weight. 2. Aldehydes and ketones are not hydrogen bond donors (they can’t donate a proton); therefore, they have lower boiling points than alcohols of similar molecular weight. 3. Aldehydes and ketones are hydrogen bond acceptors; this makes them have considerable solubility's in water. The carbonyl carbon of an aldehyde or ketone is sp2 -hybridized. The bond angle is close to 120° (trigonal planar). The carbon-oxygen double bond consists of: i. A (σ) C-O bond ii. A (π) C=O bond We can compare the C=O bond length to those of C=C double bonds 1. COMMON SYSTEM: The name of simple aldehydes are derived from the common names of the corresponding carboxylic acids. The characteristic -ic acid ending in common name of the carboxylic acids is dropped and replaced by -aldehyde. For e.g.: Formic acid (HCOOH) is replaced with corresponding aldehyde i.e. Formaldehyde (HCHO) Substituted aldehydes can be named as derivatives of the parent, continuous-chain aldehyde. The parent compound must contain the aldehyde group, and the carbon atoms on carbon chain are assigned Greek letters in following manner: δ ϒ β α C–C–C–C–C=O H The names and Greek-letter designations of the substituents indicate their location on longest chain. 2. IUPAC SYSTEM: The IUPAC system of naming aldehyde uses the parent alkane name corresponding to the longest continuous chain containing the aldehyde group the final –e of the alkane name is dropped and replaced by –al of the characteristic ending for an aldehyde. The numbering of longest continuous chain starts with C-1 as the carbon bearing the aldehyde group. Substituents are indicated by name and position number. Following examples are illustrated below. 1. Common system: Ketones can be named by using the alkyl or aryl group names of the substituent that are attached to the carbonyl group and following them by the word ketone. There is also simple, common name for the simplest ketone i.e. Acetone (CH3COOH3) 1. IUPAC system: The longest chain that contains the keto group is used as the parent compound in IUPAC system. Ketone names are derived from the corresponding alkane name by dropping –e ending and replacing it with –one. The substituents are identified by name and position number, and the longest chain is numbered so that the carbon-oxygen double bond is given the lowest possible number. The word -oxo is sometimes used as prefix for carbonyl group. ELECTROMERIC EFFECT: The instantaneous formation of a dipole in the molecule of an organic compound due to the complete transfer of shared pi electron pairs to one of the atoms under the influence of an attacking reagent is referred to as the Electromeric effect. This effect can be observed in organic compounds that contain at least one multiple bond. When the atoms participating in this multiple bond come under the influence of an attacking reagent, one pi bonding pair of electrons is completely transferred to one of the two atoms. The Electromeric effect is a temporary effect that remains as long as the attacking reagent is present and exposed to the organic compound. Once this attacking reagent is removed from the system, the molecule that was polarized goes back to its original state. IMPORTANT POINTS IN ELECTROMERIC EFFECT: 1. It is temporary effect 2. Only occurs in multiple bonds. 3. Displacement of pi (π) electrons only. 4. Only occur in the presence of attacking reagent TYPES OF ELECTROMERIC EFFECT: The Electromeric effect can be broken down into two types, namely the +E effect and the -E effect. This classification is done based on the direction in which the electron pair is transferred. +E EFFECT: This effect occurs when the electron pair of the pi bond is moved towards the attacking reagent. The +E effect can be observed in the addition of acid to alkenes. The attacking reagent attaches itself to the atom which obtained an electron pair in the transfer. The +E effect is generally observed when the attacking reagent is an electrophile and the pi electrons are transferred towards the positively charged atom. An example where the +E effect occurs is the protonation of ethene which is illustrated below. -E EFFECT: This effect occurs when the electron pair of the pi bond is moved away from the attacking reagent. The attacking reagent attaches itself to the positively charged atom in the molecule, i.e. the atom which lost the electron pair in the transfer. The -E effect is generally observed when the attacking reagent is a nucleophile and the pi electrons are transferred to the atom which the attacking reagent will not bond with. An example where the -E effect occurs would be the addition of nucleophiles to carbonyl compounds as illustrated below. +E effect -E effect The -E effect is observed when the shared pair of The +E effect occurs when the shared electron pair electrons is transferred away from the attacking is transferred toward the attacking reagent. reagent. The nucleophilic reagent attacks the atom that has lost The attacking reagent forms a bond with the atom its shared pair of electrons and forms a bond with that has received the shared pair of electrons. it. This phenomenon is greatly observed in reactions This +E effect can be observed in the reaction involving carbonyl carbon atoms like aldehydes and involving the addition of acid to alkenes. ketones. Here, π electrons of the multiple bonds are Here, π electrons of the multiple bonds are transferred transferred to the atom to which the reagent gets to the atom to which the reagent does not get attached. attached. The attacking reagent is an Electrophile The attacking reagent is an Nucleophile For e.g.: A protonation of ethene. For e.g.: The reaction of an aldehyde with a cyanide ion. 1. Oxidation of alcohol 2. Catalytic dehydrogenation of alcohol 3. Oxidation of alkene 4. Hydration of alkynes 5. Hydrolysis of gem-dihalides 6. Reduction of acid chlorides 7. From nitriles using Grignard reagents 8. Oxo process 1. OXIDATION OF ALCOHOL: Alcohols and ketones can be prepared by controlled distillation of 1 and 2 alcohol using an acidified solution of K2Cr2O7 & KMnO4. 1 alcohols produce aldehydes 2. CATALYTIC DEHYDROGENATION OF ALCOHOL: Aldehydes may be prepared by passing the vapors of 1 alcohols over a copper catalyst heated to about 300 C 3. OXIDATION OF ALKENE (OZONOLYSIS): Aldehydes & ketones can be obtained by ozonolysis of alkenes. This involves the treatment of alkenes with ozone to give ozonides. The ozonides are not isolated because they are often explosive in a dry state. They are reduced with Zn/H2O or (CH3)3S to aldehydes or ketones H2O + 4. HYDRATION OF ALKYNES: Hydration of acetylene yields acetaldehyde. Water adds to acetylene in the presence of mercuric sulfate and sulphuric acid to form an unstable enol-intermediate. This intermediate rearranges to acetaldehyde + H2SO4 5. HYDROLYSIS OF GEM HALIDES: A gem-halide is a compound that has two halogen atoms attached to the same carbon. Aldehydes are prepared by alkaline hydrolysis of those gem halides in which 2 halogen atoms are attached to the terminal carbon. -Added NaOH -Removed + -HCl -NaCl 6. REDUCTION OF ACID CHLORIDES: Aldehydes can be prepared by hydrogenation of acid chlorides in the presence of palladium supported over barium sulfate. This reaction is also called as a Rosenmund reaction. “Ketone cannot be prepared by this method” 7. FROM NITRILES USING GRIGNARD REAGENT: A Grignard reagent attacks nitrile to give the magnesium salt of imines. Acid hydrolysis of the imines leads to ketone 8. OXO PROCESS: Alkene reacts with hydrogen and carbon monoxide to form aldehyde. CO 1. Nucleophilic addition reaction 2. Aldol Condensation reaction 3. Crossed Aldol Condensation reaction 4. Benzoin Condensation Reaction 5. Perkin Condensation Reaction 6. Cannizaro Reaction 7. Crossed Cannizaro Reaction The carbonyl group of aldehyde and ketones is a highly polar group. It may be represented as: The positively charged carbon is readily attacked by electron-rich nucleophiles. The negatively charged oxygen is attacked by electron-deficient electrophiles Step-1: The nucleophile (Nu:) attacks the positively charged carbonyl carbon to form a new bond. As the new bond is formed, the bond between carbon and oxygen is broken. The electron pair goes to oxygen, which acquires a negative charge. Step-2: The electrophile (e.g.: H+) attacks negatively charged oxygen to form an addition product. 1. Base-Catalyzed Addition: The nucleophilic addition reactions of carbonyl compounds may be catalyzed by acids or bases. Bases convert a weak neutral nucleophile to a strong one by removing a proton. The strong nucleophile then adds to the carbonyl group as shown here: 2. Acid-Catalyzed Addition: Step-1: The hydrogen ion from the acid attacks the negatively charged carbonyl oxygen to give protonated carbonyl group. The protonated carbonyl group is resonance stabilized. Step-2: The nucleophile attacks the protonated carbonyl group to form the additional product. A. ADDITION OF WATER: Water can add to a carbonyl group to form a 1,1 diol called a gem- diol or hydrate. B. ADDITION OF HYDROGEN CYANIDE: Hydrogen cyanide can add to the carbonyl group of an aldehyde or ketone to form cyanohydrins. C. ADDITION OF GRIGNARD REAGENT: Aldehydes and ketones react with Grignard reagents to give an addition product that can be hydrolyzed to give alcohol. + MgO + HI The aldol condensation reaction is an organic reaction introduced by Charles Wurtz, who first prepared the β-hydroxy aldehyde from acetaldehyde in 1872. Aldehydes containing –hydrogen undergoes self-addition in the presence of a base to form products called aldols. The reaction is called Aldol Condensation. ALDOLS: An aldol or aldol adduct (from "aldehyde alcohol") is a hydroxy ketone or aldehyde and is the product of aldol addition (as opposed to aldol condensation, which produces an α,β-unsaturated carbonyl moiety). When used alone, the term "aldol" may also refer specifically to the compound 3-hydroxy butanal GENERAL ALDOL CONDENSATION: Aldol Condensation can be defined as an organic reaction in which an enolate ion reacts with a carbonyl compound to form β-hydroxy ketone or β-hydroxy aldehyde, followed by dehydration to give a conjugated enone. Aldol Condensation plays a vital role in organic synthesis, creating a path to form carbon- carbon bonds. MECHANISM OF REACTION: Step 1: The enolate ion is formed. Step 2: The enolate ion attacks the carbonyl group of another un-ionized aldehyde molecule. MECHANISM OF REACTION: Step-3: The negative oxygen in the product accepts a proton from water to give aldol. + The condensation reaction between two different molecules of an aldehyde or ketone in a protic solvent such as water or alcohol constitutes the crossed aldol reaction. When condensation is between two different carbonyl compounds, it is called crossed aldol condensation. If both carbonyl compounds have -hydrogens, then we can’t control which is the donor and which is the acceptor. But if we choose one carbonyl compound that does not have -hydrogens, it can only act as an acceptor. Then, but adding the donor to a dilute solution of the acceptor, we can control the reaction to give only one product. KETOL CONDENSATION: Ketones containing –hydrogen also undergo aldol condensation to form Ketols. For example, 2 molecules of acetone combine with each other in the presence of Ba(OH)2 to form 4-hydroxy-4-methyl 2-pentanone. (diacetone alcohol) HISTORY: The Benzoin condensation is a condensation reaction between two aromatic aldehydes, especially benzaldehyde that is catalyzed by a cyanide. The reaction product is an aromatic acyloin with benzoin as the parent compound. The history of this reaction goes back to 1832 when it was reported by German scientists Justus Von Liebig and Friedrich Wohler. GENERAL PRINCIPLE: The benzoin condensation is the condensation between two molecules of benzaldehyde to form benzoin in the presence of a cyanide catalyst (e.g., NaCN and KCN) or thiamine (vitamin B). The structure of benzoin is that of a ketone. It consists of acetophenone bearing hydroxy and phenyl substituents at the alpha-position (α‐hydroxyl ketone). Its IUPAC name is 2-hydroxy-1,2-diphenylethanone with a 212.24 g/mol molecular mass. A cyanide ion usually catalyzes benzoin condensation. 2 MECHANISM: STEP 1: The standard method for performing the benzoin condensation begins with benzaldehyde treated with a catalytic amount of sodium cyanide in the presence of a base. The cyanide ion forms a stable cyanohydrin intermediate. MECHANISM: STEP 2: The second step is the condensation reaction that occurs between the cyanohydrin and the benzaldehyde. MECHANISM: STEP 3: In the third phase, rearrangement and the cyanide ions' resulting in the formation of benzoin. Perkin reaction involves the treatment of an aromatic aldehyde with acidic anhydride of an aliphatic acid and sodium salt of that acid. Products are , –unsaturated acids. For e.g.: benzaldehyde reacts with acetic anhydride in the presence of sodium acetate at 180 C to cinnamic acid. (CH3CO)2O/CH3COONa STEPS OF MECHANISM: It involves the following 5 steps: 1. Formation of the anion of acetic anhydride A 2. Addition of A to benzaldehyde gives B. Notice that anion adds to the carbonyl carbon of benzaldehyde. 3. Protonation of B gives C. Proton is supplied by acetic acid from Step-1. 4. Internal proton transfer in C followed by elimination of water molecule gives D 5. Hydrolysis of D gives Cinnamic acid STEPS OF MECHANISM: Step-1 Step-2 Step-3 H+ Step-5 Step-4