Topic 2 Chromosomal Inheritance PDF
Document Details
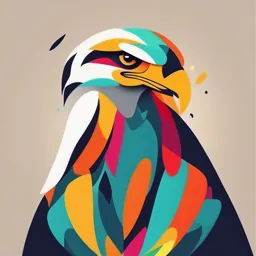
Uploaded by AppreciableDouglasFir
University of Nicosia
Tags
Related
- 1 OUTLINE Chapter 15-Chromosomal basis of inheritance.pdf
- L2 Part I - Chromosomal Basis of Inheritance 2024 Handout PDF
- ANSC20010 Genetics and Biotech Section 3: The Chromosomal Basis of Inheritance PDF
- Chromosomal Theory of Inheritance PDF
- Chapter 15: The Chromosomal Basis of Inheritance PDF
- Chromosomal Basis of Inheritance PDF
Summary
This document provides information on the chromosomal basis of inheritance, including sex-linked inheritance, the role of gene linkage in inheritance, and human disorders, and a summary of the degrees of linkage, using visuals and diagrams to illustrate the concepts.
Full Transcript
Topic 2 The Chromosomal Basis of Inheritance: Linkage and chromosomes Learning objectives (LOBs) Explain the chromosomal basis of inheritance, including sex-linked inheritance. Explain the role of gene linkage in inheritance. Describe human disorders that occur due to chromosomal alterations. Recomm...
Topic 2 The Chromosomal Basis of Inheritance: Linkage and chromosomes Learning objectives (LOBs) Explain the chromosomal basis of inheritance, including sex-linked inheritance. Explain the role of gene linkage in inheritance. Describe human disorders that occur due to chromosomal alterations. Recommended reading: Chapter 15, Campbell Biology Locating Genes Along Chromosomes Mendel did not know the existence of genes or chromosomesMendel’s “hereditary factors” were genes We now know that genes are located on chromosomes Gene locus: The location of a particular gene on a chromosome - can be seen by tagging isolated chromosomes with a fluorescent dye that highlights the gene Genes: Each gene is a long DNA molecule and consists from several nucleotides Each gene carries information for the synthesis of a specific protein (in its nucleotide sequence) – Each chromosome has > 1000 genes Fig. 15-1 Homologous chromosomes Homologous chromosomes (homologs): - The chromosome pairs during Meiosis I - They carry the same genes but they may carry different alleles of those genes In each pair, one homologous chromosome is inherited from the father (paternal homolog) and the other one from the mother (maternal homolog) => One allele is inherited from the father and the other one from the mother Homologous chromosome pairs in the human karyotype Homologous chromosomes Mendelian inheritance based on the behaviour of chromosomes (chromosomal inheritance) The chromosomal theory of inheritance states that: – Genes have specific loci (positions) on chromosomes – Chromosomes undergo segregation (separation) and independent assortment => The separation of chromosomes during meiosis accounts for Mendel’s laws of segregation and independent assortment Figure 13.7-3 Interphase Separation of homologous chromosomes in meiosis Pair of homologous chromosomes in diploid parent cell P A Duplicated pair of homologous chromosomes M Chromosomes duplicate Sister chromatids Allele separation P= paternal homolog M= maternal homolog a A, a= gene alleles A= dominant allele a = recessive allele Diploid cell with duplicated chromosomes Meiosis I A a A P Meiosis I: separates homologous chromosomes a M 1 Homologous chromosomes separate Haploid cells with duplicated chromosomes Meiosis II 2 Sister chromatids separate A P a A P M Haploid cells with unduplicated chromosomes 50% of gametes have allele A (homolog 1) a M Meiosis II: separates sister chromatids 50% of gametes have allele a (homolog 2) Fig. 15-2 P Generation Yellow-round seeds (YYRR) Y Y R Green-wrinkled seeds (yyrr) y r R y r Meiosis Fertilization y RY Gametes r All F1 plants produce yellow-round seeds (YyRr) F1 Generation R R y r Y Y LAW OF SEGREGATION The two alleles for each gene separate during gamete formation. y r LAW OF INDEPENDENT ASSORTMENT Alleles of genes on non-homologous chromosomes assort independently during gamete formation. Meiosis R r Y y r R Y y Metaphase I 1 1 R r Y y r R Y y Anaphase I R r Y y Metaphase II r R Y y 2 2 Y Y Gametes R 1/ YR 4 F2 Generation R y y r 1/ 4 Y Y r r r 1/ yr 4 Yr y R y R 1/ yR 4 An F1 F1 cross-fertilization 3 3 9 :3 :3 :1 We will follow two genes present on different chromosomes: P Generation Green-wrinkled seeds ( yyrr) Yellow-round seeds (YYRR) Y Y=yellow y= green Y r R R y y r Meiosis R=round r=wrinkled Fertilization Gametes R Y y All F1 plants produce yellow-round seeds (YyRr) r All F1 plants produce yellow-round seeds (YyRr) F1 Generation R R y r Y LAW OF SEGREGATION The two alleles for each gene separate during gamete formation. y r Y LAW OF INDEPENDENT ASSORTMENT Alleles of genes on non-homologous chromosomes assort independently during gamete formation. Meiosis r R r R Y y Metaphase I Y y 1 1 R r r R Y y Anaphase I Y y r R Metaphase II R r 2 2 Gametes R R 14 F 2 y Y Y Generation YR r r r A n F yr 1 r 14 F 1 Y Y y 14 y Y y Y Yr y y R R 14 yR cross-fertilization 3 3 9 : 3 : 3 : 1 Fig. 14-8 Y= yellow (dominant) y=green (recessive) R=round (dominant) EXPERIMENT YYRR P Generation Gametes YR Yellow Round If genes are on the same chromosome yyrr F1 Generation Predictions r= wrinkled (recessive) yr Green wrinkled YyRr Hypothesis of dependent assortment Hypothesis of independent assortment Sperm or Predicted offspring of 1/ 2 1/ 4 Sperm 1/ 1/ YR 2 yr 2 F2generation 1/ 4 YR 1/ 4 Yr 1/ 4 yR 1/ 4 yr YR YYRR YYRr YyRR YyRr YYRr YYrr YyRr Yyrr YyRR YyRr yyRR yyRr YyRr Yyrr yyRr yyrr YR YYRR Eggs 1/ 2 YyRr 1/ 4 Yr Eggs yr YyRr 3/ 4 yyrr 1/ 4 yR 1/ 4 1/ 4 Phenotypic ratio 3:1 yr 9/ 16 108 3/ 16 3/ 16 1/ 16 Phenotypic ratio 9:3:3:1 RESULTS 315 If genes are on different chromosomes 101 32 Phenotypic ratio approximately 9:3:3:1 Figure 13.10-3 Independent Assortment of Chromosomes Possibility 2 Possibility 1 P1 Y- -y 2 equally probable arrangements of chromosomes at metaphase I M1 P2 R- - r M2 Y- P1 R- P2 M1 M2 -y -r Combination 1 Combination 2 P1 and P2 YR M1 and M2 yr P1 Y- -y M1 r- -R P2 M2 Y- P1 M1 -y r- M2 P2 -R Metaphase II Daughter cells (gametes) Combination 3 Combination 4 P1 and M2 Yr M1 and P2 yR Figure 13.7-3 Interphase Separation of homologous chromosomes in meiosis Pair of homologous chromosomes in diploid parent cell Duplicated pair of homologous chromosomes P Y- M -y R- -r Chromosomes duplicate Sister chromatids Allele separation P= paternal homolog M= maternal homolog Y/y, R/r = gene alleles Y,R= dominant alleles y,r = recessive alleles Diploid cell with duplicated chromosomes Meiosis I y--y Y- -Y P R- -R 1 Homologous chromosomes separate M r- -r Haploid cells with duplicated chromosomes Meiosis I: separates homologous chromosomes Meiosis II 2 Sister chromatids separate y-Y M P P rR-R Haploid cells with unduplicated chromosomes Y- -y M -r Meiosis II: separates sister chromatids 50% of gametes have alleles YR (homolog 1) 50% of gametes have alleles yr (homolog 2) Morgan’s Experimental Evidence Thomas Hunt Morgan: Chromosomal theory of inheritance: Genes are located on chromosomes Morgan’s experiments with fruit flies provided evidence that genes (Mendel’s heritable factors) are located on chromosomes Morgan’s Experimental Evidence Several characteristics make fruit flies a convenient organism for genetic studies: – They breed at a high rate – A generation can be bred every 2 weeks – They have only 4 pairs of chromosomes Morgan observed wild type (normal) phenotypes that were common in the fly populations and alternative traits called mutant phenotypes Fig. 15-3 Wild type Red eyes Mutant White eyes Correlating Behaviour of a Gene’s Alleles with Behaviour of a Chromosome Pair Morgan mated male flies with white eyes (mutant) with female flies with red eyes (wild type) – The F1 generation all had red eyes – The F2 generation showed the 3:1 red:white eye ratio, but only males had white eyes Morgan determined that the white-eyed mutant allele must be located on the X chromosome Morgan’s finding supported the chromosomal theory of inheritance Fig. 15-4 EXPERIMENT P Generation F1 Generation All offspring had red eyes RESULTS R = red eyes allele r = white eyes allele F2 Generation CONCLUSION (EXPLANATION) w+= red eyes allele w= white eyes allele P Generation w+ X X w+ X Y F1 Generation w+ Sperm w+ w+ w+ F2 Generation w+ w w+ w+ w w+ Y XR X R X r X R Y XR X RXr XRY w Eggs X rY XRXR Xr w Eggs w Sperm XRXr XRY w+ XR w Y X R XRXR XRY Xr r XRX r X Y The Chromosomal Basis of Sex: Sex-linked genes exhibit unique patterns of inheritance In humans and some other animals, there is a chromosomal basis of sex determination In humans and other mammals, there are 2 different types of sex chromosomes: a larger X chromosome and a smaller Y chromosome The X and Y chromosomes are different (nonhomologous) Only the ends of the Y chromosome have regions that are homologous with the X chromosome (X and Y chromosome have about 18 genes in common) Females are XX and males are XY genotype Each ovum contains an X chromosome, while a sperm may contain either an X or a Y chromosome Other animals have different methods of sex determination The X and Y chromosomes X Y Sex determination Fig. 15-6a Sex determination 44 + XY 44 + XX Parents 22 + 22 + or Y X Sperm 44 + XX 22 + X + Egg or 44 + XY Zygotes (offspring) (a) The X-Y system Inheritance of Sex-Linked Genes The sex chromosomes have genes for many characters unrelated to sex Sex-linked gene: A gene located on either sex chromosome In humans, sex-linked usually refers to a gene on the X chromosome (X-linked genes), as the Y chromosome carries few genes - X chromosome has about 1100 genes, whereas Y chromosome only has 78 genes => very few Y-linked genes Inheritance of Sex-Linked Genes Sex-linked genes follow specific patterns of inheritance For a recessive sex-linked trait to be expressed – A female needs 2 copies of the allele – A male needs only 1 copy of the allele Sex-linked recessive disorders are much more common in males than in females X-linked recessive disorders examples: haemophilia, colour blindness, Duchenne muscular dystrophy Sex-linked disorders: X-linked (recessive or dominant) Y-linked The transmission of sexlinked recessive traits When a homozygous normal woman has children with an affected male: N= normal sight n= colour blindness XNXN Sperm Xn Xn Y (1) All female children will be heterozygous as they will receive a normal copy of the gene from the mother and a mutant copy of the gene from the father Y Eggs XN XNXn XNY XN XNXn XNY (2) All male children will be normal as they will receive only one normal X chromosome from the mother The transmission of sexlinked recessive traits XNXn Sperm XN XNY Y When a heterozygous woman has children with a normal male: (1) 50% of all female children will be normal and 50% will be heterozygous Eggs XN XNXN XNY Xn Xn XN XnY N= normal sight n= colour blindness (2) 50% of all male children will be normal and 50% will have color blindness The transmission of sexlinked recessive traits XNXn Sperm Xn Xn Y Y Eggs XN XNXn XNY Xn Xn Xn Xn Y N= normal sight n= colour blindness When a heterozygous woman has children with a male with colour blindness: (1) 50% of all female children will be carriers and 50% will have color blindness (2) 50% of all male children will be normal and 50% will have color blindness Y-linked genes and Y-linked genetic disorders Example of Y chromosome gene: the SRY gene on the Y chromosome codes for the development of testes - Absence of SRY gene in females leads to development of gonads into ovaries - Rare abnormalities in this gene lead to the development of XY as females and XX as males Sex-linked genes: Y-linked genetic disorders Swyer syndrome (XY gonadal dysgenesis): Mutations in SRY gene (=> inactivation) give rise to XY females with gonadal dysgenesis SRY is essential for “maleness” => inactivation of the SRY gene on the Y chromosome means XY individuals that are normally male will have female characteristics XX male syndrome: translocation of part of the Y chromosome containing the SRY gene to the X chromosome => X chromosome carries the normally male SRY gene => females have male characteristics Gender verification problem in Olympics: athletes with SRY gene were not permitted to participate as females (1992 Olympics, then ruled out) SRY gene disorders Swyer syndrome: 46XY males with female characteristics due to SRY inactivation Caster Semenya case, Olympics 2008 (not permitted to participate as female) SRY gene disorders: XX male syndrome Translocation: exchange of chromosomal fragments between non-homologous chromosomes (abnormal) Genetic recombination: exchange of chromosomal fragments between homologous chromosomes (normal) Paternal chromosomes Y chromosome Sperm chromosomes Abnormal Y chromosome Meiosis SRY gene X chromosome Abnormal X chromosome (abnormal sperm) Upon fertilisation => formation of abnormal XX zygote leading to XX male syndrome Sex-linked genetic disorder types X-linked recessive disorders X-linked dominant disorders Y- linked disorders Sex-linked genes: X-linked recessive disorders Some disorders caused by recessive alleles on the X chromosome in humans: Colour blindness - inability or decreased ability to see perceive colour differences Duchenne muscular dystrophy - Affects 1/ 3500 males in the US - Progressive weakening of the muscles and loss of coordination - Affected individuals rarely pass the age of 20 Haemophilia - A disease in which progressive bleeding is prolonged in the affected individual following an injury - Due to clotting factor VIII deficiency X Inactivation in Female Mammals Mammalian females: 1 of the 2 X chromosomes in each somatic cell is randomly inactivated during embryonic development The inactive X condenses into a Barr body which lies inside of the nuclear envelope In the ovaries, the Barr body chromosomes are reactivated in the cells that give rise to eggs, so every female gamete has an active X If a female is heterozygous for a particular gene located on the X chromosome, she will be a mosaic for that character => Mosaicism: Some somatic cells will express the phenotype of one X-linked gene and some cells of the other Fig. 15-8 X chromosomes Early embryo: Two cell populations in adult cat: Active X Allele for orange fur Allele for black fur Cell division and X chromosome inactivation Active X Inactive X Black fur Orange fur X-inactivation in human females Mosaicism in humans can be observed in an X-linked mutation that prevents the development of sweat glands (hypohidrotic ectodermal dysplasia) A woman who is heterozygous has patches of normal skin and patches of skin lacking sweat glands Mosaicism in humans: X-inactivation Hypohidrotic ectodermal dysplasia (different from vitiligo) heterochromia Gene linkage: How Linkage Affects Inheritance Gene linkage: Genes located near each other on the same chromosome that tend to be inherited together are called linked genes Morgan did other experiments with fruit flies to see how linkage affects inheritance of 2 characters Morgan crossed flies that differed in traits of body color and wing size Morgan discovered that body color and wing size are usually inherited together in specific combinations because the genes for these characters are on the same chromosome => Mendel’s law of independent assortment does not apply to linked genes Thomas Hunt Morgan experiments GGWW ggww P generation F1 generation G= gray body g=black body W= normal wings w = small wings GgWw Gray body, normal wings F1 generation: GgWw genotype Gene linkage ◼ Prediction: Law of independent assortment predicts 25% probability of appearance of each phenotype ◼ Results: However, the experimental results were different: 80% parental phenotypes 20% non-parental phenotypes ▪ 40% 40% 10% 10% Explanation: the genes G/W and g/w are linked = located near each other on the same chromosome How does linkage work? G g g W w w w g Chromosomes Gametes: GW gw If the genes were completely linked, the offspring genotypes would be: - 50% GgWw - 50% ggww gw gw Gametes gw gw GW GgWw GgWw gw ggww ggww However, there is a small percentage of non-parental genotypes (Ggww, ggWw) Genetic Recombination and Linkage Linked genes are inherited together as a package Complete linkage should have resulted in 2 phenotypes (50% grey normal wings, 50% black reduced wings) However, the result was: 80% parental phenotypes: 40% grey normal wings, 40% black reduced wings 20% non-parental phenotypes: 10% black normal wings, 10% grey reduced wings were produced due to genetic recombination (crossing over) between G/g and W/w Genetic Recombination and Linkage Morgan found that body color and wing size are usually inherited together in specific combinations (=> parental phenotypes) These genes do not assort independently because they are on the same chromosome (law of independent assortment does not apply to linked genes) However, non-parental phenotypes were also produced due to genetic recombination => the production of offspring with combinations of traits differing from either parent The genetic findings of Mendel and Morgan relate to the chromosomal basis of recombination Recombination of Linked Genes: Crossing Over Morgan discovered that genes can be linked, but the linkage (in this case) was incomplete, as evident from recombinant phenotypes Morgan proposed that genetic recombination can sometimes break the physical connection between genes on the same chromosome => unlinks (separates) genes Genetic recombination: crossing over of non-sister chromatids of homologous chromosomes during Meiosis I Animation: Crossing Over Genetic recombination non-parental chromosomes Meiosis Before Meiosis Gametes produced Chromosomes in gametes Exchange of genetic material between non –sister chromatids of homologous chromosomes Recombination frequency Recombination frequency= number of offspring with recombinant (non-parental) phenotypes Total number of offspring Fig. 15-10 Gray body, normal wings (F1 dihybrid) Testcross parents b+= gray body (G) Replication of chromob=black body (g) somes + vg = normal wings (W) vg=vestigial (small) Meiosis I wings (w) Black body, vestigial wings (double mutant) b+ vg+ b vg b vg b vg Replication of chromosomes b+ vg+ b vg b+ vg+ b vg bvg bvg bvg bvg b+ vg+ Meiosis I and II b+ vg b vg+ b vg Meiosis II Recombinant chromosomes Eggs Testcross offspring b+ vg+ b vg b+ vg b vg+ 965 944 206 185 Wild type (gray-normal) Blackvestigial Grayvestigial Blacknormal b+ vg+ b vg b+ vg b vg+ b vg b vg b vg b vg Parental-type offspring b vg Sperm Recombinant offspring Recombination = 391 recombinants 100 = 17% frequency 2300 total offspring Approx. 20% Unlinked Genes: Independent Assortment of Chromosomes Mendel observed that combinations of traits in some offspring differ from either parent (non-parental phenotypes) Offspring with a phenotype matching one of the parental phenotypes are called parental types Offspring with non-parental phenotypes (new combinations of traits) are called recombinant types (recombinants) Unlinked genes: frequency of recombination = 50% (in heterozygous x homozygous recessive crossing) => 50% new phenotypes observed for any 2 genes on different chromosomes (unlinked genes) because of the independent assortment of chromosomes Unlinked Genes: Independent Assortment of Chromosomes Gametes from yellow-round heterozygous parent (YyRr) Gametes from greenwrinkled homozygous recessive parent ( yyrr) YR yr Yr yR YyRr yyrr Yyrr yyRr yr Parentaltype offspring 50% Recombinant offspring 50% Genetic Recombination and Linkage Recombinant offspring exhibit new combinations of traits inherited from two parents (non-parental chromosomes) Genetic recombination (happens during Meiosis I) may cause linked genes to separate => Production of new non-parental genotypes as if the genes were not linked (according to independent assortment) Linked genes: Recombination frequency < 50% -crossing over between non-sister chromatids during meiosis I accounts for the observed recombinants Completely linked genes: Recombination frequency= 0% Incompletely linked genes: Recombination frequency between 0- 50 % Mapping the Distance Between Genes Using Recombination Data Morgan predicted that the further apart 2 genes are => the higher the probability that a crossover will occur between them => the higher the chance they separate (unlink) The higher the recombination frequency => the higher the chance that 2 linked genes may separate Genes that are far apart on the same chromosome can have a recombination frequency near 50% => Such genes are physically linked, but genetically unlinked => behave as if found on different chromosomes Mapping the Distance Between Genes Using Recombination Data Genetic map: an ordered list of the genetic loci along a particular chromosome Linkage map: a genetic map of a chromosome based on recombination frequencies Distances between genes can be expressed as map units 1 map unit (centimorgan) = 1% recombination frequency Cytogenetic (linkage) maps indicate the relative positions of genes with respect to chromosomal features, not precise locations of genes Fig. 15-11 Linkage map RESULTS Recombination frequencies 9% Chromosome 9.5% 17% b cn vg Fig. 15-12 A partial genetic (linkage) map of a Drosophila chromosome Mutant phenotypes Short aristae 0 Long aristae (appendages on head) Black body 48.5 Gray body Cinnabar eyes 57.5 Red eyes Vestigial wings 67.0 Normal wings Wild-type phenotypes Brown eyes 104.5 Red eyes Summary: Linkage degrees Relative position Completely linked genes very close to each other on the same chromosome Incompletely on the same (partially) linked chromosome but there genes is some distance between them Physically linked but genetically unlinked genes Recombination Frequency (RF) no genetic recombination occurs (RF=0%) genetic recombination takes place => 0< RF< 50% (depending on the distance between them) Genes located far away RF close to 50% from each other on the same chromosome => often behave as unlinked genes Unlinked genes on different chromosomes RF= 50% (no genetic => are NOT inherited recombination occurs, together => they follow due to law of Mendel’s law of independent independent assortment) assortment Note: the RF values in these lectures refer to the dihybrid crosses of heterozygote x recessive homozygote crossing (AaBb x aabb) Alterations of chromosome number or structure cause genetic disorders Large-scale chromosomal alterations often lead to spontaneous abortions (miscarriages) or cause a variety of developmental disorders Chromosomal abnormalities: - Chromosome number - Chromosome structure Abnormal Chromosome Number: Aneuploidy Aneuploidy: presence of abnormal chromosome number - results from the fertilization of gametes in which nondisjunction occurred => Offspring with this condition have an abnormal number of a particular chromosome => abnormal karyotype Nondisjunction: abnormal separation of homologous chromosome pairs during meiosis I or sister chromatids during meiosis II Nondisjunction of homologous chromosomes in meiosis I => one gamete receives both homologous chromosomes from a pair, and another gamete receives none (0 chromosomes) -Nondisjunction of sister chromatids in meiosis II: results from non-separation of sister chromatids during meiosis II => half the gametes have 1 more or 1 less chromosome at the end of meiosis II, the other half are normal A monosomic zygote has only 1 copy of a particular chromosome A trisomic zygote has 3 copies of a particular chromosome Fig. 15-13-3 Meiosis I Nondisjunction Meiosis II Nondisjunction Gametes n+1 n+1 n–1 n–1 n+1 n–1 n Number of chromosomes (a) Nondisjunction of homologous chromosomes in meiosis I (b) Nondisjunction of sister chromatids in meiosis II n Abnormal Chromosome Number Aneuploidy is a condition in which the number of chromosomes in a cell is not an exact multiple of the monoploid (haploid) number of a particular species Polyploidy is a condition in which an organism has more than 2 complete sets of chromosomes – Triploidy (3n) is three sets of chromosomes – Tetraploidy (4n) is four sets of chromosomes Polyploidy is common in plants, but not animals Polyploids are more normal in appearance than aneuploids Fig. 15-14 Abnormal Chromosome Number tetraploid mammal Alterations of Chromosome Structure Breakage of a chromosome can lead to 4 types of changes in chromosome structure: – Deletion: removal of a chromosomal segment – Duplication: repetition of a segment – Inversion: reversal of a segment within a chromosome – Translocation: exchange of segments between nonhomologous chromosomes (movement of a segment from one chromosome to another) Alterations of Chromosome Structure Fig. 15-15 (a) (b) (c) (d) A B C D E FG H A B C D E F G H A B C D E FG H A B C D E FG H Deletion Duplication A B C E FG H A B C B C D E Inversion A D C B E R FG H M N O C D E Reciprocal translocation M N O P Q F G H A B P Q R FG H Human Disorders Due to Chromosomal Alterations Alterations of chromosome number and structure are associated with some serious disorders Some types of aneuploidy appear to upset the genetic balance less than others, resulting in individuals surviving to birth and beyond These surviving individuals have a set of symptoms, or syndrome, characteristic of the type of aneuploidy Common types of human autosomal trisomies: Trisomy 21 (Down syndrome) Trisomy 18 (Edwards syndrome) Trisomy 13 (Patau syndrome) Autosomal trisomy symptoms: birth defects, intellectual disability (mental retardation) and shortened life expectancy Down Syndrome (Trisomy 21) Down syndrome is an aneuploid condition that results from 3 copies of chromosome 21 It affects about 1 out of every 700 children born The frequency of Down syndrome increases with the age of the mother, a correlation that has not been explained Fig. 15-16b Down Syndrome Karyotype Down’s syndrome Aneuploidy of Sex Chromosomes Non-disjunction of sex chromosomes produces a variety of aneuploid conditions: - XXY or ΧΥΥ males - XXX, XXXX, or XΟ females Klinefelter syndrome: XXY individuals, males with an extra X chromosome => males with some female characteristics and developmental abnormalities (e.g. gynecomastia) Turner syndrome (monosomy X) : XO females, sterile (infertile); it is the only known viable monosomy in humans Klinefelter Syndrome (XXY) Klinefelter Syndrome(XXY) Karyotype Klinefelter Syndrome(XXY) Gynecomastia Turner Syndrome (XO) Disorders Caused by Structurally Altered Chromosomes Syndrome Cri du chat (“cry of the cat”): -results from deletion of part of chromosome 5 -children born with this syndrome are mentally retarded and have a cat-like cry; usually die in infancy or early childhood Certain cancers, including chronic myelogenous leukaemia (CML), are caused by translocations of chromosomes Cri du Chat syndrome Chromosome structure abnormality: deletion of part of chromosome 5 Results in serious mental retardation Cri du Chat syndrome Fig. 15-17 Chronic myelogenous leukaemia (CML) Normal chromosome 9 Normal chromosome 22 Reciprocal translocation Translocated chromosome 9 Translocated chromosome 22 (Philadelphia chromosome) Reciprocal translocation: exchange of fragments between non–homologous chromosomes Sex chromosome structural abnormalities: XX male syndrome (SRY gene disorders) Translocation: exchange of chromosomal fragments between non-homologous chromosomes (abnormal) Genetic recombination: exchange of chromosomal fragments between homologous chromosomes (normal) Paternal chromosomes Y chromosome Sperm chromosomes Abnormal Y chromosome Meiosis SRY gene X chromosome Abnormal X chromosome (abnormal sperm) Upon fertilisation => formation of abnormal XX zygote leading to XX male syndrome Some inheritance patterns are exceptions to the standard chromosomal theory of inheritance There are 2 normal exceptions to Mendelian genetics: Inheritance of nuclear genes: genomic imprinting Inheritance of genes located outside the nucleus: extranuclear or cytoplasmic genes (e.g. organellar genes) Genomic Imprinting Genomic Imprinting: silencing (inhibition of expression, inactivation) of either the maternal or paternal alleles of certain genes at the beginning of development => variation in phenotype depending on which parent passed along the alleles for certain mammalian traits to the offspring Silencing of certain genes: involves “stamping” with an imprint (methylation) during gamete production Imprinting is the result of DNA methylation (addition of – CH3) Only affects a small fraction of mammalian genes (1 %) Most imprinted genes are critical for embryonic development Fig. 15-18 Genomic imprinting of the mouse Igf2 gene Paternal chromosome Normal Igf2 allele is expressed Maternal chromosome Normal Igf2 allele is not expressed Wild-type mouse (normal size) (a) Homozygote Mutant Igf2 allele inherited from mother Normal size mouse (wild type) Dwarf mouse (mutant) Normal Igf2 allele is expressed Imprinting (inactivation) of the mutant allele Normal allele is active normal phenotype Mutant Igf2 allele inherited from father Mutant Igf2 allele is expressed Paternal chromosome Maternal chromosome Mutant Igf2 allele is not expressed Normal Igf2 allele is not expressed (b) Heterozygotes: carry 1 mutant allele Imprinting (inactivation) of the normal allele => mutant allele is active => mutant phenotype Genomic imprinting role in humans Igf2: growth factor essential for embryonic/fetal development Also imprinted (inactivated) in humans => maternal allele normally silenced by methylation Beckwith-Wiedemann Syndrome (BWS): - Abnormal activation of maternal Igf2 allele during egg formation/early development - Symptoms: overgrowth and increased risk of childhood cancer - 1: 15000 births Inheritance of Organelle Genes Extranuclear genes (cytoplasmic genes): - genes found in organelles in the cytoplasm - Genes of mitochondria, chloroplasts, and other plant plastids: these organelles carry small circular DNA molecules (which contain genes) Extranuclear genes are inherited maternally because the zygote’s cytoplasm comes from the egg The first evidence of extranuclear genes came from studies on the inheritance of yellow or white patches on leaves of an otherwise green plant Fig. 15-19 Variegated leaves from Croton dioicus Patterned leaves due to mutations that affect expression of pigment genes in the plastids (maternally inherited) Mitochondrial disorder inheritance Inheritance of Organelle Genes Some defects in mitochondrial genes prevent cells from making enough ATP (e.g. ATP synthase disorders) and result in serious neuromuscular disorders - Examples: mitochondrial myopathy and Leber’s hereditary optic neuropathy Glossary: -Myopathy= muscle deterioration -Neuropathy= nerve deterioration Mitochondrial disorder prevention ‘Three-person IVF” procedure: IVF with healthy embryo from “2 mothers” (3 parents) i.e. 2 eggs enables women with mitochondrial DNA disorders (mtDNA) to have healthy children (abnormal mtDNA/ mitochondria) Healthy egg from 2 mothers (normal mtDNA/ mitochondria) Healthy embryo from 3 parents SUMMARY OF GENETIC DISORDERS Inheritance mode Genetic disorder Autosomal recessive Cystic fibrosis Sickle cell anaemia α/β thalassaemia Albinism Autosomal dominant Huntington’s disease Achondroplasia X-linked recessive Ηaemophilia Colour blindness Duchenne muscular dystrophy X-linked dominant Vitamin D resistant (hypophosphataemic) rickets Alport syndrome Y-linked Swyer syndrome (XY gonadal dysgenesis) Mitochondrial disorders Mitochondrial myopathy Leber’s optic neuropathy SUMMARY OF CHROMOSOMAL ABNORMALITIES Abnormality type Disorder Aetiology Autosomal aneuploidy Down syndrome Edward syndrome Patau syndrome Trisomy 21 Trisomy 18 Trisomy 13 Sex chromosome aneuploidy Klinefelter syndrome Turner syndrome XXY XO Autosomal structural abnormality Cri du chat syndrome CML Chr5 part missing Translocation between chr9 and chr22 Sex chromosome structural abnormality XX male syndrome SRY gene translocation on X chromosome Summary Sex-linked inheritance: females have 2 alleles, males have only 1 allele on the X chromosome Gene linkage: genes located near each other on the same chromosome tend to be inherited together do not follow Mendel’s law of independent assortment Linkage degrees: - Completely linked genes: very close to each other on the same chromosome => no genetic recombination occurs (RF=0%) - Incompletely (partially) linked genes: on the same chromosome but there is some distance between them => genetic recombination takes place => RF= 0 - 50% (depending on the distance between them) - Unlinked genes: on different chromosomes => are NOT inherited together => they follow Mendel’s law of independent assortment => RF= 50% (in heterozygote x recessive homozygote crossing). Genes located far away from each other on the same chromosome often behave as unlinked genes (RF close to 50%) Summary Chromosomal abnormalities: - Chromosome number (e.g. Down syndrome) - Chromosome structure (e.g. Cri du Chat syndrome) Organellar gene inheritance: - e.g. mitochondrial genes, maternally inherited Gene expression regulation: Genomic imprinting: silencing (inactivation) of either the paternal or maternal alleles of certain genes involved in development by DNA methylation X-inactivation: random silencing (inactivation) of 1 out of the 2 X chromosomes in somatic cells of mammalian females SBA example Which of the following statements about homologous chromosomes is FALSE? Α. They carry the same alleles Β. They carry the same genes C. In each pair, one is inherited from the father and the other one is inherited from the mother D. Each homolog is replicated during cell division