Vectors for Gene Cloning: Plasmids and Bacteriophages PDF
Document Details
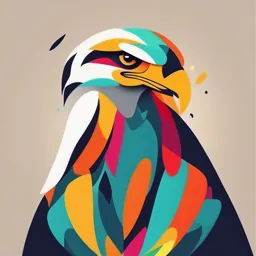
Uploaded by ClearedMossAgate3840
جامعة تبريز كلية العلوم الطبيعية
2016
T.A. Brown
Tags
Summary
This chapter discusses plasmids and bacteriophages as vectors in gene cloning. It explores their key features, including replication, size, and various types, and their applications in the laboratory. The text also examines plasmid conjugation and classification.
Full Transcript
Chapter 2 Vectors for Gene Cloning: Plasmids and Bacteriophages Chapter contents CHAPTER CONTENTS 2.1 Plasmids 2.2 Bacteriophages A DNA molecule needs to display several features to be able to act as a vector for gene cloning. Most importantly, it must be able to replicate within...
Chapter 2 Vectors for Gene Cloning: Plasmids and Bacteriophages Chapter contents CHAPTER CONTENTS 2.1 Plasmids 2.2 Bacteriophages A DNA molecule needs to display several features to be able to act as a vector for gene cloning. Most importantly, it must be able to replicate within the host cell, so that numerous copies of the recombinant DNA molecule can be produced and passed to the daughter cells. A cloning vector also needs to be relatively small, ideally less than 10 kb in size, as large molecules tend to break down during purification, and are also more difficult to manipulate. Two kinds of DNA molecule that satisfy these criteria can be found in bacterial cells, namely plasmids and bacteriophage chromosomes. 2.1 Plasmids Plasmids are circular molecules of DNA that lead an independent existence in the bac- terial cell (Figure 2.1). Plasmids almost always carry one or more genes, and often these genes are responsible for a useful characteristic displayed by the host bacterium. For example, the ability to survive in normally toxic concentrations of antibiotics such as chloramphenicol or ampicillin is often due to the presence in the bacterium of a plasmid carrying antibiotic resistance genes. In the laboratory, antibiotic resistance is often used as a selectable marker to ensure that bacteria in a culture contain a particular plasmid (Figure 2.2). Most plasmids possess at least one DNA sequence that can act as an origin of repli- cation, so they are able to multiply within the cell independently of the main bacterial Gene Cloning and DNA Analysis: An Introduction, Seventh Edition. T.A. Brown. © 2016 John Wiley & Sons, Ltd. Published 2016 by John Wiley & Sons, Ltd. 13 Companion Website: www.wiley.com/go/brown/cloning 14 Part I The Basic Principles of Gene Cloning and DNA Analysis Figure 2.1 Plasmids Plasmids Plasmids: independent genetic elements found in bacterial cells. Bacterial chromosome chromosome (Figure 2.3a). The smaller plasmids make use of the host cell’s own DNA replicative enzymes in order to make copies of themselves, whereas some of the larger ones carry genes that code for special enzymes that are specific for plasmid replication. A few types of plasmid are also able to replicate by inserting themselves into the bac- terial chromosome (Figure 2.3b). These integrative plasmids or episomes may be stably maintained in this form through numerous cell divisions, but they always at some stage exist as independent elements. 2.1.1 Size and copy number The size and copy number of a plasmid are particularly important as far as cloning is concerned. We have already mentioned the relevance of plasmid size, and stated that less Figure 2.2 Ampicillin The use of antibiotic resistance as a selectable marker resistance for a plasmid. RP4 (top) carries genes for resistance to RP4 ampicillin, tetracycline, and kanamycin. Only those E. coli cells that contain RP4 (or a related plasmid) are Kanamycin Tetracycline able to survive and grow in a medium that contains resistance resistance toxic amounts of one or more of these antibiotics. Cell with plasmid Cell without E. coli cells, some plasmid containing RP4 Normal growth Growth medium medium – +50 µg/ml no antibiotic tetracycline All cells Only cells containing can grow RP4 can grow Chapter 2 Vectors for Gene Cloning: Plasmids and Bacteriophages 15 (a) Non-integrative plasmid Plasmids Cell division Bacterial chromosome (b) Episome Plasmid Cell division Bacterial chromosome Chromosome carrying integrated plasmid Figure 2.3 Replication strategies for (a) a non-integrative plasmid, and (b) an episome. than 10 kb is desirable for a cloning vector. Plasmids range from about 1.0 kb for the smallest to over 250 kb for the largest plasmids (Table 2.1), so only a few are useful for cloning purposes. However, as we will see in Chapter 7, larger plasmids can be adapted for cloning under some circumstances. The copy number refers to the number of molecules of an individual plasmid that are normally found in a single bacterial cell. The factors that control copy number are not well understood. Some plasmids, especially the larger ones, are stringent and have a low copy number of perhaps just one or two per cell; others, called relaxed plasmids, are present in multiple copies of 50 or more per cell. Generally speaking, a useful cloning vector needs to be present in the cell in multiple copies so that large quantities of the recombinant DNA molecule can be obtained. Table 2.1 Sizes of representative plasmids. SIZE NUCLEOTIDE MOLECULAR PLASMID LENGTH (kb) MASS (MDa) ORGANISM pUC8 2.1 1.8 E. coli ColE1 6.4 4.2 E. coli RP4 54 36 Pseudomonas and others F 95 63 E. coli TOL 117 78 Pseudomonas putida pTiAch5 213 142 Agrobacterium tumefaciens 16 Part I The Basic Principles of Gene Cloning and DNA Analysis Figure 2.4 Donor cell Conjugative Recipient cell plasmid Plasmid transfer by conjugation between bacterial cells. The donor and recipient cells attach to each other by a pilus, a hollow appendage present on the surface of the donor cell. A copy of the plasmid is then passed to the recipient cell. Transfer is thought to occur through the pilus, but this has not been proven and transfer by some other means (e.g., directly across the bacterial cell walls) remains a possibility. Pilus 2.1.2 Conjugation and compatibility Plasmids fall into two groups: conjugative and non-conjugative. Conjugative plasmids are characterized by the ability to promote sexual conjugation between bacterial cells (Figure 2.4), a process that can result in a conjugative plasmid spreading from one cell to all the other cells in a bacterial culture. Conjugation and plasmid transfer are controlled by a set of transfer or tra genes, which are present on conjugative plasmids but absent from the non-conjugative type. A non-conjugative plasmid may, under some circumstances, be cotransferred along with a conjugative plasmid when both are present in the same cell. Several different kinds of plasmid may be found in a single cell, including more than one different conjugative plasmid at any one time. In fact, cells of E. coli have been known to contain up to seven different plasmids at once. To be able to coexist in the same cell, different plasmids must be compatible. If two plasmids are incompatible, then one or the other will be rapidly lost from the cell. Different types of plasmid can therefore be assigned to different incompatibility groups on the basis of whether or not they can coexist, and plasmids from a single incompatibility group are often related to each other in various ways. The basis of incompatibility is not well understood, but events during plasmid replication are thought to underlie the phenomenon. 2.1.3 Plasmid classification The most useful classification of naturally occurring plasmids is based on the main characteristic coded by the plasmid genes. The five major types of plasmid according to this classification are as follows: r Fertility or F plasmids carry only tra genes and have no characteristic beyond the ability to promote conjugal transfer of plasmids. A well-known example is the F plasmid of E. coli. r Resistance or R plasmids carry genes conferring on the host bacterium resistance to one or more antibacterial agents, such as chloramphenicol, ampicillin, and mercury. R plasmids are very important in clinical microbiology as their spread through Chapter 2 Vectors for Gene Cloning: Plasmids and Bacteriophages 17 natural populations can have profound consequences in the treatment of bacterial infections. An example is RP4, which is commonly found in Pseudomonas, but also occurs in many other bacteria. r Col plasmids code for colicins, proteins that kill other bacteria. An example is ColE1 of E. coli. r Degradative plasmids allow the host bacterium to metabolize unusual molecules such as toluene and salicylic acid, an example being TOL of Pseudomonas putida. r Virulence plasmids confer pathogenicity on the host bacterium; these include the Ti plasmids of Agrobacterium tumefaciens, which induce crown gall disease on dicotyledonous plants. 2.1.4 Plasmids in organisms other than bacteria Although plasmids are widespread in bacteria they are by no means as common in other organisms. The best-characterized eukaryotic plasmid is the 2 μm circle that occurs in many strains of the yeast Saccharomyces cerevisiae. The discovery of the 2 μm plasmid was very fortuitous as it allowed the construction of cloning vectors for this very impor- tant industrial organism (p. 111). The search for plasmids in other eukaryotes (such as filamentous fungi, plants and animals) has proved disappointing, and it is suspected that many higher organisms simply do not harbour plasmids within their cells. 2.2 Bacteriophages Bacteriophages, or phages as they are commonly known, are viruses that specifically infect bacteria. Like all viruses, phages are very simple in structure, consisting merely of a DNA (or occasionally ribonucleic acid (RNA)) molecule carrying a number of genes, including several for replication of the phage, surrounded by a protective coat or capsid made up of protein molecules (Figure 2.5). (a) Head-and-tail (b) Filamentous Figure 2.5 The two main types of phage structure. (a) Head-and- Head Protein tail (e.g., λ). (b) Filamentous (e.g., M13). (contains molecules DNA) (capsid) DNA molecule Tail 18 Part I The Basic Principles of Gene Cloning and DNA Analysis Phage particle DNA 1 The phage attaches to the bacterium and injects its DNA Phage DNA molecule 2 The phage DNA molecule is replicated 3 Capsid components are synthesized, new phage particles are assembled and released Capsid components Cell lysis New phage particle Figure 2.6 The general pattern of infection of a bacterial cell by a bacteriophage. 2.2.1 The phage infection cycle The general pattern of infection, which is the same for all types of phage, is a three-step process (Figure 2.6): 1 The phage particle attaches to the outside of the bacterium and injects its DNA chromosome into the cell. 2 The phage DNA molecule is replicated, usually by specific phage enzymes coded by genes in the phage chromosome. 3 Other phage genes direct synthesis of the protein components of the capsid, and new phage particles are assembled and released from the bacterium. With some phage types the entire infection cycle is completed very quickly, possibly in less than 20 minutes. This type of rapid infection is called a lytic cycle, as release of the new phage particles is associated with lysis of the bacterial cell. The characteristic feature of a lytic infection cycle is that phage DNA replication is immediately followed Chapter 2 Vectors for Gene Cloning: Plasmids and Bacteriophages 19 by synthesis of capsid proteins, and the phage DNA molecule is never maintained in a stable condition in the host cell. 2.2.2 Lysogenic phages In contrast to a lytic cycle, lysogenic infection is characterized by retention of the phage DNA molecule in the host bacterium, possibly for many thousands of cell divisions. With many lysogenic phages the phage DNA is inserted into the bacterial genome, in a manner similar to episomal insertion (see Figure 2.3b). The integrated form of the phage DNA (called the prophage) is quiescent, and a bacterium (referred to as a lysogen) that carries a prophage is usually physiologically indistinguishable from an uninfected cell. The prophage is eventually released from the host genome and the phage reverts to the lytic mode and lyses the cell. The infection cycle of lambda (λ), a typical lysogenic phage of this type, is shown in Figure 2.7. A limited number of lysogenic phages follow a rather different infection cycle. When M13 or a related phage infects E. coli, new phage particles are continuously assem- bled and released from the cell. The M13 DNA is not integrated into the bacterial genome and does not become quiescent. With these phages, cell lysis never occurs, and the infected bacterium can continue to grow and divide, albeit at a slower rate than uninfected cells. Figure 2.8 shows the M13 infection cycle. Although there are many different varieties of bacteriophage, only λ and M13 have found a major role as cloning vectors. We will now consider the properties of these two phages in more detail. Gene organization in the 𝛌 DNA molecule Lambda is a typical example of a head-and-tail phage (see Figure 2.5a). The DNA is contained in the polyhedral head structure and the tail serves to attach the phage to the bacterial surface and to inject the DNA into the cell (see Figure 2.7). The λ DNA molecule is 49 kb in size and has been intensively studied by the tech- niques of gene mapping and DNA sequencing. As a result, the positions and identities of all of the genes in the λ DNA molecule are known (Figure 2.9). A feature of the λ genetic map is that genes related in terms of function are clustered together in the genome. For example, all of the genes coding for components of the capsid are grouped together in the left-hand third of the molecule, and genes controlling integration of the prophage into the host genome are clustered in the middle of the molecule. Clustering of related genes is profoundly important for controlling expression of the λ genome, as it allows genes to be switched on and off as a group rather than individually. Clustering is also important in the construction of λ-based cloning vectors, as we will discover when we return to this topic in Chapter 6. The linear and circular forms of 𝛌 DNA A second feature of λ that turns out to be of importance in the construction of cloning vectors is the conformation of the DNA molecule. The molecule shown in Figure 2.9 is linear, with two free ends, and represents the DNA present in the phage head structure. This linear molecule consists of two complementary strands of DNA, base-paired according to the Watson–Crick rules (that is, double-stranded DNA). At either end of the molecule is a short 12-nucleotide stretch in which the DNA is single-stranded (Figure 2.10a); these two single strands are complementary and so can 20 Part I The Basic Principles of Gene Cloning and DNA Analysis λ phage particle attaches to an E.coli cell and injects its DNA Bacterial chromosome λ DNA circularizes λ DNA λ DNA integrates into the host chromosome Cell Cell division division A Induction: A λ DNA excises from the host chromosome B New phage particles are produced (see steps 2 and 3 of figure 2.6) B Figure 2.7 The lysogenic infection cycle of bacteriophage λ. base pair with one another to form a circular, completely double-stranded molecule (Figure 2.10b). Complementary single strands are often referred to as ‘sticky’ ends or cohesive ends, because base pairing between them can ‘stick’ together the two ends of a DNA molecule (or the ends of two different DNA molecules). The λ cohesive ends are called the cos Chapter 2 Vectors for Gene Cloning: Plasmids and Bacteriophages 21 M13 phage Pilus M13 DNA M13 phage attaches to a pilus on an E.coli cell and injects its DNA M13 DNA replication New M13 phages are continuously extruded M13 phages from an infected cell Infected cells continue to grow and divide Daughter cells continue to release M13 particles Figure 2.8 The infection cycle of bacteriophage M13. sites and they play two distinct roles during the λ infection cycle. First, they allow the linear DNA molecule that is injected into the cell to be circularized, which is a necessary prerequisite for insertion into the bacterial genome (see Figure 2.7). The second role of the cos sites is rather different, and comes into play after the prophage has excised from the host genome. At this stage a large number of new λ DNA molecules are produced by the rolling circle mechanism of replication (Figure 2.10c), in which a continuous DNA strand is ‘rolled off’ the template molecule. The result is a catenane consisting of a series of linear λ genomes joined together at the cos sites. The role of the cos sites is now to act as recognition sequences for an endonuclease that cleaves the catenane at the cos sites, producing individual λ genomes. This endonucle- ase, which is the product of gene A on the DNA molecule, creates the single-stranded sticky ends, and also acts in conjunction with other proteins to package each λ genome into a phage head structure. The cleavage and packaging processes recognize just the Lysis of the host Early regulation Late regulation DNA synthesis and excision Integration Capsid components b2 region and assembly (non-essential) AWB C D EFZUVGT H M LKI J int xis exo clll N cl cro OP Q SR 0 2 4 6 8 10 12 14 16 18 20 22 24 26 28 30 32 34 36 38 40 42 44 46 48 49kb Figure 2.9 The λ genetic map, showing the positions of the important genes and the functions of the gene clusters. 22 Part I The Basic Principles of Gene Cloning and DNA Analysis (a) The linear form of the λ DNA molecule Right cohesive end C C C GC C G C T G GA GG G C GG CG A CC T Left cohesive end (b) The circular form of cos site the λ DNA molecule CC G TG CG G A C C GGC AC C GC T GG (c) Replication and packaging of λ DNA cos cos cos Catenane ‘rolled off’ cos the λ DNA molecule 3 2 1 The gene A endonuclease cleaves the catenane at the cos sites Protein components of the capsid New phage particles are assembled Figure 2.10 The linear and circular forms of λ DNA. (a) The linear form, showing the left and right cohesive ends. (b) Base pairing between the cohesive ends results in the circular form of the molecule. (c) Rolling circle replication produces a catenane of new linear λ DNA molecules, which are individually packaged into phage heads as new λ particles are assembled. cos sites and the DNA sequences to either side of them, so changing the structure of the internal regions of the λ genome, for example by inserting new genes, has no effect on these events so long as the overall length of the λ genome is not altered too greatly. M13 – a filamentous phage M13 is an example of a filamentous phage (see Figure 2.5b) and is completely different in structure from λ. Furthermore, the M13 DNA molecule is much smaller than the λ Chapter 2 Vectors for Gene Cloning: Plasmids and Bacteriophages 23 M13 particle Figure 2.11 injects DNA The M13 infection cycle, showing the different into cell types of DNA replication that occur. (a) After Pilus infection, the single-stranded M13 DNA molecule (a) Injection of single- is converted into the double-stranded replicative stranded DNA into form (RF). (b) The RF replicates to produce the host cell, followed multiple copies of itself. (c) Single-stranded by synthesis of the second strand molecules are synthesized by rolling circle replication and used in the assembly of new M13 Single-stranded Double-stranded DNA DNA - replicative particles. form (RF) (b) Replication of the RF to produce new double-stranded molecules (c) Mature M13 phage are continuously produced RF replicates by rolling circle mechanism to produce linear single-stranded DNA Circularized DNA Mature phage particles genome, being only 6407 nucleotides in length. It is circular and is unusual in that it consists entirely of single-stranded DNA. The smaller size of the M13 DNA molecule means that it has room for fewer genes than the λ genome. This is possible because the M13 capsid is constructed from multiple copies of just three proteins (requiring only three genes), whereas synthesis of the λ head-and-tail structure involves over 15 different proteins. In addition, M13 follows a simpler infection cycle than λ, and does not need genes for insertion into the host genome. Injection of an M13 DNA molecule into an E. coli cell occurs via the pilus, the struc- ture that connects two cells during sexual conjugation (see Figure 2.4). Once inside the cell the single-stranded molecule acts as the template for synthesis of a complemen- tary strand, resulting in normal double-stranded DNA (Figure 2.11a). This molecule is not inserted into the bacterial genome, but instead replicates until over 100 copies are present in the cell (Figure 2.11b). When the bacterium divides, each daughter cell receives copies of the phage genome, which continues to replicate, thereby maintaining its overall numbers per cell. As shown in Figure 2.11c, new phage particles are continu- ously assembled and released, with about 1000 new phages being produced during each generation of an infected cell. Several features of M13 make this phage attractive as a cloning vector. The genome is less than 10 kb in size, well within the range desirable for a potential vector. In addition, the double-stranded replicative form (RF) of the M13 genome behaves very much like a plasmid, and can be treated as such for experimental purposes. It is easily prepared from a culture of infected E. coli cells (p. 43) and can be reintroduced by transfection (p. 83). Most importantly, genes cloned with an M13-based vector can be obtained in 24 Part I The Basic Principles of Gene Cloning and DNA Analysis the form of single-stranded DNA. Single-stranded versions of cloned genes are useful for several techniques, such as in vitro mutagenesis (p. 216). Cloning in an M13 vector is an easy and reliable way of obtaining single-stranded DNA for this type of work. M13 vectors are also used in phage display, a technique for identifying pairs of genes whose protein products interact with one another (p. 241). 2.2.3 Viruses as cloning vectors for other organisms Most living organisms are infected by viruses, and it is not surprising that there has been great interest in the possibility that viruses might be used as cloning vectors for higher organisms. This is especially important when it is remembered that plasmids are not commonly found in organisms other than bacteria and yeast. Several eukaryotic viruses have been employed as cloning vectors for specialized applications. For example, human adenoviruses are used in gene therapy (p. 286), baculoviruses are used to synthe- size important pharmaceutical proteins in insect cells (p. 262), and caulimoviruses and geminiviruses have been used for cloning in plants (p. 127). These vectors are discussed more fully in Chapter 7. Further reading FURTHER READING Dale, J.W. and Park, S.T. (2010) Molecular Genetics of Bacteria, 5th edn, Wiley-Blackwell, Chichester. [Provides a detailed description of plasmids and bacteriophages.] Willey, J., Sherwood, L., and Woolverton, C. (2013) Prescott’s Microbiology, 9th edn, McGraw-Hill Higher Education, Maidenhead. [A good introduction to microbiology, including plasmids and phages.]