Fabrication and Characterization of Boric Acid-Crosslinked Ethyl Cellulose and Polyvinyl Alcohol Films for Topical Drug Delivery PDF
Document Details
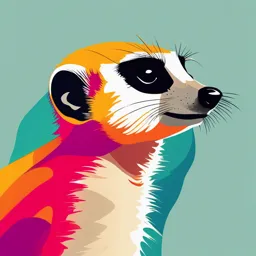
Uploaded by pepinos
Brock University
2020
Nasrin Aliasgharlou, Farzin Asghari Sana, Saba Khoshbakht, Pezhman Zolfaghari, Hamed Charkhian
Tags
Related
- Organic Pharmaceutical Chemistry IV Lecture 6 (2017-2018) PDF
- Nanoparticles in Drug Delivery: From History to Therapeutic Applications PDF
- Synthesis and Evaluation of pH- and Temperature-Responsive Chitosan-p(MAA-co-NIPAM) Hydrogels PDF
- Nanotechnology and Applications in Medicine Presentation PDF
- Chapter 12 Fundamentals of a targeted drug delivery system PDF
- Targeted Drug Delivery System Fundamentals PDF
Summary
This research article details the fabrication and characterization of boric acid-crosslinked ethyl cellulose and polyvinyl alcohol films as potential drug delivery systems for topical applications. The study focuses on improving the characteristics of these films for controlled drug release. The developed formulations are studied for antibacterial activity and drug release properties.
Full Transcript
Turkish Journal of Chemistry Turk J Chem (2020) 44: 1723-1732 http://journals.tubitak.gov.tr/chem/...
Turkish Journal of Chemistry Turk J Chem (2020) 44: 1723-1732 http://journals.tubitak.gov.tr/chem/ © TÜBİTAK Research Article doi:10.3906/kim-2008-23 Fabrication and characterization of boric acid-crosslinked ethyl cellulose and polyvinyl alcohol films as potential drug release systems for topical drug delivery 1 2,3, 3 4 Nasrin ALIASGHARLOU , Farzin ASGHARI SANA *, Saba KHOSHBAKHT , Pezhman ZOLFAGHARI , Hamed 5 CHARKHIAN 1 Department of Chemistry, Faculty of Science, Urmia University, Urmia, Iran 2 Department of Nanotechnology & Nanomedicine, Hacettepe University, Ankara, Turkey 3 Department of Pharmaceutics, School of Pharmacy, Urmia University of Medical Science, Urmia, Iran 4 Department of Chemical Engineering, Faculty of Chemical and Petroleum Engineering, University of Tabriz, Tabriz, Iran 5 Young Researchers Club, Urmia Branch, Islamic Azad University, Urmia, Iran Received: 15.08.2020 Accepted/Published Online: 06.11.2020 Final Version: 16.12.2020 Abstract: In this study, boric acid (BA) is employed as a crosslinking agent to improve the characteristics of two commonly used polymeric films, ethyl cellulose (EC) and polyvinyl alcohol (PVA), for topical drug delivery applications. The developed films are characterized by FTIR spectroscopy and SEM analysis. The results show that the surfaces of the prepared films are even and transparent, except for the BA-modified EC sample. The initial cumulative release for erythromycin (EM) is found to be 0.30 and 0.36 mg/mL for EC and PVA films, which drops to 0.25 and 0.20 mg/mL after BA crosslinking, respectively, after 1 h at 25 °C. Further, the developed formulations are stable for 75 days. Also, the antibacterial activity of the developed formulations is investigated against S. aureus (ATCC® 25923™ and ATCC® 29213™). The obtained data confirm that the application of BA as the crosslinking agent extends the release of EM from EC and PVA polymeric films. The findings of this study suggest that BA-crosslinked EC and PVA films are promising carriers for controlled topical drug delivery applications. Key words: Drug delivery system, polymeric film, polyvinyl alcohol, ethyl cellulose, boric acid, erythromycin 1. Introduction In recent years, drug delivery systems (DDSs) have been widely investigated by researchers owing to their numerous advantages, such as minimizing the side effects of drugs, better targeting, and cost-effectiveness. Various sustained- release systems have been developed to adopt an effectively controlled drug release, including but not limited to particulate carriers, lipids, polymeric films, and gels [2–4]. Researchers have strived to develop pharmaceutical formulations based on polymeric films due to their drug-related properties, biocompatibility, and nontoxicity. Assorted natural and synthetic polymers have been used in film formation processes and classified into two major categories: hydrophilic and hydrophobic films [5,6]. Ethyl cellulose (EC), as a hydrophobic polysaccharide polymer, is a biodegradable and biocompatible polymer with unique properties such as high stability as well as heat, oxygen, and moisture resistance. Further, EC has desirable solubility in different organic solvents and is miscible with various water-soluble materials. It has excellent film-forming, filling, and adhesive capabilities, promoting its application in food, cosmetics, and pharmaceutical industries, especially as packaging material, coating, and encapsulating agents [7–9].Contrary to EC, polyvinyl alcohol (PVA) is a hydrophilic synthetic polymer with a linear and flexible structure. Being approved by Food and Drug Administration (FDA), PVA has widespread applications in cosmetics and pharmaceutical industries. It is known as a versatile carrier in sustained drug release systems in terms of various fibers, gels, and films due to its distinctive characteristics. However, high moisture absorption and low mechanical strength hindered its application as a protective barrier [11–14]. The efficacy of polymeric films as DDSs is mainly determined by their stability, controlled, and selective drug release. Thus, the improvement of their properties is still a necessity to attain better results. Crosslinking is a promising method to enhance the structural and mechanical properties of polymers [15,16]. Boric acid (BA) is widely utilized in polymeric composites, owing to its ease of functionalizing and safety. It has been established as an eco-friendly material and can aid * Correspondence: [email protected] 1723 This work is licensed under a Creative Commons Attribution 4.0 International License. ALIASGHARLOU et al. / Turk J Chem plant growth by acting as a nutrition source. Additionally, its therapeutic effects against a wide range of bacteria, yeast, and fungi are well proven [17–19]. It was also proven that boronic acid derivatives are selective inhibitors of the serine protease (β-lactamase family), while being cheaper than other alternatives. The incorporation of BA with antibiotics can offer a new platform to overcome antibiotic resistance, which is a major public health challenge [20,21]. This study aimed to develop erythromycin-loaded PVA and EC films as topical controlled-release drug delivery systems. Erythromycin (EM) is a hydrophobic macrolide antibiotic with a therapeutic effect against gram-positive and gram-negative bacteria via blocking protein synthesis in the ribosome of bacteria. However, EM contains a high quantity of alcohol for topical treatments, provoking skin irritations in patients [22,23]. Nevertheless, to the best of our knowledge, the effect of BA on the physicochemical properties of polymeric matrices has sparsely been investigated. The main purpose of this work was to examine the effect of BA on the in vitro drug release and the physicochemical properties of PVA- and EC-based films. Also, the possibility of synergistic antibacterial activity of BA in conjunction with EM in the developed films was explored. 2. Materials and methods 2.1. Materials Erythromycin was purchased from Sepidaj Pharmaceutical, Iran. PVA (MW: 89000-91000 g/mol) and EC (Ethoxy groups, viscosity: 10 mPa.s) was obtained from Sigma-Aldrich, USA. Two standard strains of S. aureus, including S. aureus (ATCC® 25923™) and S. aureus (ATCC® 29213™), were purchased from KWICK-STICK Microbiologics, France. Mueller-Hinton Agar (MHA) and Mueller-Hinton Broth (MHB) were obtained from Becton Dickinson, France. The RPMI (Roswell Park Memorial Institute) 1640 W/L-Glutamine was obtained from Bio West, Germany. All other chemicals and reagents are analytical grade and used without purification. 2.2. Film preparation EC films were prepared by dissolving 5% w/v of EC in an ethanol-water solution (80% w/w of ethanol). PVA films were fabricated by the addition of PVA (5% w/v) to deionized water. The developed solutions were heated up to 90 °C under mild stirring. To prepare the blended PVA-BA and EC-BA mixtures, the solutions were cooled down to reach a temperature of 45 °C, and 1% w/v BA was added to the resulted solutions under mild stirring. After the dissolution of BA, the pH was adjusted to 7 using a 1M NaOH. Afterward, EM (0.75% w/v) was added to the prepared gels and poured into Petri dishes. Finally, the developed films were dried in a vacuum chamber. Table 1 reports the composition of the developed films and their respective abbreviations, which will be utilized throughout this paper. 2.3. Characterization The morphological structures of the films were assessed using a scanning electron microscope (SEM; Zeiss Co., Germany) with an acceleration voltage of 20 kV. Fourier transform infrared spectrometer (FTIR; Perkin Elmer) was utilized to characterize the chemical structures of PVA, EC, and crosslinked films. The spectra were recorded in the range of 500 to 4000 cm–1 with a resolution of 4 cm–1. 2.4. Swelling index and thickness The effect of BA on the swelling behavior of the developed films was examined in water. Samples were prepared with a surface area of 100 × 100 mm, weighed, and then immersed in the aqueous medium. The weight of the dried sample Table 1. Composition of the polymeric films. PVA EC BA EM Drug Abbreviation (% w/v) (% w/v) (% w/v) (% w/v) Loading (%) P.f 5 – – – – E.f – 5 – – – PB.f 5 – 1 – – EB.f – 5 1 – – P-EM.f 5 – – 0.075 1.45 E-EM.f – 5 – 0.075 1.5 PB-EM.f 5 – 1 0.075 1.22 EB-EM.f – 5 1 0.075 1.26 1724 ALIASGHARLOU et al. / Turk J Chem was determined before and after the swelling process, while the surface wetness was removed using dry filter paper. The swelling index of each film was calculated using the following equation: 𝑊𝑊1 − 𝑊𝑊3 𝑠𝑠𝑠𝑠𝑠𝑠𝑠𝑠𝑠𝑠𝑠𝑠𝑠𝑠𝑠𝑠 𝑖𝑖𝑖𝑖𝑖𝑖𝑖𝑖𝑖𝑖(𝑆𝑆𝑆𝑆) = × 100 (1) 𝑊𝑊3 Here Wt is the weight of swollen film at time t , and W0 corresponds to the dry film sample, respectively [24,25]. The 𝐷𝐷𝐷𝐷𝐷𝐷𝐷𝐷 𝑐𝑐𝑐𝑐𝑐𝑐𝑐𝑐𝑐𝑐𝑐𝑐𝑐𝑐 (𝑚𝑚𝑚𝑚) 𝐿𝐿𝐿𝐿𝐿𝐿𝐿𝐿𝐿𝐿𝐿𝐿𝐿𝐿thickness film’s 𝑑𝑑𝑑𝑑𝑑𝑑𝑑𝑑% =(mm) was measured using a×Palmer 100 digital micrometer. The measurements were conducted at different points of each sample and the 𝑤𝑤𝑤𝑤𝑤𝑤𝑤𝑤ℎ𝑡𝑡 𝑁𝑁𝑁𝑁𝑁𝑁𝑁𝑁𝑁𝑁𝑁𝑁𝑁𝑁𝑁𝑁𝑁𝑁 average (𝑚𝑚𝑚𝑚) value was reported. 2.5. In vitro drug release The actual EM content was determined using the total immersion method. In brief, a predetermined amount of each sample was dissolved in phosphate buffer solution (PBS, pH: 7.4) and placed in a shaker incubator (rotation speed of 100 rpm) at the temperatures of 25 and 32 °C. Eventually, a desired amount of samples was collected and replaced with the same volume of fresh𝑖𝑖𝑖𝑖𝑖𝑖𝑖𝑖𝑖𝑖(𝑆𝑆𝑆𝑆) PBS. The = 𝑊𝑊1 − 𝑊𝑊3 drug release was determined by UV-vis spectrophotometer (Cecil CE 7200, cumulative 𝑠𝑠𝑠𝑠𝑠𝑠𝑠𝑠𝑠𝑠𝑠𝑠𝑠𝑠𝑠𝑠 × 100 UK) at the wavelength of 202 nm. The𝑊𝑊 following 3 equation was applied to measure the EM Loading dose (%): 𝐷𝐷𝐷𝐷𝐷𝐷𝐷𝐷 𝑐𝑐𝑐𝑐𝑐𝑐𝑐𝑐𝑐𝑐𝑐𝑐𝑐𝑐 (𝑚𝑚𝑚𝑚) (2) 𝐿𝐿𝐿𝐿𝐿𝐿𝐿𝐿𝐿𝐿𝐿𝐿𝐿𝐿 𝑑𝑑𝑑𝑑𝑑𝑑𝑑𝑑% = × 100 𝑁𝑁𝑁𝑁𝑁𝑁𝑁𝑁𝑁𝑁𝑁𝑁𝑁𝑁𝑁𝑁𝑁𝑁 𝑤𝑤𝑤𝑤𝑤𝑤𝑤𝑤ℎ𝑡𝑡 (𝑚𝑚𝑚𝑚) 2.6. Stability studies The stability tests of EM-loaded films were carried out at 25 ºC (room temperature) and 4 ºC (fridge temperature) for 75 days. For this purpose, samples were collected to measure the drug content spectrophotometrically at the wavelength of 202 nm. 2.7. Zone of inhibition for antibacterial activities Antibacterial activities of the prepared polymer-based formulations were evaluated against gram-positive bacteria S. aureus (ATCC® 25923™ and ATCC® 29213™) by measuring the inhibition zone around each film disc using disc diffusion methodology. MHA plates were seeded with 0.1 mL of bacteria suspensions with a bacterium count of 108 CFU/mL. Circular discs with a diameter of 6 mm were obtained from the films and placed on the MHA medium previously inoculated with the test bacteria. The agar plates were incubated at 35 °C for 24 h and the inhibition zones of the developed formulations were determined. 3. Results and discussions 3.1. Characterization The SEM images of the EM-loaded blank (E-EM.f and P-EM.f) and crosslinked (EB-EM.f and PB-EM.f) films are shown in Figure 1. The P-EM.f sample displayed a smooth and even surface. Likewise, the E-EM.f micrographs revealed a slightly homogenous surface with some nanopores corresponding to the application of the mixture of water-ethanol as the solvent. Hjartstam et al. fabricated EC film by dissolving EC powder in various mixtures of water/ethanol compositions and reported that the film porosity increases with the elevation of water content. The PB-EM.f film had a comparatively smooth surface texture, which showed that BA was scattered homogenously, improving the surface integrity. On the other hand, the presence of BA in the EB-EM.f film formed some circular bubbles instead of pores on the film’s surface. Also, the SEM micrographs indicated the presence of some insolubilized EM particles on the surface of the films. Figure 2 presents the FTIR spectra of the fabricated films. For the P.f sample, an absorption peak was observed around the wavenumber of 3525 cm–1, which is ascribed to the vibrations of hydroxyl groups, while the peak at 1720 cm–1 is attributed to the stretching of the C=O group. Also, the C–H bond vibrations resulted in a sharp band at 2954 cm–1. The FTIR spectra curve of pure E.f sample demonstrated peaks at 3470, 2923, 1466, and 1384 cm–1 corresponding to OH, CH, CH2, and CH3 bond stretching vibrations, respectively. The formulations containing boric acid exhibited a peak within the region of 1050–1150 cm–1, suggesting the formation of B-O-C bonds [32,33]. The FTIR spectra of EM indicated absorbances at 3480, 2974, and 1715 cm−1, corresponding to OH, CH, and C=O stretching vibrations, respectively. However, there was no discrete band in the blank and EM-loaded films (E-EM.F, P-EM.F, EB-EM.F, and PB-EM.F), which may be related to lack of chemical reaction between EM and the polymers. 3.2. Swelling index and thickness The swelling index (SI) of the developed films was measured at room temperature. The results depicted by Figure 3 revealed that the EB.f and PB.f samples have had a lower SI compared to E.f and P.f films, which can be related to the crosslinking and filling effect of BA. Accordingly, BA increases the viscosity of the polymeric film and fills the pores 1725 ALIASGHARLOU et al. / Turk J Chem Figure 1. SEM images of the fabricated films; (a) P-EM.f, (b) PB-EM.f, (c) E-EM.f, and (d) EB-EM.f. 1726 ALIASGHARLOU et al. / Turk J Chem EB-EM.f E-EM.f EB.f E.f BA EM 2928 Transmittance (a.u) 1061 3445 2927 3465 2925 1070 3450 1384 1466 2923 3470 1715 2974 3480 500 1000 1500 2000 2500 3000 3500 4000 Wave number (cm-1 ) PB-EM. f PB-EM. f PB.f P.f EM BA 1116 1734 2945 3434 Transmittance (a.u) 1735 2943 1736 2932 3409 1124 3422 1720 2954 3527 1715 2974 3480 500 1000 1500 2000 2500 3000 3500 4000 Wave number (cm-1 ) Figure 2. FTIR spectra of EM, BA, and the developed a) EC-based films; b) PVA-based films of the polymeric films. As shown in Figure 3, the introduction of BA to the PVA film (PB.f) caused a more significant reduction in SI compared to BA-crosslinked EC samples (EB.f), which may be associated with the stronger crosslinking effect of BA with PVA. As a drug delivery platform, the developed films should exhibit an even surface, which guarantees consistent mechanical properties and drug distribution. For this purpose, an equal amount of homogenous gels was poured into the Petri dishes located on an even surface to form the films. Figure 3 depicts the mean thickness values of the manufactured films varying from 80.33 to 107.33 mm. Also, insignificant standard deviation values verify the homogenous formulation of the films, except for the EB.f sample, which has a relatively diverse thickness distribution. 3.3. In vitro drug release The EM loading quantities for the developed formulations are tabulated in Table 1. According to this table, drug loading decreased upon the addition of BA. Figure 4 demonstrates the in vitro EM release profile of all samples carried out in phosphate buffer at 25 °C (room temperature) and 32 °C (simulated skin temperature). The cumulative EM release was found to be 0.36, 0.20, 0.30, and 0.25 mg/mL in the first hour for P-EM.F, PB-EM.F, E-EM.F, and EB-EM.F formulations, respectively, at 25 °C. As observed, all formulations exhibited a rapid drug release in the first hours, followed by a sustained drug release path, which is desirable for the treatment of skin infections, considering the initial pathological load. P-EM.F 1727 ALIASGHARLOU et al. / Turk J Chem 350 E-EM.F 25 E-EM.F 32 EB-EM.F 32 EB-EM.F 32 0.7 P.f PB.f E.f EB.f 300 0.6 Cumulative drug release (%) 250 0.5 Swelling Index (%) 200 0.4 0.3 150 0.2 100 0.1 50 0 0 12 24 36 48 60 72 84 96 0 Time (h) P-EM.f 25 P-EM.f 32 PB-EM.f 25 PB-EM.f 32 140 0.5 Cumulative drug release (%) 120 P PB E EB 0.4 100 0.3 Thickness (µm) 80 0.2 60 0.1 40 20 0 0 3 6 9 12 15 0 Time (h) Figure 3. Swelling index (a) and Thickness (b) of the developed Figure 4. a) Release profiles of EM from E-EM.f and EB-EM.f films films at 25 and 32 °C; b) EM Release profiles from P-EM.f and PB-EM.f films at 25 and 32 °C. and PB-EM.F samples released more than 0.35 mg/mL of the drug during the first and eighth hours, respectively, which was sustained with a slower rate after 15 h. However, E-EM.F and EB-EM.F samples reached this milestone at 2 and 22 h at the temperature 25 °C, respectively, promising a finely controlled drug release. The initial burst EM release may result from the presence of drug particles on the surface of the films, which was confirmed by the SEM micrographs. Various parameters affect the drug diffusion from a polymeric matrix, including temperature, degradation of the polymeric matrix, and the solubility behavior of drug and polymer. In this research, the model drug (EM) was hydrophobic, retarding its release from the polymeric matrix with a slow-release path when compared to hydrophilic drugs. It was shown that EC-based drug formulations have a prolonged drug release profile due to the hydrophobic nature of the polymeric carrier, leading to a lower erosion rate of EC films in aqueous environments. As a result, the entrapped EM molecules in the EC polymeric matrix diffused slowly to the phosphate buffer medium. On the other hand, EM had a shorter release path using PVA carrier due to the fast dissolution of the polymer in aqueous solutions. For all of the formulations, the EM release rate was higher at 32 °C compared to 25 °C. This phenomena can be attributed to the lower stability of EM at higher temperatures [23,37]. The introduction of BA as the crosslinking agent formed hydrogen bonds, which were weakened at higher temperatures and led to a reduction of the crosslinking effect. Further, PB-EM.f and EB-EM.f films freed EM at a slow rate in comparison to P-EM.f and E-EM.f formulations, respectively. BA could enhance the viscosity and surface tension of the system, delaying drug diffusion in the polymeric matrix. Also, BA lowered the dissolution rate and increased the water resistance of PVA. Subrahmanyam et al. prepared theophylline tablets using guar gum and borax modified guar gum as a colon DDS. It has been reported that borax-crosslinked guar gum had a slower release path, explained by the enhancement of the viscosity. In another study, Martinez et al. studied the effect of the glutaraldehyde, genipin, and disulfide as crosslinking agents on the in vitro drug release of protein polymers. The results revealed that crosslinked films exhibited a reduced initial burst release of rapamycin. Various polymers can be used in film formulations as DDSs, 1728 ALIASGHARLOU et al. / Turk J Chem depending on the release pattern required for the treatment of infections. The obtained drug release results indicate that the introduction of BA as the crosslinking agent successfully improved the sustained release of EM using both polymeric carriers. Kown et al. employed EM-loaded PVA films as a controlled transdermal drug release system for acne treatment. In their study, they added polyethylene glycol600 and glycerol to the fabricated films to extend the EM release profile. The release of EM from blended polyethylene glycol/PVA showed an initial burst release with a sustained release for the next hours, which was similar to the drug release behavior of PB-EM.f sample. 3.4. Stability The stability of EM in the developed EM-loaded films at room (RT, 25 °C) and fridge (FT, 4 °C) temperatures was assessed for 75 days. The aqueous drug solution was used as the control group and sealed to prevent light irradiation and drug degradation. After 75 days, the stability of the control sample was found to be 97.2 ± 2.12% and 97.67 ± 1.49% at RT and FT, respectively. As observed in Figure 5, the drug was stable over 75 days of storage in all formulations. Further, there was no difference in the EM stability of the drug-loaded films at both investigated temperatures, eliminating a low-temperature storage requirement. However, it was found that borate ion accelerates the degradation of some drugs such as benzylpenicillin, carbenicillin, cefotaxime, atropine, hydrocortisone, indomethacin, methotrexate, oxytetracycline, minocycline, and 5-fluorouracil. In the present study, it was shown that BA, as a borate compound, did not have any negative effect on the EM stability. 3.5. In vitro antibacterial assay ® The antimicrobial activity of the prepared films was investigated against methicillin-susceptible S. aureus (ATCC 25923 ), ™ ® ™ and oxacillin sensitive S. aureus (ATCC 29213 ) by Kirby Bauer disc diffusion tests (Figure 6). As it was shown in Table ® ™ 2, EB.f and PB.f formulations did not exhibit any antibacterial activity against (ATCC 25923 ) and (ATCC 29213 ). Boric acid as a crosslinking agent forms bonds with the polymers via hydroxyl groups. Also, it was demonstrated that an ® ™ increase in the number of free B-OH groups directly improves the antibacterial effect [19,43,44]. However, in the present study, BA may be surrounded by PVA and EC molecules, which reduces the number of free B-OH groups, leading to the inhibition of the bacterial growth. 7 30 60 75 EM Solution (FT) EM Solution (RT) PB-EM.f (FT) PB-EM.f (RT) P-EM.f (FT) P-EM.f (RT) EB-EM.f (FT) EB-EM.f (RT) E-EM.f (FT) E-EM.f (RT) 94 95 96 97 98 99 100 Stability (%) Figure 5. Stability tests of the fabricated formulation after 7, 30, 60, and 75 days. 1729 ALIASGHARLOU et al. / Turk J Chem 1 Figure 6. Inhibition zones of E-EM.f, EB-EM.f, P-EM.f and PB-EM.f samples against S. aureus ATCC25923 and S. aureus ATCC29213. 2 Figure 6. Inhibition zones of E-EM.f, EB-EM.f, P-EM.f and PB-EM.f samples against S. Table 2. Antibacterial activity of the developed formulations and standard antibiotic. 3 aureus ATCC25923 and S. aureus ATCC29213. Strains Sensitivity diameter (mm) 4 Samples Standard antibiotic 5 PB.f P-EM.f PB-EM.f EB.f E-EM.f EB-EM.f EM 15 S. aureus ATCC25923 0 30 27 0 30 32 30 S. aureus ATCC29213 0 38 22 0 38 30 33 Accordingly, E-EM.f and P-EM.f samples had similar antibacterial effects against (ATCC® 25,923™) and (ATCC® 29,213™), with inhibition zones of 30 and 38 mm, respectively. However, BA demonstrated synergistic antibacterial activity , noncrosslinked films expressing a larger inhibition zone compared to crosslinked formulations. Further, the EB-EM.f sample showed a greater inhibition zone against both bacteria in comparison with PB-EM.f. These may be related to the crosslinking effect of BA on the polymers, retarding EM diffusion. As shown in Figure 4, BA delayed EM diffusion from the polymeric matrix. 4. Conclusions In the current study, BA-crosslinked EC and PVA films were developed for topical drug delivery. The effect of BA was examined on the morphological structure, physicochemical properties, drug release, stability, and antibacterial activity of EM-loaded EC and PVA films. The results showed good compatibility between EM particles and polymeric films. The drug was stable in all formulations after 75 days, offering exceptional drug stability, regardless of storage conditions. The introduction of BA to the PVA and EC films not only managed to improve their swelling behaviors but also extend the drug release path, reducing the frequency of dressing change. The results obtained from the present study suggest that BA-modified EC and PVA films can be considered as efficient choices for various real-world topical DDSs without the side effects of conventional drug formulations. Ultimately, BA, as a cost-effective β-lactamases inhibitor, can be applied in conjunction with other antibiotics for combating bacterial resistance, which requires further investigations. Acknowledgement The authors are truly thankful to Hacettepe University and Urmia University of Medical Sciences for their support. 1730 23 ALIASGHARLOU et al. / Turk J Chem References 1. Alam MI, Paget T, Elkordy AA. Formulation and advantages of furazolidone in liposomal drug delivery systems. European Journal of Pharmaceutical Sciences 2016; 84: 139-145. doi: 10.1016/j.ejps.2016.01.017 2. Raghav N, Mor N, Gupta RD, Kaur R, Sharma MR et al. Some cetyltrimethylammonium bromide modified polysaccharide supports as sustained release systems for curcumin. International Journal of Biological Macromolecules 2020; 154: 361-370. doi: 10.1016/j.ijbiomac.2020.02.317 3. Steinberg D, Friedman M. Sustained-release drug delivery of antimicrobials in controlling of supragingival oral biofilms. Expert Opinion on Drug Delivery 2017; 14 (4): 571-581. doi: 10.1080/17425247.2016.1213239 4. Ahika S, Unaydin G, Yilmaz A. Improvement of solubility of celecoxib by inclusion in MCM-41 mesoporous silica: drug loading and release. Turkish Journal of Chemistry 2015; 39: 1-17. doi: 10.3906/kim-1409-56 5. Ghavami-Lahiji M, Shafiei F, Najafi F, Erfan M. Drug-loaded polymeric films as a promising tool for the treatment of periodontitis. Journal of Drug Delivery Science and Technology 2019; 52: 122-129. doi: 10.1016/j.jddst.2019.04.034 6. Morillon V, Debeaufort F, Capelle M, Blond G, Voilley A. Influence of the physical state of water on the barrier properties of hydrophilic and hydrophobic films. Journal of Agricultural and Food Chemistry 2000; 48 (1): 11-16. doi: 10.1021/jf990809z 7. Li X, Jiang F, Ni X, Yan W, Fang Y et al. Preparation and characterization of konjac glucomannan and ethyl cellulose blend films. Food Hydrocolloids 2015; 44: 229-236. doi: 10.1016/j.foodhyd.2014.09.027 8. Su X, Yang Z, Tan KB, Chen J, Huang J et al. Preparation and characterization of ethyl cellulose film modified with capsaicin. Carbohydrate Polymers 2020; 241: 116259. doi: 10.1016/j.carbpol.2020.116259 9. Shi J, Liu W, Jiang X, Liu W. Preparation of cellulose nanocrystal from tobacco-stem and its application in ethyl cellulose film as a reinforcing agent. Cellulose 2020; 27 (3): 1393-1406. doi: 10.1007/s10570-019-02904-0 10. Acik G. Soybean oil modified bio-based poly(vinyl alcohol)s via ring-opening polymerization. Journal of Polymers and the Environment 2019; 27 (11): 2618-2623. doi: 10.1007/s10924-019-01547-3 11. Tripathi S, Mehrotra GK, Dutta PK. Physicochemical and bioactivity of cross-linked chitosan–PVA film for food packaging applications. International Journal of Biological Macromolecules 2009; 45 (4): 372-376. doi: 10.1016/j.ijbiomac.2009.07.006 12. Ma Q, Du L, Yang Y, Wang L. Rheology of film-forming solutions and physical properties of tara gum film reinforced with polyvinyl alcohol (PVA). Food Hydrocolloids 2017; 63: 677-684. doi: 10.1016/j.foodhyd.2016.10.009 13. Monjazeb Marvdashti L, Koocheki A, Yavarmanesh M. Alyssum homolocarpum seed gum-polyvinyl alcohol biodegradable composite film: Physicochemical, mechanical, thermal and barrier properties. Carbohydrate Polymers 2017; 155: 280-293. doi: 10.1016/j.carbpol.2016.07.123 14. Meng F, Zhang Y, Xiong Z, Wang G, Li F et al. Mechanical, hydrophobic and thermal properties of an organic-inorganic hybrid carrageenan- polyvinyl alcohol composite film. Composites Part B: Engineering 2018; 143: 1-8. doi: 10.1016/j.compositesb.2017.12.009 15. Etxabide A, Coma V, Guerrero P, Gardrat C, de la Caba K. Effect of cross-linking in surface properties and antioxidant activity of gelatin films incorporated with a curcumin derivative. Food Hydrocolloids 2017; 66: 168-175. doi: 10.1016/j.foodhyd.2016.11.036 16. Uranga J, Leceta I, Etxabide A, Guerrero P, de la Caba K. Cross-linking of fish gelatins to develop sustainable films with enhanced properties. European Polymer Journal 2016; 78: 82-90. doi: 10.1016/j.eurpolymj.2016.03.017 17. Kader MA, Khan MA, Molla ME. Effect of boric acid on the properties of sorbitol plasticized starch/PVA blends. 2018 2018; 5 (2): 12. doi: 10.18034/ei.v5i2.1083 18. Rouhi M, Razavi SH, Mousavi SM. Optimization of crosslinked poly(vinyl alcohol) nanocomposite films for mechanical properties. Materials Science and Engineering: C 2017; 71: 1052-1063. doi: 10.1016/j.msec.2016.11.135 19. Donmez Cavdar A, Mengeloğlu F, Karakus K. Effect of boric acid and borax on mechanical, fire and thermal properties of wood flour filled high density polyethylene composites. Measurement 2015; 60: 6-12. doi: 10.1016/j.measurement.2014.09.078 20. Bush K, Bradford PA. Interplay between β-lactamases and new β-lactamase inhibitors. Nature Reviews Microbiology 2019; 17 (5): 295-306. doi: 10.1038/s41579-019-0159-8 21. Wang Y-L, Liu S, Yu Z-J, Lei Y, Huang M-Y et al. Structure-based development of (1-(3′-Mercaptopropanamido)methyl)boronic acid derived broad-spectrum, dual-action inhibitors of metallo- and serine-β-lactamases. Journal of Medicinal Chemistry 2019; 62 (15): 7160-84. doi: 10.1021/acs.jmedchem.9b00735 22. Jelić D, Antolović R. From erythromycin to azithromycin and new potential ribosome-binding antimicrobials. Antibiotics 2016; 5 (3): 29. doi: 23. Brisaert M, Heylen M, Plaizier-Vercammen J. Investigation on the chemical stability of erythromycin in solutions using an optimization system. Pharmacy World and Science 1996; 18 (5): 182-186. doi: 10.1007/BF00820730 24. Paolicelli P, Petralito S, Varani G, Nardoni M, Pacelli S et al. Effect of glycerol on the physical and mechanical properties of thin gellan gum films for oral drug delivery. International Journal of Pharmaceutics 2018; 547 (1): 226-234. doi: 10.1016/j.ijpharm.2018.05.046 1731 ALIASGHARLOU et al. / Turk J Chem 25. Evranos B, Aycan D, Alemdar N. Production of ciprofloxacin loaded chitosan/gelatin/bone ash wound dressing with improved mechanical properties. Carbohydrate Polymers 2019; 222: 115007. doi: 10.1016/j.carbpol.2019.115007 26. Hosseini MS, Nabid MR. Synthesis of chemically cross-linked hydrogel films based on basil seed (Ocimum basilicum L.) mucilage for wound dressing drug delivery applications. International Journal of Biological Macromolecules 2020; 163: 336-347. doi: 10.1016/j.ijbiomac.2020.06.252 27. Avizheh L, Peirouvi T, Diba K, Fathi-Azarbayjani A. Electrospun wound dressing as a promising tool for the therapeutic delivery of ascorbic acid and caffeine. Therapeutic Delivery 2019; 10 (12): 757-767. doi: 10.4155/tde-2019-0059 28. Azarbayjani AF, Talebi N, Diba K. Development and characterization of hydroquinone-loaded nanofiber for topical delivery: effect of chitosan. International Journal of Polymer Analysis and Characterization 2019; 24 (3): 227-235. doi: 10.1080/1023666X.2018.1564575 29. Hu D, Wang L. Physical and antibacterial properties of polyvinyl alcohol films reinforced with quaternized cellulose. Journal of Applied Polymer Science 2016; 133 (25): doi: 10.1002/app.43552 30. Hjärtstam J, Hjertberg T. Studies of the water permeability and mechanical properties of a film made of an ETHYL CELLULOSE–ETHANOL– WATER TErnary mixture. Journal of Applied Polymer Science 1999; 74 (8): 2056-2062. doi: 10.1002/(sici)1097-4628(19991121)74:83.0.co;2-y 31. Chetouani A, Elkolli M, Bounekhel M, Benachour D. Chitosan/oxidized pectin/PVA blend film: mechanical and biological properties. Polymer Bulletin 2017; 74 (10): 4297-4310. doi: 10.1007/s00289-017-1953-y 32. Prosanov IY, Abdulrahman ST, Thomas S, Bulina NV, Gerasimov KB. Complex of polyvinyl alcohol with boric acid: Structure and use. Materials Today Communications 2018; 14: 77-81. doi: 10.1016/j.mtcomm.2017.12.012 33. Chen J, Li Y, Zhang Y, Zhu Y. Preparation and characterization of graphene oxide reinforced PVA film with boric acid as crosslinker. Journal of Applied Polymer Science 2015; 132 (22): doi: 10.1002/app.42000 34. Alavi T, Rezvanian M, Ahmad N, Mohamad N, Ng S-F. Pluronic-F127 composite film loaded with erythromycin for wound application: formulation, physicomechanical and in vitro evaluations. Drug Delivery and Translational Research 2019; 9 (2): 508-519. doi: 10.1007/s13346- 017-0450-z 35. AKBAR MOHAMMADI JSM. Mesoporous starch aerogels production as drug delivery matrices: synthesis optimization, ibuprofen loading, and release property Turkish Journal of Chemistry 2020; 44: 614-633. doi: 10.3906/kim-1912-18 36. Caserta F, Brown MB, McAuley WJ. The use of heat and chemical penetration enhancers to increase the follicular delivery of erythromycin to the skin. European Journal of Pharmaceutical Sciences 2019; 132: 55-62. doi: 10.1016/j.ejps.2019.02.030 37. Cyphert E, Wallat J, Pokorski J, Recum H. Erythromycin modification that improves its acidic stability while optimizing it for local drug delivery. Antibiotics 2017; 6: 11. doi: 10.3390/antibiotics6020011 38. Jia Y, Bai S, Park CB, Wang Q. Effect of boric acid on the foaming properties and cell structure of poly(vinyl alcohol) foam prepared by supercritical-CO2 thermoplastic extrusion foaming. Industrial & Engineering Chemistry Research 2017; 56 (23): 6655-6663. doi: 10.1021/acs. iecr.7b01171 39. Subrahmanyam PJ. Design and development of guar gum and borax crosslinked guar gum matrix tablets of theophylline for colon specific drug. Journal of Chemical and Pharmaceutical Research 2012; 4 (2): 1052-1060. doi: 40. Martinez A, Caves J, Ravi S, Li W, Chaikof E. Effects of crosslinking on the mechanical properties drug release, and cytocompatibility of protein polymers. Acta Biomaterialia 2013; 10: doi: 10.1016/j.actbio.2013.08.029 41. Kwon JS, Kim DY, Seo HW, Jeong SH, Kim JH et al. Preparation of erythromycin-loaded poly(vinylalcohol) film and investigation of its feasibility as a transdermal delivery carrier. Tissue Engineering and Regenerative Medicine 2014; 11 (3): 211-216. doi: 10.1007/s13770-014- 0018-7 42. Shahnavi I, Ahmed S, Sheraz M, Vaid F. Borate: Toxicity, effect on drug stability and analytical applications. In: Chung MP (editor). Handbook on Borates: Chemistry, Production and Applications. Hauppauge, NY, USA: Nova Science, 2010, pp. 19-58. 43. Ahmadi Y, Siddiqui MT, Haq QMR, Ahmad S. Synthesis and characterization of surface-active antimicrobial hyperbranched polyurethane coatings based on oleo-ethers of boric acid. Arabian Journal of Chemistry 2020; 13 (1): 2689-2701. doi: 10.1016/j.arabjc.2018.07.001 44. Ahmad S, Mahfuzul Haque M, Ashraf SM, Ahmad S. Urethane modified boron filled polyesteramide: a novel anti-microbial polymer from a sustainable resource. European Polymer Journal 2004; 40 (9): 2097-2104. doi: 10.1016/j.eurpolymj.2004.05.013 45. Efhamisisi D, Thevenon M-F, Hamzeh Y, Pizzi A, Karimi A et al. Tannin-boron complex as a preservative for 3-ply beech plywoods designed for humid conditions. Holzforschung 2017; 71 (3): 249. doi: 10.1515/hf-2016-0130 46. Hernandez-Patlan D, Solis-Cruz B, Méndez-Albores A, Latorre JD, Hernandez-Velasco X et al. Comparison of PrestoBlue® and plating method to evaluate antimicrobial activity of ascorbic acid, boric acid and curcumin in an in vitro gastrointestinal model. Journal of Applied Microbiology 2018; 124 (2): 423-430. doi: 10.1111/jam.13659 1732