Chapter 3: Purification of DNA from Living Cells PDF
Document Details
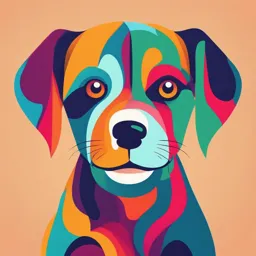
Uploaded by PremierGroup7135
T.A. Brown
Tags
Summary
Chapter 3 of 'Gene Cloning and DNA Analysis' describes various methods for purifying DNA from living cells, including total cell DNA, plasmid DNA, and bacteriophage DNA. It details the process of preparing bacterial cultures, types of growth media and the importance of considering the specific characteristics of various microorganisms.
Full Transcript
Chapter 3 Purification of DNA from Living Cells Chapter contents CHAPTER CONTENTS 3.1 Preparation of total cell DNA 3.2 Preparation of plasmid DNA 3.3 Preparation of bacteriophage DNA The genetic engineer will, at different times, need to prepare at least three distinct kinds o...
Chapter 3 Purification of DNA from Living Cells Chapter contents CHAPTER CONTENTS 3.1 Preparation of total cell DNA 3.2 Preparation of plasmid DNA 3.3 Preparation of bacteriophage DNA The genetic engineer will, at different times, need to prepare at least three distinct kinds of DNA. First, total cell DNA will often be required as a source of material from which to obtain genes to be cloned. Total cell DNA may be DNA from a culture of bacteria, from a plant, from animal cells, or from any other type of organism that is being studied. It consists of the genomic DNA of the organism along with any additional DNA mole- cules, such as plasmids, that are present. The second type of DNA that will be required is pure plasmid DNA. Preparation of plasmid DNA from a culture of bacteria follows the same basic steps as purification of total cell DNA, with the crucial difference that at some stage the plasmid DNA must be separated from the main bulk of chromosomal DNA also present in the cell. Finally, phage DNA will be needed if a phage cloning vector is to be used. Phage DNA is generally prepared from bacteriophage particles rather than from infected cells, so there is no problem with contaminating bacterial DNA. However, special techniques are needed to remove the phage capsid. An exception is the double-stranded replicative form of M13, which is prepared from E. coli cells in the same way as a bacterial plasmid. 3.1 Preparation of total cell DNA The fundamentals of DNA preparation are most easily understood by first considering the simplest type of DNA purification procedure, that where the entire DNA complement Gene Cloning and DNA Analysis: An Introduction. 6th edition. By T.A. Brown. Published 2010 by 25 Blackwell Publishing. 26 Part I The Basic Principles of Gene Cloning and DNA Analysis Centrifugation Bacterial culture Pellet of cells Cell extract Pure DNA 1 2 3 4 A culture of bacteria is The cells are removed and The DNA is purified The DNA is grown and then harvested broken to give a cell extract from the cell extract concentrated Figure 3.1 The basic steps in preparation of total cell DNA from a culture of bacteria. of a bacterial cell is required. The modifications needed for plasmid and phage DNA preparation can then be described later. The procedure for total DNA preparation from a culture of bacterial cells can be divided into four stages (Figure 3.1): 1 A culture of bacteria is grown and then harvested. 2 The cells are broken open to release their contents. 3 This cell extract is treated to remove all components except the DNA. 4 The resulting DNA solution is concentrated. 3.1.1 Growing and harvesting a bacterial culture Most bacteria can be grown without too much difficulty in a liquid medium (broth culture). The culture medium must provide a balanced mixture of the essential nutrients at concentrations that will allow the bacteria to grow and divide efficiently. Two typical growth media are detailed in Table 3.1. M9 is an example of a defined medium in which all the components are known. This medium contains a mixture of inorganic nutrients to provide essential elements such as Table 3.1 The composition of two typical media for the growth of bacterial cultures. MEDIUM COMPONENT g/l OF MEDIUM M9 medium Na2HPO4 6.0 KH2PO4 3.0 NaCl 0.5 NH4Cl 1.0 MgSO4 0.5 Glucose 2.0 CaCl2 0.015 LB (Luria-Bertani medium) Tryptone 10.0 Yeast extract 5.0 NaCl 10.0 Chapter 3 Purification of DNA from Living Cells 27 nitrogen, magnesium, and calcium, as well as glucose to supply carbon and energy. In practice, additional growth factors such as trace elements and vitamins must be added to M9 before it will support bacterial growth. Precisely which supplements are needed depends on the species concerned. The second medium described in Table 3.1 is rather different. Luria-Bertani (LB) is a complex or undefined medium, meaning that the precise identity and quantity of its components are not known. This is because two of the ingredients, tryptone and yeast extract, are complicated mixtures of unknown chemical compounds. Tryptone in fact supplies amino acids and small peptides, while yeast extract (a dried preparation of partially digested yeast cells) provides the nitrogen requirements, along with sugars and inorganic and organic nutrients. Complex media such as LB need no further supple- mentation and support the growth of a wide range of bacterial species. Defined media must be used when the bacterial culture has to be grown under precisely controlled conditions. However, this is not necessary when the culture is being grown simply as a source of DNA, and under these circumstances a complex medium is appropriate. In LB medium at 37°C, aerated by shaking at 150–250 rpm on a rotary platform, E. coli cells divide once every 20 min or so until the culture reaches a maximum density of about 2–3 × 109 cells /ml. The growth of the culture can be mon- itored by reading the optical density (OD) at 600 nm (Figure 3.2), at which wavelength 1 OD unit corresponds to about 0.8 × 109 cells /ml. In order to prepare a cell extract, the bacteria must be obtained in as small a volume as possible. Harvesting is therefore performed by spinning the culture in a centrifuge (Figure 3.3). Fairly low centrifugation speeds will pellet the bacteria at the bottom of the centrifuge tube, allowing the culture medium to be poured off. Bacteria from a (a) Measurement of optical density Figure 3.2 Estimation of bacterial cell number by Incident light measurement of optical density (OD). (a) A at 600 nm Transmitted OD sample of the culture is placed in a glass cuvette wavelength light and light with a wavelength of 600 nm shone through. The amount of light that passes Detector through the culture is measured and the OD (also called the absorbance) calculated as: Bacterial culture contained intensity of transmitted light 1 OD unit = log10 in a 1 cm wide cuvette intensity of incident light The operation is performed with a (b) Estimation of cell number spectrophotometer. (b) The cell number from a calibration curve corresponding to the OD reading is calculated 2.5 from a calibration curve. This curve is plotted from the OD values of a series of cultures Optical density at 600 nm 2.0 of known cell density. For E. coli, 1 OD unit = 0.8 × 109 cells/ml. 1.5 1.0 0.5 0 0.4 0.8 1.2 1.6 2.0 Cell number (× 109) per ml 28 Part I The Basic Principles of Gene Cloning and DNA Analysis Spin at 8000 rpm for 10 minutes Bacterial culture Pellet of bacteria Centrifuge rotor Figure 3.3 Harvesting bacteria by centrifugation. 1000 ml culture at maximum cell density can then be resuspended into a volume of 10 ml or less. 3.1.2 Preparation of a cell extract The bacterial cell is enclosed in a cytoplasmic membrane and surrounded by a rigid cell wall. With some species, including E. coli, the cell wall may itself be enveloped by a second, outer membrane. All of these barriers have to be disrupted to release the cell components. Techniques for breaking open bacterial cells can be divided into physical methods, in which the cells are disrupted by mechanical forces, and chemical methods, where cell lysis is brought about by exposure to chemical agents that affect the integrity of the cell barriers. Chemical methods are most commonly used with bacterial cells when the object is DNA preparation. Chemical lysis generally involves one agent attacking the cell wall and another disrupting the cell membrane (Figure 3.4a). The chemicals that are used depend on the species of bacterium involved, but with E. coli and related organisms, weakening of the cell wall is usually brought about by lysozyme, ethylenediamine tetraacetate (EDTA), or a combination of both. Lysozyme is an enzyme that is present in egg white and in secretions such as tears and saliva, and which digests the polymeric compounds that give the cell wall its rigidity. EDTA removes magnesium ions that are essential for pre- serving the overall structure of the cell envelope, and also inhibits cellular enzymes that could degrade DNA. Under some conditions, weakening the cell wall with lysozyme or (a) Cell lysis (b) Centrifugation to remove cell debris Disrupt cell wall Cell extract Cell extract Disrupt cell Centrifuge DNA, RNA, membrane protein Cell debris Figure 3.4 Preparation of a cell extract. (a) Cell lysis. (b) Centrifugation of the cell extract to remove insoluble debris. Chapter 3 Purification of DNA from Living Cells 29 EDTA is sufficient to cause bacterial cells to burst, but usually a detergent such as sodium dodecyl sulphate (SDS) is also added. Detergents aid the process of lysis by removing lipid molecules and thereby cause disruption of the cell membranes. Having lysed the cells, the final step in preparation of a cell extract is removal of insoluble cell debris. Components such as partially digested cell wall fractions can be pelleted by centrifugation (Figure 3.4b), leaving the cell extract as a reasonably clear supernatant. 3.1.3 Purification of DNA from a cell extract In addition to DNA, a bacterial cell extract contains significant quantities of protein and RNA. A variety of methods can be used to purify the DNA from this mixture. One approach is to treat the mixture with reagents which degrade the contaminants, leaving a pure solution of DNA (Figure 3.5a). Other methods use ion-exchange chromatography to separate the mixture into its various components, so the DNA is removed from the proteins and RNA in the extract (Figure 3.5b). Removing contaminants by organic extraction and enzyme digestion The standard way to deproteinize a cell extract is to add phenol or a 1 : 1 mixture of phenol and chloroform. These organic solvents precipitate proteins but leave the nucleic acids (DNA and RNA) in aqueous solution. The result is that if the cell extract is mixed gently with the solvent, and the layers then separated by centrifugation, precipitated protein molecules are left as a white coagulated mass at the interface between the aqueous and organic layers (Figure 3.6). The aqueous solution of nucleic acids can then be removed with a pipette. With some cell extracts the protein content is so great that a single phenol extraction is not sufficient to completely purify the nucleic acids. This problem could be solved by carrying out several phenol extractions one after the other, but this is undesirable as each mixing and centrifugation step results in a certain amount of breakage of the DNA molecules. The answer is to treat the cell extract with a protease such as pronase or (a) Degradation of contaminants Figure 3.5 Two approaches to DNA purification. (a) Treating the mixture with reagents which degrade the contaminants, leaving a pure solution of DNA. (b) Separating the mixture into different fractions, one of which is pure DNA. DNA Contaminants Pure DNA (b) Separation of DNA from contaminants + Pure DNA Contaminants 30 Part I The Basic Principles of Gene Cloning and DNA Analysis Figure 3.6 Aqueous layer Removal of protein contaminants by phenol (DNA + RNA) extraction. Interface (coagulated proteins) Phenol Cell extract Mix with Separate layers phenol by centrifugation proteinase K before phenol extraction. These enzymes break polypeptides down into smaller units, which are more easily removed by phenol. Some RNA molecules, especially messenger RNA (mRNA), are removed by phenol treatment, but most remain with the DNA in the aqueous layer. The only effective way to remove the RNA is with the enzyme ribonuclease, which rapidly degrades these molecules into ribonucleotide subunits. Using ion-exchange chromatography to purify DNA from a cell extract Biochemists have devised various methods for using differences in electrical charge to separate mixtures of chemicals into their individual components. One of these methods is ion-exchange chromatography, which separates molecules according to how tightly they bind to electrically charged particles present in a chromatographic matrix or resin. DNA and RNA are both negatively charged, as are some proteins, and so bind to a positively charged resin. The electrical attachment is disrupted by salt (Figure 3.7a), removal of the more tightly bound molecules requiring higher concentrations of salt. By gradually increasing the salt concentration, different types of molecule can be detached from the resin one after another. The simplest way to carry out ion-exchange chromatography is to place the resin in a glass or plastic column and then add the cell extract to the top (Figure 3.7b). The extract passes through the column, and because this extract contains very little salt all the negatively charged molecules bind to the resin and are retained in the column. If a salt solution of gradually increasing concentration is now passed through the column, the different types of molecule will elute (i.e., become unbound) in the sequence protein, RNA, and finally DNA. However, such careful separation is usually not needed so just two salt solutions are used, one whose concentration is sufficient to elute the protein and RNA, leaving just the DNA bound, followed by a second of a higher concentration which elutes the DNA, now free from protein and RNA contaminants. 3.1.4 Concentration of DNA samples Organic extraction often results in a very thick solution of DNA that does not need to be concentrated any further. Other purification methods give more dilute solutions and it is therefore important to consider methods for increasing the DNA concentration. The most frequently used method of concentration is ethanol precipitation. In the presence of salt (strictly speaking, monovalent cations such as sodium ions (Na+ )), and at a temperature of −20°C or less, absolute ethanol efficiently precipitates polymeric nucleic acids. With a thick solution of DNA the ethanol can be layered on top of the sample, causing molecules to precipitate at the interface. A spectacular trick is to push a glass rod through the ethanol into the DNA solution. When the rod is removed, Chapter 3 Purification of DNA from Living Cells 31 (a) Attachment of DNA to ion-exchange particles Figure 3.7 DNA purification by ion-exchange + + + chromatography. (a) Attachment of DNA to ion- + exchange particles. (b) DNA is purified by column + Salt + + chromatography. The solutions passing through + the column can be collected by gravity flow or by + the spin column method, in which the column is + placed in a low-speed centrifuge. lon-exchange DNA particle molecule (b) DNA purification by ion-exchange chromatography Cell extract More Salt salt lon-exchange resin Protein DNA + RNA Discard DNA molecules adhere and can be pulled out of the solution in the form of a long fiber (Figure 3.8a). Alternatively, if ethanol is mixed with a dilute DNA solution, the precipit- ate can be collected by centrifugation (Figure 3.8b), and then redissolved in an appropriate volume of water. Ethanol precipitation has the added advantage of leaving short-chain and monomeric nucleic acid components in solution. Ribonucleotides produced by ribonuclease treatment are therefore lost at this stage. 3.1.5 Measurement of DNA concentration It is crucial to know exactly how much DNA is present in a solution when carrying out a gene cloning experiment. Fortunately DNA concentrations can be accurately measured by ultraviolet (UV) absorbance spectrophotometry. The amount of UV radiation absorbed by a solution of DNA is directly proportional to the amount of DNA in the 32 Part I The Basic Principles of Gene Cloning and DNA Analysis Figure 3.8 Collecting DNA by ethanol precipitation. (a) Absolute ethanol is layered on top of a Glass rod concentrated solution of DNA. Fibers of DNA can be withdrawn with a glass rod. (b) For less concentrated solutions ethanol is added (at a ratio of 2.5 volumes of absolute ethanol to 1 volume of DNA fibers DNA solution) and precipitated DNA collected by centrifugation. Ethanol Ethanol Concentrated Precipitated DNA solution DNA, collected by centrifugation (a) (b) sample. Usually absorbance is measured at 260 nm, at which wavelength an absorbance (A 260) of 1.0 corresponds to 50 mg of double-stranded DNA per ml. Measurements of as little as 1 fl of a DNA solution can be carried out in spectrophotometers designed especially for this purpose. Ultraviolet absorbance can also be used to check the purity of a DNA preparation. With a pure sample of DNA, the ratio of the absorbances at 260 and 280 nm (A 260 /A 280) is 1.8. Ratios of less than 1.8 indicate that the preparation is contaminated, either with protein or with phenol. 3.1.6 Other methods for the preparation of total cell DNA Bacteria are not the only organisms from which DNA may be required. Total cell DNA from, for example, plants or animals will be needed if the aim of the genetic engineering project is to clone genes from these organisms. Although the basic steps in DNA purification are the same whatever the organism, some modifications may have to be introduced to take account of the special features of the cells being used. Obviously growth of cells in liquid medium is appropriate only for bacteria, other microorganisms, and plant and animal cell cultures. The major modifications, however, are likely to be needed at the cell breakage stage. The chemicals used for disrupting bacterial cells do not usually work with other organisms: lysozyme, for example, has no effect on plant cells. Specific degradative enzymes are available for most cell wall types, but often physical techniques, such as grinding frozen material with a mortar and pestle, are more efficient. On the other hand, most animal cells have no cell wall at all, and can be lysed simply by treating with detergent. Another important consideration is the biochemical content of the cells from which DNA is being extracted. With most bacteria the main biochemicals present in a cell extract are protein, DNA and RNA, so phenol extraction and/or protease treatment, followed by removal of RNA with ribonuclease, leaves a pure DNA sample. These treatments may not, however, be sufficient to give pure DNA if the cells also contain significant quantities of other biochemicals. Plant tissues are particularly difficult in this respect as they often contain large amounts of carbohydrates that are not removed by phenol extraction. Instead a different approach must be used. One method makes use of a detergent called cetyltrimethylammonium bromide (CTAB), which forms an insoluble complex with nucleic acids. When CTAB is added to a plant cell extract the Chapter 3 Purification of DNA from Living Cells 33 Carbohydrates, proteins, etc. Discard Ethanol CTAB Centrifuge supernatant precipitation, ribonuclease Resuspend pellet Pure treatment in 1 M NaCl DNA Cell extract Precipitated nucleic Nucleic acid– acid–CTAB complex CTAB complex Figure 3.9 The CTAB method for purification of plant DNA. nucleic acid–CTAB complex precipitates, leaving carbohydrate, protein, and other contaminants in the supernatant (Figure 3.9). The precipitate is then collected by centrifugation and resuspended in 1 M sodium chloride, which causes the complex to break down. The nucleic acids can now be concentrated by ethanol precipitation and the RNA removed by ribonuclease treatment. The need to adapt organic extraction methods to take account of the biochemical contents of different types of starting material has stimulated the search for DNA purification methods that can be used with any species. This is one of the reasons why ion-exchange chromatography has become so popular. A similar method involves a compound called guanidinium thiocyanate, which has two properties that make it useful for DNA purification. First, it denatures and dissolves all biochemicals other than nucleic acids and can therefore be used to release DNA from virtually any type of cell or tissue. Second, in the presence of guanidinium thiocyanate, DNA binds tightly to silica particles (Figure 3.10a). This provides an easy way of recovering the DNA from the denatured mix of biochemicals. One possibility is to add the silica directly to the cell extract but, as with the ion-exchange methods, it is more convenient to use a chromatography column. The silica is placed in the column and the cell extract added (Figure 3.10b). DNA binds to the silica and is retained in the column, whereas the denatured biochemicals pass straight through. After washing away the last contaminants with guanidinium thiocyanate solution, the DNA is recovered by adding water, which destabilizes the interactions between the DNA molecules and the silica. 3.2 Preparation of plasmid DNA Purification of plasmids from a culture of bacteria involves the same general strategy as preparation of total cell DNA. A culture of cells, containing plasmids, is grown in liquid medium, harvested, and a cell extract prepared. The protein and RNA are removed, and the DNA probably concentrated by ethanol precipitation. However, there is an important distinction between plasmid purification and preparation of total cell DNA. In a plasmid preparation it is always necessary to separate the plasmid DNA from the large amount of bacterial chromosomal DNA that is also present in the cells. Separating the two types of DNA can be very difficult, but is nonetheless essential if the plasmids are to be used as cloning vectors. The presence of the smallest amount of contaminating bacterial DNA in a gene cloning experiment can easily lead to undesirable results. Fortunately several methods are available for removal of bacterial DNA during 34 Part I The Basic Principles of Gene Cloning and DNA Analysis Figure 3.10 (a) Attachment of DNA to silica particles DNA purification by the guanidinium thiocyanate + + + and silica method. (a) In the presence of guanidinium thiocyanate, DNA binds to silica + + Salt + particles. (b) DNA is purified by column + chromatography. + + + Silica DNA particle molecule (b) DNA purification by column chromatography Cell extract Water Silica particles Denatured DNA biochemicals Discard plasmid purification, and the use of these methods, individually or in combination, can result in isolation of very pure plasmid DNA. The methods are based on the several physical differences between plasmid DNA and bacterial DNA, the most obvious of which is size. The largest plasmids are only 8% of the size of the E. coli chromosome, and most are much smaller than this. Techniques that can separate small DNA molecules from large ones should therefore effectively purify plasmid DNA. In addition to size, plasmids and bacterial DNA differ in conformation. When applied to a polymer such as DNA, the term conformation refers to the overall spatial configura- tion of the molecule, with the two simplest conformations being linear and circular. Plasmids and the bacterial chromosome are circular, but during preparation of the cell extract the chromosome is always broken to give linear fragments. A method for separating circular from linear molecules will therefore result in pure plasmids. Chapter 3 Purification of DNA from Living Cells 35 3.2.1 Separation on the basis of size The usual stage at which size fractionation is performed is during preparation of the cell extract. If the cells are lysed under very carefully controlled conditions, only a minimal amount of chromosomal DNA breakage occurs. The resulting DNA fragments are still very large—much larger than the plasmids—and can be removed with the cell debris by centrifugation. This process is aided by the fact that the bacterial chromosome is physically attached to the cell envelope, so fragments of the chromosome sediment with the cell debris if these attachments are not broken. Cell disruption must therefore be carried out very gently to prevent wholesale break- age of the bacterial DNA. For E. coli and related species, controlled lysis is performed as shown in Figure 3.11. Treatment with EDTA and lysozyme is carried out in the presence of sucrose, which prevents the cells from bursting immediately. Instead, sphaeroplasts are formed, cells with partially degraded cell walls that retain an intact cytoplasmic membrane. Cell lysis is now induced by adding a non-ionic detergent such as Triton X-100 (ionic detergents, such as SDS, cause chromosomal breakage). This method causes very little breakage of the bacterial DNA, so centrifugation leaves a cleared lysate, consisting almost entirely of plasmid DNA. A cleared lysate will, however, invariably retain some chromosomal DNA. Further- more, if the plasmids themselves are large molecules, they may also sediment with the Figure 3.11 Bacterial chromosome Plasmids Preparation of a cleared lysate. Lysozyme + EDTA 25% sucrose Intact cell membrane Sphaeroplast Disrupted cell wall Triton X-100 Cell extract Centrifuge Cleared lysate Plasmids Large DNA fragments Cell debris 36 Part I The Basic Principles of Gene Cloning and DNA Analysis Figure 3.12 Two conformations of circular double-stranded DNA: (a) supercoiled—both strands are intact; (b) open-circular Nick —one or both strands are nicked. (a) Supercoiled (b) Open-circular cell debris. Size fractionation is therefore rarely sufficient on its own, and we must consider alternative ways of removing the bacterial DNA contaminants. 3.2.2 Separation on the basis of conformation Before considering the ways in which conformational differences between plasmids and bacterial DNA can be used to separate the two types of DNA, we must look more closely at the overall structure of plasmid DNA. It is not strictly correct to say that plasmids have a circular conformation, because double-stranded DNA circles can take up one of two quite distinct configurations. Most plasmids exist in the cell as supercoiled molecules (Figure 3.12a). Supercoiling occurs because the double helix of the plasmid DNA is partially unwound during the plasmid replication process by enzymes called topoisomerases (p. 69). The supercoiled conformation can be maintained only if both polynucleotide strands are intact, hence the more technical name of covalently closed- circular (ccc) DNA. If one of the polynucleotide strands is broken the double helix reverts to its normal relaxed state, and the plasmid takes on the alternative conforma- tion, called open-circular (oc) (Figure 3.12b). Supercoiling is important in plasmid preparation because supercoiled molecules can be fairly easily separated from non-supercoiled DNA. Two different methods are commonly used. Both can purify plasmid DNA from crude cell extracts, although in practice best results are obtained if a cleared lysate is first prepared. Alkaline denaturation The basis of this technique is that there is a narrow pH range at which non-supercoiled DNA is denatured, whereas supercoiled plasmids are not. If sodium hydroxide is added to a cell extract or cleared lysate, so that the pH is adjusted to 12.0–12.5, then the hydrogen bonding in non-supercoiled DNA molecules is broken, causing the double helix to unwind and the two polynucleotide chains to separate (Figure 3.13). If acid is now added, these denatured bacterial DNA strands reaggregate into a tangled mass. The insoluble network can be pelleted by centrifugation, leaving plasmid DNA in the supernatant. An additional advantage of this procedure is that, under some circum- stances (specifically cell lysis by SDS and neutralization with sodium acetate), most of the protein and RNA also becomes insoluble and can be removed by the centrifugation step. Further purification by organic extraction or column chromatography may there- fore not be needed if the alkaline denaturation method is used. Ethidium bromide–caesium chloride density gradient centrifugation This is a specialized version of the more general technique of equilibrium or density gradient centrifugation. A density gradient is produced by centrifuging a solution of Chapter 3 Purification of DNA from Living Cells 37 pH 12.0 to 12.5 pH 7.0 Centrifuge Supercoiled plasmids Pellet of linear Supercoiled Single-stranded Tangled mass DNA molecules Linear DNA plasmids linear DNA of linear DNA Figure 3.13 Plasmid purification by the alkaline denaturation method. Figure 3.14 1.55 Protein Caesium chloride density gradient centrifugation. (a) A CsCl density gradient produced by high speed centrifugation. (b) Separation of protein, CsCl density (g/cm3) 1.60 DNA, and RNA in a density gradient. Increasing 1.65 CsCl concentration 1.70 DNA 1.75 RNA (a) (b) caesium chloride (CsCl) at a very high speed (Figure 3.14a). Macromolecules present in the CsCl solution when it is centrifuged form bands at distinct points in the gradient (Figure 3.14b). Exactly where a particular molecule bands depends on its buoyant density: DNA has a buoyant density of about 1.70 g /cm3, and therefore migrates to the point in the gradient where the CsCl density is also 1.70 g /cm3. In contrast, protein molecules have much lower buoyant densities, and so float at the top of the tube, whereas RNA forms a pellet at the bottom (Figure 3.14b). Density gradient centrifuga- tion can therefore separate DNA, RNA, and protein and is an alternative to organic extraction or column chromatography for DNA purification. More importantly, density gradient centrifugation in the presence of ethidium bro- mide (EtBr) can be used to separate supercoiled DNA from non-supercoiled molecules. Ethidium bromide binds to DNA molecules by intercalating between adjacent base pairs, causing partial unwinding of the double helix (Figure 3.15). This unwinding results in a decrease in the buoyant density, by as much as 0.125 g /cm3 for linear DNA. However, supercoiled DNA, with no free ends, has very little freedom to unwind, and can only bind a limited amount of EtBr. The decrease in buoyant density of a supercoiled molecule is therefore much less, only about 0.085 g /cm3. As a consequence, supercoiled molecules form a band in an EtBr–CsCl gradient at a different position to linear and open-circular DNA (Figure 3.16a). Ethidium bromide–caesium chloride density gradient centrifugation is a very efficient method for obtaining pure plasmid DNA. When a cleared lysate is subjected to this procedure, plasmids band at a distinct point, separated from the linear bacterial DNA, 38 Part I The Basic Principles of Gene Cloning and DNA Analysis Figure 3.15 NH2 Partial unwinding of the DNA double helix by EtBr intercalation between adjacent base pairs. The normal DNA molecule shown on the left is partially unwound by taking up four EtBr molecules, Chemical structure of N+ Br– resulting in the “stretched” structure on the right. ethidium bromide H2N C2H5 3.4Å Sugar- phosphate backbone Intercalated EtBr molecule EtBr 3.4Å >3.4Å Base pair Normal DNA double helix Protein EtBr in Linear and Shake with organic oc DNA n-butanol, allow phase Buffer layers to separate out Supercoiled DNA Remove DNA supercoiled DNA in solution in DNA with aqueous dialysis a syringe phase tubing RNA CsCl diffuses into buffer (a) An EtBr–CsCl (b) Removal of the (c) Extraction of the (d) Removal of the density gradient DNA band EtBr with n-butanol CsCl by dialysis Figure 3.16 Purification of plasmid DNA by EtBr–CsCl density gradient centrifugation. Chapter 3 Purification of DNA from Living Cells 39 Incubate in the presence of chloramphenicol Multicopy plasmids Bacterial Plasmids present at (copy number 20+) DNA a copy number of 1000+ Figure 3.17 Plasmid amplification. with the protein floating on the top of the gradient and RNA pelleted at the bottom. The position of the DNA bands can be seen by shining ultraviolet radiation on the tube, which causes the bound EtBr to fluoresce. The pure plasmid DNA is removed by puncturing the side of the tube and withdrawing a sample with a syringe (Figure 3.16b). The EtBr bound to the plasmid DNA is extracted with n-butanol (Figure 3.16c) and the CsCl removed by dialysis (Figure 3.16d). The resulting plasmid preparation is virtually 100% pure and ready for use as a cloning vector. 3.2.3 Plasmid amplification Preparation of plasmid DNA can be hindered by the fact that plasmids make up only a small proportion of the total DNA in the bacterial cell. The yield of DNA from a bacterial culture may therefore be disappointingly low. Plasmid amplification offers a means of increasing this yield. The aim of amplification is to increase the copy number of a plasmid. Some multi- copy plasmids (those with copy numbers of 20 or more) have the useful property of being able to replicate in the absence of protein synthesis. This contrasts with the main bacterial chromosome, which cannot replicate under these conditions. This property can be utilized during the growth of a bacterial culture for plasmid DNA purification. After a satisfactory cell density has been reached, an inhibitor of protein synthesis (e.g., chloramphenicol) is added, and the culture incubated for a further 12 hours. During this time the plasmid molecules continue to replicate, even though chromosome replication and cell division are blocked (Figure 3.17). The result is that plasmid copy numbers of several thousand may be attained. Amplification is therefore a very efficient way of increasing the yield of multicopy plasmids. 3.3 Preparation of bacteriophage DNA The key difference between phage DNA purification and the preparation of either total cell DNA or plasmid DNA is that for phages the starting material is not normally a cell extract. This is because bacteriophage particles can be obtained in large numbers from the extracellular medium of an infected bacterial culture. When such a culture is centrifuged, the bacteria are pelleted, leaving the phage particles in suspension (Figure 3.18). The phage particles are then collected from the suspension and their DNA extracted by a single deproteinization step to remove the phage capsid. This overall process is more straightforward than the procedure used to prepare total cell or plasmid DNA. Nevertheless, successful purification of significant quantities of 40 Part I The Basic Principles of Gene Cloning and DNA Analysis Figure 3.18 Phage suspension Preparation of a phage suspension from an infected culture of bacteria. Centrifuge Infected culture (bacteria + extracellular phage) Pellet of bacteria phage DNA is subject to several pitfalls. The main difficulty, especially with e, is growing an infected culture in such a way that the extracellular phage titer (the number of phage particles per ml of culture) is sufficiently high. In practical terms, the maximum titer that can reasonably be expected for e is 1010 per ml; yet 1010 e particles will yield only 500 ng of DNA. Large culture volumes, in the range of 500–1000 ml, are therefore needed if substantial quantities of e DNA are to be obtained. 3.3.1 Growth of cultures to obtain a high * titer Growing a large volume culture is no problem (bacterial cultures of 100 liters and over are common in biotechnology), but obtaining the maximum phage titer requires a certain amount of skill. The naturally occurring e phage is lysogenic (p. 19), and an infected culture consists mainly of cells carrying the prophage integrated into the bacterial DNA (see Figure 2.7). The extracellular e titer is extremely low under these circumstances. To get a high yield of extracellular e, the culture must be induced, so that all the bacteria enter the lytic phase of the infection cycle, resulting in cell death and release of e particles into the medium. Induction is normally very difficult to control, but most laboratory strains of e carry a temperature-sensitive (ts) mutation in the cI gene. This is one of the genes that are responsible for maintaining the phage in the integrated state. If inactivated by a mutation, the cI gene no longer functions correctly and the switch to lysis occurs. In the cIts mutation, the cI gene is functional at 30°C, at which temperature normal lysogeny can occur. But at 42°C, the cIts gene product does not work properly, and lysogeny cannot be maintained. A culture of E. coli infected with a e phages carrying the cIts mutation can therefore be induced to produce extracellular phages by transferring from 30°C to 42°C (Figure 3.19). 3.3.2 Preparation of non-lysogenic * phages Although most e strains are lysogenic, many cloning vectors derived from e are modified, by deletions of the cI and other genes, so that lysogeny never occurs. These phages cannot integrate into the bacterial genome and can infect cells only by a lytic cycle (p. 18). With these phages the key to obtaining a high titer lies in the way in which the culture is grown, in particular the stage at which the cells are infected by adding phage particles. If phages are added before the cells are dividing at their maximal rate, then all the cells are lysed very quickly, resulting in a low titer (Figure 3.20a). On the other hand, if the cell density is too high when the phages are added, then the culture will never be completely lysed, and again the phage titer will be low (Figure 3.20b). The ideal situation is when the age of the culture, and the size of the phage inoculum, are balanced such that the culture continues to grow, but eventually all the cells are infected Chapter 3 Purification of DNA from Living Cells 41 λ prophage 30°C No induction 42°C Induction Excised λ genome Cell lysis New λ particles Figure 3.19 Induction of a λ cIts lysogen by transferring from 30°C to 42°C. λ inoculum Figure 3.20 Achieving the right balance between culture age and inoculum size when preparing a sample of a Add phage non-lysogenic phage. Bacteria All the cells are rapidly lysed = low phage titer (a) Culture density is too low Culture is never completely lysed = low phage titer (b) Culture density is too high Culture continues to grow but is eventually lysed = high phage titer (c) Culture density is just right 42 Part I The Basic Principles of Gene Cloning and DNA Analysis Figure 3.21 Collection of phage particles by Add PEG polyethylene glycol (PEG) precipitation. + NaCl Centrifuge Phage Precipitated Pellet of phage particles Resuspend suspension phage particles and cell debris pellet and lysed (Figure 3.20c). As can be imagined, skill and experience are needed to judge the matter to perfection. 3.3.3 Collection of phages from an infected culture The remains of lysed bacterial cells, along with any intact cells that are inadvertently left over, can be removed from an infected culture by centrifugation, leaving the phage particles in suspension (see Figure 3.18). The problem now is to reduce the size of the suspension to 5 ml or less, a manageable size for DNA extraction. Phage particles are so small that they are pelleted only by very high speed centrifuga- tion. Collection of phages is therefore usually achieved by precipitation with polyethy- lene glycol (PEG). This is a long-chain polymeric compound which, in the presence of salt, absorbs water, thereby causing macromolecular assemblies such as phage particles to precipitate. The precipitate can then be collected by centrifugation, and redissolved in a suitably small volume (Figure 3.21). 3.3.4 Purification of DNA from * phage particles Deproteinization of the redissolved PEG precipitate is sometimes sufficient to extract pure phage DNA, but usually e phages are subjected to an intermediate purification step. This is necessary because the PEG precipitate also contains a certain amount of bacterial debris, possibly including unwanted cellular DNA. These contaminants can be separated from the e particles by CsCl density gradient centrifugation. The e particles band in a CsCl gradient at 1.45–1.50 g /cm3 (Figure 3.22), and can be withdrawn from Figure 3.22 1.35 Purification of λ phage particles by CsCl density gradient centrifugation. 1.40 CsCl density (g/cm3) 1.45 λ phage particles 1.50 1.55 1.60 Chapter 3 Purification of DNA from Living Cells 43 the gradient as described previously for DNA bands (see Figure 3.16). Removal of CsCl by dialysis leaves a pure phage preparation from which the DNA can be extracted by either phenol or protease treatment to digest the phage protein coat. 3.3.5 Purification of M13 DNA causes few problems Most of the differences between the M13 and e infection cycles are to the advantage of the molecular biologist wishing to prepare M13 DNA. First, the double-stranded replicative form of M13 (p. 23), which behaves like a high copy number plasmid, is very easily purified by the standard procedures for plasmid preparation. A cell extract is prepared from cells infected with M13, and the replicative form separated from bacterial DNA by, for example, EtBr–CsCl density gradient centrifugation. However, the single-stranded form of the M13 genome, contained in the extracellu- lar phage particles, is frequently required. In this respect the big advantage compared with e is that high titers of M13 are very easy to obtain. As infected cells continually secrete M13 particles into the medium (see Figure 2.8), with lysis never occurring, a high M13 titer is achieved simply by growing the infected culture to a high cell density. In fact, titers of 1012 per ml and above are quite easy to obtain without any special tricks being used. Such high titers mean that significant amounts of single-stranded M13 DNA can be prepared from cultures of small volume—5 ml or less. Furthermore, as the infected cells are not lysed, there is no problem with cell debris contaminating the phage suspension. Consequently the CsCl density gradient centrifugation step, needed for e phage preparation, is rarely required with M13. In summary, single-stranded M13 DNA preparation involves growth of a small volume of infected culture, centrifugation to pellet the bacteria, precipitation of the phage particles with PEG, phenol extraction to remove the phage protein coats, and ethanol precipitation to concentrate the resulting DNA (Figure 3.23). M13 phage suspension M13 DNA Protein Phenol Pelleted Precipitated M13 DNA cells M13 phage (a) Culture of (b) Centrifuge (c) Add PEG to (d) Resuspend (e) Add phenol (f) Remove (g) Resuspend infected cells to remove cells phage suspension, phage in buffer to remove aqueous layer, M13 DNA in a centrifuge protein capsid add ethanol, small volume centrifuge Figure 3.23 Preparation of single-stranded M13 DNA from an infected culture of bacteria. 44 Part I The Basic Principles of Gene Cloning and DNA Analysis Further reading FURTHER READING Birnboim, H.C. & Doly, J. (1979) A rapid alkaline extraction procedure for screening recom- binant plasmid DNA. Nucleic Acids Research, 7, 1513–1523. [A method for preparing plasmid DNA.] Boom, R., Sol, C.J.A., Salimans, M.M.M., Jansen, C.L., Wertheim van Dillen, P.M.E. & van der Noordaa, J. (1990) Rapid and simple method for purification of nucleic acids. Journal of Clinical Microbiology, 28, 495–503. [The guanidinium thiocyanate and silica method for DNA purification.] Clewell, D.B. (1972) Nature of ColE1 plasmid replication in Escherichia coli in the presence of chloramphenicol. Journal of Bacteriology, 110, 667– 676. [The biological basis of plasmid amplification.] Marmur, J. (1961) A procedure for the isolation of deoxyribonucleic acid from microorgan- isms. Journal of Molecular Biology, 3, 208–218. [Genomic DNA preparation.] Radloff, R., Bauer, W. & Vinograd, J. (1967) A dye-buoyant-density method for the detec- tion and isolation of closed-circular duplex DNA. Proceedings of the National Academy of Sciences of the USA, 57, 1514–1521. [The original description of ethidium bromide density gradient centrifugation.] Rogers, S.O. & Bendich, A.J. (1985) Extraction of DNA from milligram amounts of fresh, herbarium and mummified plant tissues. Plant Molecular Biology, 5, 69–76. [The CTAB method.] Yamamoto, K.R., Alberts, B.M., Benzinger, R., Lawhorne, L. & Trieber, G. (1970) Rapid bacteriophage sedimentation in the presence of polyethylene glycol and its application to large scale virus preparation. Virology, 40, 734–744. [Preparation of l DNA.] Zinder, N.D. & Boeke, J.D. (1982) The filamentous phage ( Ff ) as vectors for recombinant DNA. Gene, 19, 1–10. [Methods for phage growth and DNA preparation.]