Week 11 Lecture 1: Biotechnology Lecture Notes PDF
Document Details
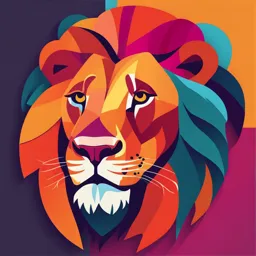
Uploaded by TopnotchOcarina
Tags
Summary
This document is a lecture transcript on biotechnology that covers the topics of DNA sequencing, PCR and cDNA libraries. It also discusses the cost and timeline of the human genome project, as well as notable scientific figures.
Full Transcript
WEEK 11 LECTURE 1: CHAPTER 20 BIOTECH PART 2 Slide 1 – What’s in your toolbox This is the second lecture for chapter 20 on biotechnology. And again I’m going to be adding to your toolbox. This is part 2. Slide 2 - Diagram So in the last lecture, we looked at DNA sequencing, PCR, and cDNA library....
WEEK 11 LECTURE 1: CHAPTER 20 BIOTECH PART 2 Slide 1 – What’s in your toolbox This is the second lecture for chapter 20 on biotechnology. And again I’m going to be adding to your toolbox. This is part 2. Slide 2 - Diagram So in the last lecture, we looked at DNA sequencing, PCR, and cDNA library. And we’re going to be moving up and add a few more skills into your toolbox. But before we do this I want to go back a little bit and talk about DNA sequencing. Slide 3 – The public effort So the sequencing of the human genome was an enormous undertaking. It was first a public effort, which means there were universities all over the world that decided to work together and it was led by Francis Collins in the United States. Was started in 1990 and it was expected to be done in 2010. But it finished seven years early. And I want to explain to you why that happened. It’s quite exciting. The cost of sequencing the first human genome was $3 BILLION, yes that's a B. The reason that the sequencing came faster is there was a private effort, which means a company, several companies but this one in particular that was also interested in sequencing a human genome. And this fellow Craig Venter was the owner of Solara genomics. You can see all the sequencing machines in Solera at the time and this caused a race between the publicly-funded universities that were working all over the world, each country would get a certain part of a chromosome and then the private effort where tons of money was poured into this company, and they were able to develop some fast techniques for sequencing. Slide 4 – Winter Holiday Books And as a result of this, the genome was sequenced, 3 er, 7 years earlier than expected which was very exciting. This was a really interesting time, and so I just wanted to highlight some books that maybe over the holidays you want something to read. These are examples of great books. So this one by Kevin Davies ‘Inside the race for the human genome sequence’ that would be a great one to look at the, basically the race between the public and the privately-funded sequencing groups. Craig Venter who was leading that company, he went on to synthesize the first synthetic organism. Which means he started from scratch, synthesized an entire piece of DNA to be an organism. And that’s in that ‘Life at the speed of light’. ‘The Common Thread’ is another great one that is the story of the politics and the ethics and the race to sequence the human genome. So super cool. And then two other ones that are awesome and there's many many more. ‘The double helix’ is an older one, talking about the discovery of DNA. It’s a must-read and then I also think a must read is the one about Rosalind Franklin The Dark Lady of DNA. Because of course as you know, we have only briefly mentioned but it was Rosalind Franklin’s discovery that allowed Watson and Crick to figure out the structure of DNA. Although they got the nobel prize for the discovery it was based on her research. So it is a great read as well. So anyways, let’s move on. Slide 5 – Sequence Cost today Oh yeah I guess the one other thing I wanted to say: compared to the three billion dollars to sequence the first human genome now you can get your DNA sequenced in one day for about $1,000. Pretty interesting. Slide 6 - Chromosome Okay so I just want to remind you of the structure of DNA a little bit. So there's your chromosome and then there's a little arm of the chromosome and you can see that it has regions that have genes that are in red or dark grey and then or dark red and then we zoom down and wait at the bottom we can see a gene where you see the exons and introns and then you could see the regulatory sequences or their promoters. So I'm just trying to remind you of the structure and where these are found in the chromosome and keeping in mind that it is the exons of that gene that eventually code for a protein. Slide 7 – Chromosome % breakdown So what's really interesting and what I eluded to in the last lecture, is a very very small proportion of the human genome, or of a genome, codes for genes. And so what this picture is trying to show, of the hundred percent of the genome, there's a whole bunch of repetitive sequences so everything on the left about a 50% are repeated sequences. These are the remnant or fossils of evolution there's things called LINEs and SINEs, these are repetitive elements. There’s old retroviruses, there’s transposons, there’s all these repeats. All the remnants of evolution of humans from many other organisms. Then the other 50% are the unique sequences but look there to see the small portion in red that is the protein coding portion. Very small compared to the introns which is about 20%. And then there’s lots of other sequences that are non-repetitive but they're not part of an intron or a codon. And so these non- repetitive sequences these would be things like promoters, enhancers, and non-coding RNAs, like ribosomal RNA, TRNAs, micro-RNAs, siRNAs and so forth. Slide 8 – Genomic DNA & cDNA And so I just wanted to emphasize based on what we were talking about in the last class about making a cDNA. The cDNA is only going to pick up that very tiny part at the end. The regions that are protein coding regions plus their 5’ and 3’ untranslated regions. But the cDNA is only represented by that tiny amount which works out to be 1-3% of the genome. The entire genome included the genes could be in a genomic library but certainly everything that's underneath genomic library there, those are the types of sequences that can only be detected in genomic DNA. So while genes could be detected in genomic DNA and cDNA all these other parts, introns, promoters, and all these other repetitive elements would only be detectable in genomic DNA. They never appear in cDNA. Ok. And that is a classic question that I could ask on a quiz or final like a, I'll give you a list of different parts of DNA and just say what library what kind of library would you need to make? A genomic DNA library or a complementary DNA library in order to detect this piece. So if I said exons for example, exons, you could make either a genomic DNA or a complementary DNA because it is present in both. Whereas things like introns are only present in the genomic library but not present in the cDNA library. Slide 9 – Diagram Cloning Okay so let's get back to our biotechnology discussion and we're going to move on to the next stage we're going to talk about plasmids we’re going to talk about restriction enzymes and we’re going to talk about cloning. Slide 10 – Basic procedures Here, sorry it’s off the end there but says gain a basic understanding of some of the common procedures in a biotechnology lab so it’s similar to what we’ve talked about before. But in this case we're going to look at how to use plasmids. We’re going to learn about restriction enzymes. We're going to learn how to clone a gene into a plasmid and then we're going to end up with a way that we can look at the expression of many genes at the same time or all genes at the same time in an organism and that’s known as Global gene expression. Slide 11 – Fig 20.5 So here is a figure that shows the steps from isolating your gene, from your organism of interest, how you get it into a plasmid, and then how you can apply that down the road. So let's break this up. Slide 12 – Fig 20.5a So the first step is, on the right you have your gene of interest and depending on whether, what part of the gene you want to look at, if you just want to look at the coding sequence then you would isolate mRNA from that cell make cDNA and then you would like to put that fully processed mRNA which is now made into double stranded DNA into a plasmid. If you wanted some other piece of the DNA maybe you would like to clone the promoter, maybe you'd like to clone an intron then you would use genomic DNA to do that. We use specific enzymes that we’ll get into in a minute, in order to cut it and then insert it into a plasmid. So as you can see on the left bacteria have chromosomal, circular chromosomal DNA but they also have this extra chromosomal DNA which means outside of the chromosome called a plasmid. Bacteria use these plasmids to exchange DNA with themselves and other bacteria all the time but we exploit these in biotechnology lab because they’re small, they’re replicated by the bacterium and we can exploit them and put our gene of interest in there and this is what cloning is. Taking your gene, putting it into another organism, in this case it’s into a plasmid that’s in a bacteria. Eventually, you put your plasmids, your gene into your plasmid, and that plasmid back into the bacteria, and then you grow that up and culture and make sure that you get lots of that plasmid grown up and then you've got lots of DNA material to work with down the way. Down the road. Slide 13 – 20.5b Then depending on what you want to do with that clone gene, you could take that gene of interest and on the left you could use transformation, plants transformation and insert it into, example a corn plant to make resist pests, or maybe it’s a gene you want to put into bacteria, so you alter the bacteria so that they can clean up toxic wastes. On the other side, you might just want to leave it in the bacteria that you start with and instead, harvest the protein and in this case if it is a human growth hormone that the bacteria is making, you harvest the protein and then you can give it to children who are unusually stunted and help them to a normal size. Or you can use a protein that dissolves blood clots to prevent heart attacks. So you clone your gene, you put it in bacteria and then what you do with it after like it shows here totally depends on whether you want to express it in an organism like in plants or bacteria as you see on the left, or do you want to just isolate the protein and then apply it to a specific process as we see on the right. Slide 14 – Fig 20.6 So how do we get a gene into a plasmid? So there's the bacterial plasmid and there’s a summary, we're going to break it down into pieces. Slide 15 – Fig 20.6a So a plasmid, again, circular DNA, double stranded of course, it’s DNA. And within that are sequences of course and what we use are specific enzymes called restriction enzymes. Restriction enzymes are isolated from bacteria and they recognize a very distinctive site like you see here GAATTC. They’re always, almost always palindromes so notice from 5’ to 3’, GAATTC on the top strand and now also on the bottom GAATTC from 5’ to 3’. So this is a sequence that a specific restriction enzyme would recognize, it would see that sequence in the plasmid in this case and it would cut it in a distinctive way where those two arrows are. The result being that plasmid will now be linearized and the ends would have what are called sticky ends. So from the 5’ end, it would have a 5’ overhang of AATT and look at on the other side 5’ to 3’, the overhang is AATT. So we’ve cut the DNA and maybe before we move on these restriction enzymes come from bacteria and it is individual bacteria’s immunity really. So these are designed to protect bacteria from incoming viruses. So these restriction sites don’t appear in the bacteria that they have been isolated. So it means that the whole genome is made without this sequence let’s say a GAATTC. And if a virus infects that bacterium it likely will have this sequence and then this restriction enzyme will cut that Viral DNA and prevent infection. And so every bacteria has these restriction enzymes and biotechnologists all over the world have identified bacteria, found the restriction enzyme, purified them, and now we have thousands of these things. So lots of different sequences and lots of different opportunities to cut DNA in different ways with different sequences. And this is really been the explosion of gene cloning because this is allowed us to cut DNA and put new DNA into specific, very specific orientations and regions. Okay so let’s look at the next stage. Slide 16 – Fig 20.6b So you have your cut plasmid in green and we're just showing the ends of it, but that’s a circle and then at the same time we have your favourite gene that we have also cut with the same enzyme so that in pink it has the same overhangs so we mix them together, we add an enzyme called DNA ligase, and it links those together so that because of the overhangs, you can see the complementary base pairing of the AATT on both sides and because of that complementary base pairing they’ll lineup and then we add DNA ligase and it seals the strands. Slide 17 – Fig 20.6c And so we have now successfully created a recombinant plasmid. Which has your favourite gene inserted into the plasmid. And so this is the basis of gene cloning. Slide 18 – Common restriction enzymes So, I'll just give you an example of some common restriction enzymes and we'll look at that how that looks. So here’s one from E. coli, Escherichia coli. This one generates a 5’ overhang and you can see that, so you can see the sequence. It's the one we've been looking at so far: GAATTC is the six nucleotide palindrome that the restriction enzyme recognizes and then the scissors there and the dotted line show you where it cuts. And then the result is that 5’ overhang from 5’ to 3’ AATT. Now if you're wondering about the naming of these restriction enzymes the first three letters represent the organism that they have been isolated from. So EcoR1 is from Escherichia coli and R1 is probably just restriction enzyme 1. A different enzyme some from Providencia stuartii is PST1. This one cuts at the sequence CTGCAG, you can see the scissors there, showing, notice that in this case it's a 3’ overhang so that if I was to read from 5’ to 3’ on that top strand the overhang is TGCA with the A being the end of the 3’ end. So we can have 5’ overhangs, 3’ overhangs, and we can have blunt ends which would be in this case cutting right in the middle and we’ll see a couple of those later. Slide 19 - Sequence So let’s see what this looks like. So here's your DNA of interest. We're going to cut it, actually in this first case, what we want to do is amplify a region of your gene so we want to amplify the region between these two arrows. You remember from my supporting lecture on PCR that the primers that you're going to use to amplify this region are exactly the sequence in those two highlighted areas. And if you don't remember this please go to the supporting lecture on PCR it’s absolutely critical for understanding this process. So those are the two primers that you would use to amplify this region and these sequences are listed as we always do, from 5’ to 3’. Slide 20 - Cloning So we're interested in cloning your gene that you're amplifying into this site. The Eco R1 PST1 site. And we would like to exchange the region that has the blue arrow with your favourite gene. And so that's how we would like to use PCR to clone your gene into this plasmid. Slide 21 – Cloning (2) So, the way that we do this, you already know how to do a standard PCR with the primers in yellow and that would have amplified the region between the two arrows but now what we do is a glorious little trick where instead of creating just a regular primer for PCR, so for example on the left, rather than just the GACTAGC as a primer, we’re going to add a restriction enzyme site to it for Eco R1. So our primer is going to be extended. It’s going to have GAATTC that's the sequence of EcoR1 restriction site and then the rest of the primer GACTACG will be the rest of the primer. So we use a much longer primer, and same on the other side we’re going to add a restriction site to the end and that’s going to be the PST1 site, so reading from 5’ to 3’ CTGCAG that’s the restriction site, it’s going to be added to the end of the primer GCTATGT. So this is a challenging concept so we’re designing primers so you really need to know PCR and now we are adding extra sequences on those fragments. Slide 22 – Cloning (3) So when we do that what is super interesting is the fragment that we generate after PCR now has as we see in these boxes, the restriction enzymes site for ecoR1 on the left and PST1 on the right. And then all we need to do is cut this DNA with these two enzymes ecoR1 and PST1. Slide 23 – Restriction Enzymes Table And just as a reminder, there are many different thousands of restriction enzymes, there’s examples here, a whole bunch of bacteria on the left, then the name of the restriction enzymes, the sites, are they a 3’ overhang, a 5’, what does it look like after they cut. I’ll just draw your attention to the one in the middle where it is a HPA1 which is a blunt cutter and it just cuts in the middle. Slide 24 - Cut And so after we've done that cutting, so there's the PCR product at the top. We do the cutting, generate that super important EcoR1 5’ overhang on the left and that PST1 3’ overhang on the right. And because we have cut the original plasmid that we’re trying to clone into with these two enzymes we can now ligate this fragment into our plasmid. Slide 25 – Restriction sites So this work is showing you how regardless of what DNA that you have, you can very easily add restriction sites on the end and clone it into any plasmid. So it’s highly powerful. Slide 26 – PCR to clone genes Let's look at this in cartoons. So this is using PCR to clone genes. So PCR primers are designed so that DNA fragments obtained by PCR have at each end a restriction site matching one in one in the cloning vector like we just did. So you can see your gene is in dark pink, the light pink are the primers with the restriction site that you want to add as part of those primers. You use the same restriction enzymes to cut your Amplified DNA that has its new restriction sites as you would a cloning vector the plasmid. You mix them together, and ligate them until you get a plasmid that's complete with your gene you cloned exactly where you want it. Then you take that plasmid, you transform it into bacteria and because these plasmids have what's called an antibiotic resistance then you can simply grow the bacteria in that antibiotic and only the bacteria as you can see in the bottom that have taken up that plasmid will survive. The two on the left will survive, the one with no plasmid will not survive. And so this is how we can clone your gene into a bacteria. Slide 27 – Fig 20.12 Alright. So as I mentioned in the last lecture we can also use PCR to follow the expression of a gene where we’re isolating mRNA making cDNA and then running it on a gel to see the quantity. This is something you can do for one gene at a time. Slide 28 – Cancer cells But there are exciting mechanisms to do this for all genes in your cells. So let’s take this specific example. You have cancer cells on the left and normal cells on the right and you want to understand under some condition maybe exposure to a drug, what genes are expressed in the cancer cells compared to the genes that are expressed in the normal cells. And this is the kind of thing that would be valuable to see if the drug that you have would work well in targeting these cancer cells. So what you do is you first isolate the mRNA from the cancer cells and separately in another tube you isolate the mRNA from the normal cells. You convert them both to cDNA but the trick is that you label one red with fluorescent dyes and you label the other green with fluorescent dyes. So the normal is green, the cancerous is red, and then what you do is you hybridized a microarray. and I'll just show you what that is in the next slide. Slide 29 – Fig 20.13 So this picture in the middle is a zooming in on what's known as a microarray. It has all these dotsso every dot corresponds to a single gene from your genome, so and that DNA is applied to a microscope something similar to a microscope slide. It’s denatured so it’s single stranded and DNA that’s in that dot is ready to bind to whatever we're going to add. We then, so keep in mind that in a picture like this but all you know 28,000 genes in your genome can all be represented in a microscope slide. That’s how small the dots are. We’re then going to incubate this microscope slide with the DNA, cDNA, that we just created in the previous slide. So remember we had cDNA from cancer cells that was labelled red, cDNA from normal cells that was labeled green. We’re going to combine those so we’ve made them separately so far, we’re going to combine them and we’re going to allow them, we’re going to apply them to this microarray and so what's going to happen is if the gene any one of these genes is expressed in the cancer cell it will bind that red RNA RNA and if it's expressed in the normal cell it will bind that green RNA. And because they’re fluorescent we actually get 4 potential colours here. So spots that are exclusively red, are genes that are only expressed in the cancerous cells. Spots that are only green are genes that are only expressed the normal cells and not the cancerous cells. So these two groups are super interesting. Genes that are only expressed in cancerous cells and genes that are only expressed in normal cells. And then there’s a large proportion of the genes that are yellow and that is because in florescence combining red and green makes a yellow and these are genes that are basically equally expressed in normal cells and cancer cells. From an experiment like these, these would be the least interesting, right? If there’s no difference between cancerous and normal cells for a gene that gene is probably not a critical one that you want to study. And then on top of that there will be spots that have no colour at all and that means that in this tissue at this time they’re, that gene is not expressed in either cancerous or normal cells. So the glorious thing about this, this is called global gene expression, you can look at every gene in the genome and ask what cells, excuse me, you can ask what genes are exclusively expressed in the cancer cells and not in normal cells. These would be amazing targets for gene therapy. For trying to understand the mechanism how cancer is taking over that cell. On the other side, what are the genes that are expressed in normal but for whatever reason have been turned off in the cancer cell. Again, super important genes to study because in there could be a hint to how cells resist the cancer and then on top of that we get to see which ones aren’t involved. So this microarray is one method to do global gene expression and another method is called RNA sequencing I won't talk about it but basically it gives the same results and that we're able to identify the genes in the cancerous versus the wild-type and, normal, and be able to continue to work on that. Slide 30 – Fig 20.13a So here's just another look so just to remind you every spot is a different gene in your genome and all the spots are telling us is that gene on cancerous tissue or the normal tissue. It's so exciting. Slide 31 - Summary And so now we've added a few more tools to your toolbox. You've learned about plasmids, restriction enzymes, cloning, and a little bit about local gene expression analysis. So I recommend that you make sure that you look at my supporting lectures for the PCR reaction and make sure that you understand the steps on how to do PCR and then also the cloning I have another one on cloning. The biotechnology that you've learned in these two lectures are going to be used throughout the rest of the course for me to ask you questions about your basic knowledge of cell biology, transcription, translation, and replication. But at the same time asking it in an applied way by using these techniques. So you'll find that all the information that you’ll, all the questions that you’ll see in the final exam, not all, but a large proportion of them will be based on these techniques but asking questions about transcription, translation and replication.